Abstract
Inflammatory bowel disease (IBD), the most important entities being ulcerative colitis and Crohn's disease, are chronic, relapsing and remitting inflammatory conditions that result from chronic dysregulation of the mucosal immune system in the intestinal tract. Although the precise pathogenesis of IBD is still incompletely understood, increased levels of proinflammatory cytokines, including interleukin (IL)-1β, IL-18 and tumor necrosis factor-α, are detected in active IBD and correlate with the severity of inflammation, indicating that these cytokines may play a key role in the development of IBD. Recently, the intracellular nucleo-tide-binding oligomerization domain-like receptor (NLR) family members, including NLRP1, NLRP3, NLRC4 and NLRP6, are emerging as important regulators of intestinal homeostasis. Together, one of those aforementioned molecules or the DNA sensor absent in melanoma 2 (AIM2), apoptosis-associated speck-like protein containing ‘a caspase recruitment domain (CARD)'(ASC) and caspase-1 form a large (>700 kDa) multiprotein complex called the inflammasome. Stimulation with specific microbial and endogenous molecules triggers inflammasome assembly and caspase-1 activation. Activated caspase-1 leads to the secretion of proinflammatory cytokines, including IL-1β and IL-18, and the promotion of pyroptosis, a form of phagocyte cell death induced by bacterial pathogens, in an inflamed tissue. Therefore, inflammasomes are assumed to mediate host defense against microbial pathogens and gut homeostasis, so that their dysregulation might contribute to IBD pathogenesis. This review focuses on recent advances of the role of NLRP3 inflammasome signaling in IBD pathogenesis. Improving knowledge of the inflammasome could provide insights into potential therapeutic targets for patients with IBD.
References
1. Cario E. Toll-like receptors in inflammatory bowel diseases: a decade later. Inflamm Bowel Dis. 2010; 16:1583–1597.


2. Wells JM, Rossi O, Meijerink M, van Baarlen P. Epithelial crosstalk at the microbiota-mucosal interface. Proc Natl Acad Sci U S A. 2011; 108(Suppl 1):4607–4614.


3. Koh YS. Nucleic acid recognition and signaling by Toll-like receptor 9: compartment-dependent regulation. J Bacteriol Virol. 2011; 41:131–132.


4. Chen GY, Nuñez G. Sterile inflammation: sensing and reacting to damage. Nat Rev Immunol. 2010; 10:826–837.


5. Abreu MT. Toll-like receptor signalling in the intestinal epithelium: how bacterial recognition shapes intestinal function. Nat Rev Immunol. 2010; 10:131–144.


6. Rakoff-Nahoum S, Paglino J, Eslami-Varzaneh F, Edberg S, Medzhitov R. Recognition of commensal microflora by toll-like receptors is required for intestinal homeostasis. Cell. 2004; 118:229–241.


7. Bouskra D, Brézillon C, Bérard M, et al. Lymphoid tissue genesis induced by commensals through NOD1 regulates intestinal homeostasis. Nature. 2008; 456:507–510.


8. Saleh M, Trinchieri G. Innate immune mechanisms of colitis and colitis-associated colorectal cancer. Nat Rev Immunol. 2011; 11:9–20.


9. Kang JS. Journal walk regarding the expanding role of microbiota in our gut. J Bacteriol Virol. 2011; 41:63–64.


10. Kaser A, Zeissig S, Blumberg RS. Inflammatory bowel disease. Annu Rev Immunol. 2010; 28:573–621.


11. Sartor RB. Microbial influences in inflammatory bowel diseases. Gastroenterology. 2008; 134:577–594.


12. Kim JM. Inflammatory bowel diseases and enteric microbiota. Korean J Gastroenterol. 2010; 55:4–18.


13. Abraham C, Medzhitov R. Interactions between the host innate immune system and microbes in inflammatory bowel disease. Gastroenterology. 2011; 140:1729–1737.


14. Manichanh C, Rigottier-Gois L, Bonnaud E, et al. Reduced diversity of faecal microbiota in Crohn's disease revealed by a metagenomic approach. Gut. 2006; 55:205–221.


