Abstract
Purpose
To investigate the vitreous proteomic profiles of macular hole (MH) patients compared to donor eyes and epiretinal membrane (ERM) patients.
Methods
Ten donor eyes, 25 MH patients (15 MH without posterior vitreous detachment (PVD) and 10 MH with PVD), 10 ERM patients and 4 patients with vitreomacular traction syndrome (VMTS) were enrolled in the present study. The undiluted vitreous samples were obtained from all eyes. Proteins were identified using the micro-RPLC/MS/MS technique and using mass spectrometric data analysis from the IPI database. Proteins were searched for the function, subcellular location and tissue specificity using the Uniprot database.
Results
The mean vitreous protein concentrations were the highest (p < 0.01) in MH patients without PVD. There were no qualitative changes according to the age of donor eyes. However, the authors newly-identified 12 proteins (i.e. vasorin) in donor eyes. Ten out of 136 proteins (7.3%) (i.e. retinoschisin) were exclusively detected in the MH samples. S100A8 was exclusively detected in eyes without PVD, whereas CD59 was exclusively detected in eyes with PVD.
Figures and Tables
Figure 1
Classification of patients based on the features of optical coherence tomography (OCT). VMTS = vitreomacular traction syndrome; MH = macular hole; PVD = posterior vitreous detachment; ERM = epiretinal membrane.

Figure 2
Comparison of vitreous proteomes among donor eyes, macular hole (MH) samples and epiretinal membrane (ERM) samples. (A) Average protein concentrations in vitreous. (B) Venn diagram of proteins identified from the control (donor eye), MH and ERM samples. (C) Function categorizations of vitreous proteins identified.

Figure 3
Comparison of vitreous proteomes according to the presence of posterior vitreous detachment (PVD). (A) Average protein concentrations in vitreous. (B) Venn diagram of proteins identified based on the status of PVD. (C) Function categorizations of vitreous proteins identified.
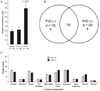
Figure 4
Representative MS/MS spectrum from four proteins that was identified specific for macular hole and epiretinal membrane.
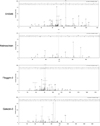
References
1. Sebag J, Balazs EA. Morphology and ultrastructure of human vitreous fibers. Invest Ophthalmol Vis Sci. 1989. 30:1867–1871.
2. Lazarus HS, Hageman GS. In situ characterization of the human hyalocyte. Arch Ophthalmol. 1994. 112:1356–1362.
3. Gärtner J. The fine structure of the vitreous base of the human eye and pathogenesis of pars planitis. Am J Ophthalmol. 1971. 71:1317–1327.
4. Bishop PN. Structural macromolecules and supramolecular organisation of the vitreous gel. Prog Retin Eye Res. 2000. 19:323–344.
5. Gaudric A, Haouchine B, Massin P, et al. Macular hole formation: new data provided by optical coherence tomography. Arch Ophthalmol. 1999. 117:744–751.
6. Hikichi T, Yoshida A, Trempe CL. Course of vitreomacular traction syndrome. Am J Ophthalmol. 1995. 119:55–61.
7. Foos RY. Ultrastructural features of posterior vitreous detachment. Albrecht Von Graefes Arch Klin Exp Ophthalmol. 1975. 196:103–111.
8. Yamane K, Minamoto A, Yamashita H, et al. Proteome analysis of human vitreous proteins. Mol Cell Proteomics. 2003. 2:1177–1187.
9. Peng J, Elias JE, Thoreen CC, et al. Evaluation of multidimensional chromatography coupled with tandem mass spectrometry (LC/LC-MS/MS) for large-scale protein analysis: the yeast proteome. J Proteome Res. 2003. 2:43–50.
10. Shen Y, Zhao R, Berger SJ, et al. High-efficiency nanoscale liquid chromatography coupled on-line with mass spectrometry using nanoelectrospray ionization for proteomics. Anal Chem. 2002. 74:4235–4249.
11. Gao BB, Chen X, Timothy N, et al. Characterization of the vitreous proteome in diabetes without diabetic retinopathy and diabetes with proliferative diabetic retinopathy. J Proteome Res. 2008. 7:2516–2525.
12. Kim T, Kim SJ, Kim K, et al. Profiling of vitreous proteomes from proliferative diabetic retinopathy and nondiabetic patients. Proteomics. 2007. 7:4203–4215.
13. Yu J, Liu F, Cui SJ, et al. Vitreous proteomic analysis of proliferative vitreoretinopathy. Proteomics. 2008. 8:3667–3678.
14. Lim SY, Raftery MJ, Goyette J, et al. Oxidative modifications of S100 proteins: functional regulation by redox. J Leukoc Biol. 2009. 86:577–587.
15. Marenholz I, Heizmann CW, Fritz G. S100 proteins in mouse and man: from evolution to function and pathology (including an update of the nomenclature). Biochem Biophys Res Commun. 2004. 322:1111–1122.
16. Ehrchen JM, Sunderkötter C, Foell D, et al. The endogenous Toll-like receptor 4 agonist S100A8/S100A9 (calprotectin) as innate amplifier of infection, autoimmunity, and cancer. J Leukoc Biol. 2009. 86:557–566.