15. Kleessen B, Kroesen AJ, Buhr HJ, Blaut M. Mucosal and invading bacteria in patients with inflammatory bowel disease compared with controls. Scand J Gastroenterol. 2002; 37:1034–1041.


16. Goyette P, Labbé C, Trinh TT, Xavier RJ, Rioux JD. Molecular pathogenesis of inflammatory bowel disease: genotypes, phenotypes and personalized medicine. Ann Med. 2007; 39:177–199.


17. Kanneganti TD, Lamkanfi M, Núñez G. Intracellular NOD-like receptors in host defense and disease. Immunity. 2007; 27:549–559.


18. Werts C, Rubino S, Ling A, Girardin SE, Philpott DJ. Nod-like receptors in intestinal homeostasis, inflammation, and cancer. J Leukoc Biol. 2011; 90:471–482.


19. Martinon F, Burns K, Tschopp J. The inflammasome: a molecular platform triggering activation of inflammatory caspases and processing of proIL-beta. Mol Cell. 2002; 10:417–426.
20. Hong S, Park S, Yu JW. Pyrin domain (PYD)-containing inflammasome in innate immunity. J Bacteriol Virol. 2011; 41:133–146.


21. Hornung V, Ablasser A, Charrel-Dennis M, et al. AIM2 recognizes cytosolic dsDNA and forms a caspase-1-activating inflammasome with ASC. Nature. 2009; 458:514–518.


22. Villani AC, Lemire M, Fortin G, et al. Common variants in the NLRP3 region contribute to Crohn's disease susceptibility. Nat Genet. 2009; 41:71–76.


23. Kanneganti TD, Ozören N, Body-Malapel M, et al. Bacterial RNA and small antiviral compounds activate caspase-1 through cryopyrin/Nalp3. Nature. 2006; 440:233–236.


24. Mariathasan S, Weiss DS, Newton K, et al. Cryopyrin activates the inflammasome in response to toxins and ATP. Nature. 2006; 440:228–232.


25. Sutterwala FS, Ogura Y, Szczepanik M, et al. Critical role for NALP3/CIAS1/Cryopyrin in innate and adaptive immunity through its regulation of caspase-1. Immunity. 2006; 24:317–327.


26. Dinarello CA. Immunological and inflammatory functions of the interleukin-1 family. Annu Rev Immunol. 2009; 27:519–550.


27. Bauer C, Duewell P, Mayer C, et al. Colitis induced in mice with dextran sulfate sodium (DSS) is mediated by the NLRP3 inflammasome. Gut. 2010; 59:1192–1199.


28. Elinav E, Strowig T, Kau AL, et al. NLRP6 inflammasome regulates colonic microbial ecology and risk for colitis. Cell. 2011; 145:745–757.


29. Hugot JP, Chamaillard M, Zouali H, et al. Association of NOD2 leucine-rich repeat variants with susceptibility to Crohn's disease. Nature. 2001; 411:599–603.


30. McGovern DP, Hysi P, Ahmad T, et al. Association between a complex insertion/deletion polymorphism in NOD1 (CARD4) and susceptibility to inflammatory bowel disease. Hum Mol Genet. 2005; 14:1245–1250.


31. Ng SC, Tsoi KK, Kamm MA, et al. Genetics of inflammatory bowel disease in Asia: Systematic review and metaanalysis. Inflamm Bowel Dis. 2011.


32. Rioux JD, Abbas AK. Paths to understanding the genetic basis of autoimmune disease. Nature. 2005; 435:584–589.


33. Travassos LH, Carneiro LA, Ramjeet M, et al. Nod1 and Nod2 direct autophagy by recruiting ATG16L1 to the plasma membrane at the site of bacterial entry. Nat Immunol. 2010; 11:55–62.


34. Maloy KJ, Powrie F. Intestinal homeostasis and its breakdown in inflammatory bowel disease. Nature. 2011; 474:298–306.


35. Henderson P, van Limbergen JE, Wilson DC, Satsangi J, Russell RK. Genetics of childhood-onset inflammatory bowel disease. Inflamm Bowel Dis. 2011; 17:346–361.


36. Hampe J, Franke A, Rosenstiel P, et al. A genome-wide association scan of nonsynonymous SNPs identifies a susceptibility variant for Crohn disease in ATG16L1. Nat Genet. 2007; 39:207–211.
37. Lees CW, Barrett JC, Parkes M, Satsangi J. New IBD genetics: common pathways with other diseases. Gut. 2011; 60:1739–1753.


38. Stappenbeck TS, Rioux JD, Mizoguchi A, et al. Crohn disease: a current perspective on genetics, autophagy and immunity. Autophagy. 2011; 7:355–374.


39. Kummer JA, Broekhuizen R, Everett H, et al. Inflammasome components NALP 1 and 3 show distinct but separate expression profiles in human tissues suggesting a site-specific role in the inflammatory response. J Histochem Cytochem. 2007; 55:443–452.


40. Leemans JC, Cassel SL, Sutterwala FS. Sensing damage by the NLRP3 inflammasome. Immunol Rev. 2011; 243:152–162.


42. Chen GY, Núñez G. Inflammasomes in intestinal inflammation and cancer. Gastroenterology. 2011; 141:1986–1919.


43. Fernandes-Alnemri T, Wu J, Yu JW, et al. The pyroptosome: a supramolecular assembly of ASC dimers mediating inflammatory cell death via caspase-1 activation. Cell Death Differ. 2007; 14:1590–1604.


44. Fink SL, Cookson BT. Pyroptosis and host cell death responses during Salmonella infection. Cell Microbiol. 2007; 9:2562–2570.


45. Mayer-Barber KD, Barber DL, Shenderov K, et al. Caspase-1 independent IL-1beta production is critical for host resistance to mycobacterium tuberculosis and does not require TLR signaling in vivo. J Immunol. 2010; 184:3326–3330.
46. van de Veerdonk FL, Netea MG, Dinarello CA, Joosten LA. Inflammasome activation and IL-1β and IL-18 processing during infection. Trends Immunol. 2011; 32:110–116.


47. Pizarro TT, Arseneau KO, Bamias G, Cominelli F. Mouse models for the study of Crohn's disease. Trends Mol Med. 2003; 9:218–222.


48. Kim JM, Kang HW, Cha MY, et al. Novel guggulsterone derivative GG-52 inhibits NF-kappaB signaling in intestinal epithelial cells and attenuates acute murine colitis. Lab Invest. 2010; 90:1004–1015.
49. Axelsson LG, Landström E, Goldschmidt TJ, Grönberg A, Bylund-Fellenius AC. Dextran sulfate sodium (DSS) induced experimental colitis in immunodeficient mice: effects in CD4(+)-cell depleted, athymic and NK-cell depleted SCID mice. Inflamm Res. 1996; 45:181–191.


50. Chen Y, Chou K, Fuchs E, Havran WL, Boismenu R. Protection of the intestinal mucosa by intraepithelial gamma delta T cells. Proc Natl Acad Sci U S A. 2002; 99:14338–14343.
51. Shintani N, Nakajima T, Okamoto T, Kondo T, Nakamura N, Mayumi T. Involvement of CD4+ T cells in the development of dextran sulfate sodium-induced experimental colitis and suppressive effect of IgG on their action. Gen Pharmacol. 1998; 31:477–481.


52. Hanai H, Takeda Y, Eberhardson M, et al. The mode of actions of the Adacolumn therapeutic leucocytapheresis in patients with inflammatory bowel disease: a concise review. Clin Exp Immunol. 2011; 163:50–58.


53. Tilg H, Moschen AR, Kaser A, Pines A, Dotan I. Gut, inflammation and osteoporosis: basic and clinical concepts. Gut. 2008; 57:684–694.


54. Dijkstra G, Moshage H, Jansen PL. Blockade of NF-kappaB activation and donation of nitric oxide: new treatment options in inflammatory bowel disease? Scand J Gastroenterol Suppl. 2002; (236):37–41.
55. Siegmund B. Interleukin-1beta converting enzyme (caspase1) in intestinal inflammation. Biochem Pharmacol. 2002; 64:1–8.
56. Lebeis SL, Powell KR, Merlin D, Sherman MA, Kalman D. Interleukin-1 receptor signaling protects mice from lethal intestinal damage caused by the attaching and effacing pathogen Citrobacter rodentium. Infect Immun. 2009; 77:604–614.
57. Leach ST, Messina I, Lemberg DA, Novick D, Rubenstein M, Day AS. Local and systemic interleukin-18 and inter-leukin-18-binding protein in children with inflammatory bowel disease. Inflamm Bowel Dis. 2008; 14:68–74.