17. Lavrovsky Y, Chatterjee B, Clark RA, Roy AK. Role of redox-regulated transcription factors in inflammation, aging and age-related diseases. Exp Gerontol. 2000. 35:521–532.
18. Qiao H, Hisatomi T, Sonoda KH, et al. The characterisation of hyalocytes: the origin, phenotype, and turnover. Br J Ophthalmol. 2005. 89:513–517.
19. Kita T, Hata Y, Arita R, et al. Role of TGF-beta in proliferative vitreoretinal diseases and ROCK as a therapeutic target. Proc Natl Acad Sci U S A. 2008. 105:17504–17509.
20. Kohno RI, Hata Y, Kawahara S, et al. Possible contribution of hyalocytes to idiopathic epiretinal membrane formation and its contraction. Br J Ophthalmol. 2009. 93:1020–1026.
21. Sommer F, Pollinger K, Brandl F, et al. Hyalocyte proliferation and ECM accumulation modulated by bFGF and TGF-beta1. Graefes Arch Clin Exp Ophthalmol. 2008. 246:1275–1284.
22. Hata Y, Nakao S, Kohno R, et al. Role of tumour necrosis factor-α (TNFα) in the functional properties of hyalocytes. Br J Ophthalmol. 2011. 95:261–265.
23. Gandorfer A, Scheler R, Haritoglou C, et al. Pathology of the macular hole rim in flat-mounted internal limiting membrane specimens. Retina. 2009. 29:1097–1105.
24. Molday RS. Focus on molecules: retinoschisin (RS1). Exp Eye Res. 2007. 84:227–228.
25. Karlstetter M, Walczak Y, Weigelt K, et al. The novel activated microglia/macrophage WAP domain protein, AMWAP, acts as a counter-regulator of proinflammatory response. J Immunol. 2010. 185:3379–3390.
26. Bonilha VL, Rayborn ME, Shadrach K, et al. Characterization of semenogelin proteins in the human retina. Exp Eye Res. 2006. 83:120–127.
27. Lazarus HS, Schoenfeld CL, Fekrat S, et al. Hyalocytes synthesize and secrete inhibitors of retinal pigment epithelial cell proliferation in vitro. Arch Ophthalmol. 1996. 114:731–736.
28. Schönfeld CL. Hyalocytes inhibit retinal pigment epithelium cell proliferation in vitro. Ger J Ophthalmol. 1996. 5:224–228.
29. Doshi BM, Hightower LE, Lee J. HSPB1, actin filament dynamics, and aging cells. Ann N Y Acad Sci. 2010. 1197:76–84.
30. Miki H, Takenawa T. Regulation of actin dynamics by WASP family proteins. J Biochem. 2003. 134:309–313.
31. Yang P, Tyrrell J, Han I, Jaffe GJ. Expression and modulation of RPE cell membrane complement regulatory proteins. Invest Ophthalmol Vis Sci. 2009. 50:3473–3481.
32. Vogt SD, Barnum SR, Curcio CA, Read RW. Distribution of complement anaphylatoxin receptors and membrane-bound regulators in normal human retina. Exp Eye Res. 2006. 83:834–840.
33. Liversidge J, Dawson R, Hoey S, et al. CD59 and CD48 expressed by rat retinal pigment epithelial cells are major ligands for the CD2-mediated alternative pathway of T cell activation. J Immunol. 1996. 156:3696–3703.
34. Fatma N, Kubo E, Sen M, et al. Peroxiredoxin 6 delivery attenuates TNF-alpha-and glutamate-induced retinal ganglion cell death by limiting ROS levels and maintaining Ca2+ homeostasis. Brain Res. 2008. 1233:63–78.
35. Henderson NC, Sethi T. The regulation of inflammation by galectin-3. Immunol Rev. 2009. 230:160–171.
36. Scott IC, Blitz IL, Pappano WN, et al. Mammalian BMP-1/Tolloid-related metalloproteinases, including novel family member mammalian Tolloid-like 2, have differential enzymatic activities and distributions of expression relevant to patterning and skeletogenesis. Dev Biol. 1999. 213:283–300.
37. Saika S, Yamanaka O, Sumioka T, et al. Fibrotic disorders in the eye: targets of gene therapy. Prog Retin Eye Res. 2008. 27:177–196.
38. Wang KC, Koprivica V, Kim JA, et al. Oligodendrocyte-myelin glycoprotein is a Nogo receptor ligand that inhibits neurite outgrowth. Nature. 2002. 417:941–944.
39. Shukunami C, Oshima Y, Hiraki Y. Chondromodulin-I and tenomodulin: a new class of tissue-specific angiogenesis inhibitors found in hypovascular connective tissues. Biochem Biophys Res Commun. 2005. 333:299–307.
40. Ikeda Y, Imai Y, Kumagai H, et al. Vasorin, a transforming growth factor beta-binding protein expressed in vascular smooth muscle cells, modulates the arterial response to injury in vivo. Proc Natl Acad Sci U S A. 2004. 101:10732–10737.