58. Pagès F, Lazar V, Berger A, et al. Analysis of interleukin-18, interleukin-1 converting enzyme (ICE) and interleukin-18-related cytokines in Crohn's disease lesions. Eur Cytokine Netw. 2001; 12:97–104.
59. Pizarro TT, Michie MH, Bentz M, et al. IL-18, a novel immunor-egulatory cytokine, is upregulated in Crohn's disease: expression and localization in intestinal mucosal cells. J Immunol. 1999; 162:6829–6835.


60. Kanai T, Watanabe M, Okazawa A, et al. Macrophage-derived IL-18-mediated intestinal inflammation in the murine model of Crohn's disease. Gastroenterology. 2001; 121:875–888.


61. Nakamura S, Otani T, Ijiri Y, Motoda R, Kurimoto M, Orita K. IFN-gamma-dependent and -independent mechanisms in adverse effects caused by concomitant administration of IL-18 and IL-12. J Immunol. 2000; 164:3330–3336.
62. Ten Hove T, Corbaz A, Amitai H, et al. Blockade of endogenous IL-18 ameliorates TNBS-induced colitis by decreasing local TNF-alpha production in mice. Gastroenterology. 2001; 121:1372–1379.
63. Wirtz S, Becker C, Blumberg R, Galle PR, Neurath MF. Treatment of T cell-dependent experimental colitis in SCID mice by local administration of an adenovirus expressing IL-18 antisense mRNA. J Immunol. 2002; 168:411–420.


64. Bauer C, Loher F, Dauer M, et al. The ICE inhibitor pralnacasan prevents DSS-induced colitis in C57BL/6 mice and suppresses IP-10 mRNA but not TNF-alpha mRNA expression. Dig Dis Sci. 2007; 52:1642–1652.
65. Siegmund B, Fantuzzi G, Rieder F, et al. Neutralization of inter-leukin-18 reduces severity in murine colitis and intestinal IFN-gamma and TNF-alpha production. Am J Physiol Regul Integr Comp Physiol. 2001; 281:R1264–R1273.
66. Sivakumar PV, Westrich GM, Kanaly S, et al. Interleukin 18 is a primary mediator of the inflammation associated with dextran sulphate sodium induced colitis: blocking interleukin 18 attenuates intestinal damage. Gut. 2002; 50:812–820.


67. Li J, Moran T, Swanson E, et al. Regulation of IL-8 and IL-1beta expression in Crohn's disease associated NOD2/CARD15 mutations. Hum Mol Genet. 2004; 13:1715–1725.
68. van Beelen AJ, Zelinkova Z, Taanman-Kueter EW, et al. Stimulation of the intracellular bacterial sensor NOD2 programs dendritic cells to promote interleukin-17 production in human memory T cells. Immunity. 2007; 27:660–669.


69. van Heel DA, Ghosh S, Butler M, et al. Muramyl dipeptide and toll-like receptor sensitivity in NOD2-associated Crohn's disease. Lancet. 2005; 365:1794–1796.


70. Tamura K, Fukuda Y, Sashio H, et al. IL18 polymorphism is associated with an increased risk of Crohn's disease. J Gastroenterol. 2002; 37(Suppl 14):111–116.


71. Zhernakova A, Festen EM, Franke L, et al. Genetic analysis of innate immunity in Crohn's disease and ulcerative colitis identifies two susceptibility loci harboring CARD9 and IL18RAP. Am J Hum Genet. 2008; 82:1202–1210.


72. Lewis GJ, Massey DC, Zhang H, et al. Genetic association between NLRP3 variants and Crohn's disease does not repli-cate in a large UK panel. Inflamm Bowel Dis. 2011; 17:1387–1391.


73. Kämpfer H, Paulukat J, Mühl H, Wetzler C, Pfeilschifter J, Frank S. Lack of interferon-gamma production despite the presence of interleukin-18 during cutaneous wound healing. Mol Med. 2000; 6:1016–1027.
74. Zaki MH, Boyd KL, Vogel P, Kastan MB, Lamkanfi M, Kanneganti TD. The NLRP3 inflammasome protects against loss of epithelial integrity and mortality during experimental colitis. Immunity. 2010; 32:379–391.


75. Dupaul-Chicoine J, Yeretssian G, Doiron K, et al. Control of intestinal homeostasis, colitis, and colitis-associated colorectal cancer by the inflammatory caspases. Immunity. 2010; 32:367–378.


76. Radtke F, Clevers H. Self-renewal and cancer of the gut: two sides of a coin. Science. 2005; 307:1904–1909.


77. Allen IC, TeKippe EM, Woodford RM, et al. The NLRP3 inflammasome functions as a negative regulator of tumorigenesis during colitis-associated cancer. J Exp Med. 2010; 207:1045–1056.


78. Reuter BK, Pizarro TT. Commentary: the role of the IL-18 system and other members of the IL-1R/TLR superfamily in innate mucosal immunity and the pathogenesis of inflammatory bowel disease: friend or foe? Eur J Immunol. 2004; 34:2347–2355.


79. Takagi H, Kanai T, Okazawa A, et al. Contrasting action of IL-12 and IL-18 in the development of dextran sodium sulphate colitis in mice. Scand J Gastroenterol. 2003; 38:837–844.
80. Hirota SA, Ng J, Lueng A, et al. NLRP3 inflammasome plays a key role in the regulation of intestinal homeostasis. Inflamm Bowel Dis. 2011; 17:1359–1372.


81. Nuding S, Zabel LT, Enders C, et al. Antibacterial activity of human defensins on anaerobic intestinal bacterial species: a major role of HBD-3. Microbes Infect. 2009; 11:384–393.


82. Yoon YM, Lee JY, Yoo D, et al. Bacteroides fragilis enterotoxin induces human beta-defensin-2 expression in intestinal epithelial cells via a mitogen-activated protein kinase/I kappaB kinase/NF-kappaB-dependent pathway. Infect Immun. 2010; 78:2024–2033.
83. De Smet K, Contreras R. Human antimicrobial peptides: defensins, cathelicidins and histatins. Biotechnol Lett. 2005; 27:1337–1317.


84. Simms LA, Doecke JD, Walsh MD, et al. Reduced alpha-de-fensin expression is associated with inflammation and not NOD2 mutation status in ileal Crohn's disease. Gut. 2008; 57:903–910.
85. Wehkamp J, Harder J, Weichenthal M, et al. NOD2 (CARD15) mutations in Crohn's disease are associated with diminished mucosal alpha-defensin expression. Gut. 2004; 53:1658–1664.
86. Araki A, Kanai T, Ishikura T, et al. MyD88-deficient mice develop severe intestinal inflammation in dextran sodium sulfate colitis. J Gastroenterol. 2005; 40:16–23.


87. Fukata M, Michelsen KS, Eri R, et al. Toll-like receptor-4 is required for intestinal response to epithelial injury and limiting bacterial translocation in a murine model of acute colitis. Am J Physiol Gastrointest Liver Physiol. 2005; 288:G1055–G1065.


88. Loher F, Bauer C, Landauer N, et al. The interleukin-1 be-ta-converting enzyme inhibitor pralnacasan reduces dextran sulfate sodium-induced murine colitis and T helper 1 T-cell activation. J Pharmacol Exp Ther. 2004; 308:583–590.
89. Siegmund B, Lehr HA, Fantuzzi G, Dinarello CA. IL-1 beta-converting enzyme (caspase-1) in intestinal inflammation. Proc Natl Acad Sci U S A. 2001; 98:13249–13254.
91. Itzkowitz SH, Yio X. Inflammation and cancer IV. Colorectal cancer in inflammatory bowel disease: the role of inflammation. Am J Physiol Gastrointest Liver Physiol. 2004; 287:G7–17.
92. Suzuki R, Kohno H, Sugie S, Tanaka T. Sequential observations on the occurrence of preneoplastic and neoplastic lesions in mouse colon treated with azoxymethane and dextran sodium sulfate. Cancer Sci. 2004; 95:721–727.


93. Tanaka T, Kohno H, Suzuki R, Yamada Y, Sugie S, Mori H. A novel inflammation-related mouse colon carcinogenesis model induced by azoxymethane and dextran sodium sulfate. Cancer Sci. 2003; 94:965–973.


94. Chen GY, Shaw MH, Redondo G, Núñez G. The innate immune receptor Nod1 protects the intestine from inflammation-induced tumorigenesis. Cancer Res. 2008; 68:10060–10067.


95. Micallef MJ, Tanimoto T, Kohno K, Ikeda M, Kurimoto M. Interleukin 18 induces the sequential activation of natural killer cells and cytotoxic T lymphocytes to protect syngeneic mice from transplantation with Meth A sarcoma. Cancer Res. 1997; 57:4557–4563.
96. Zaki MH, Vogel P, Body-Malapel M, Lamkanfi M, Kanneganti TD. IL-18 production downstream of the Nlrp3 inflammasome confers protection against colorectal tumor formation. J Immunol. 2010; 185:4912–4920.


97. Osaki T, Péron JM, Cai Q, et al. IFN-gamma-inducing factor/IL-18 administration mediates IFN-gamma- and IL-12-independent antitumor effects. J Immunol. 1998; 160:1742–1749.
98. Osaki T, Hashimoto W, Gambotto A, et al. Potent antitumor effects mediated by local expression of the mature form of the interferon-gamma inducing factor, interleukin-18 (IL-18). Gene Ther. 1999; 6:808–815.
99. Cao E, Zang X, Ramagopal UA, et al. T cell immunoglobulin mucin-3 crystal structure reveals a galectin-9-independent li-gand-binding surface. Immunity. 2007; 26:311–321.


100. Coughlin CM, Salhany KE, Wysocka M, et al. Interleukin-12 and interleukin-18 synergistically induce murine tumor regression which involves inhibition of angiogenesis. J Clin Invest. 1998; 101:1441–1452.


101. Hegardt P, Widegren B, Li L, et al. Nitric oxide synthase inhibitor and IL-18 enhance the anti-tumor immune response of rats carrying an intrahepatic colon carcinoma. Cancer Immunol Immunother. 2001; 50:491–501.


102. Salcedo R, Worschech A, Cardone M, et al. MyD88-mediated signaling prevents development of adenocarcinomas of the colon: role of interleukin 18. J Exp Med. 2010; 207:1625–1636.


103. Hu B, Elinav E, Huber S, et al. Inflammation-induced tumorigenesis in the colon is regulated by caspase-1 and NLRC4. Proc Natl Acad Sci U S A. 2010; 107:21635–21640.


104. Zaki MH, Lamkanfi M, Kanneganti TD. The Nlrp3 inflammasome: contributions to intestinal homeostasis. Trends Immunol. 2011; 32:171–179.


105. Srivastava S, Salim N, Robertson MJ. Interleukin-18: biology and role in the immunotherapy of cancer. Curr Med Chem. 2010; 17:3353–3357.


106. Hu X, Chakravarty SD, Ivashkiv LB. Regulation of interferon and Toll-like receptor signaling during macrophage activation by opposing feedforward and feedback inhibition mechanisms. Immunol Rev. 2008; 226:41–56.


107. Wilke CM, Wei S, Wang L, Kryczek I, Kao J, Zou W. Dual biological effects of the cytokines interleukin-10 and interferon-γ. Cancer Immunol Immunother. 2011; 60:1529–1541.


108. Nava P, Koch S, Laukoetter MG, et al. Interferon-gamma regulates intestinal epithelial homeostasis through converging beta-catenin signaling pathways. Immunity. 2010; 32:392–402.
109. Günzel D, Florian P, Richter JF, et al. Restitution of single-cell defects in the mouse colon epithelium differs from that of cultured cells. Am J Physiol Regul Integr Comp Physiol. 2006; 290:R1496–R1507.


110. Tong Q, Vassilieva EV, Ivanov AI, et al. Interferon-gamma inhibits T84 epithelial cell migration by redirecting transcytosis of beta1 integrin from the migrating leading edge. J Immunol. 2005; 175:4030–4038.
111. Utech M, Ivanov AI, Samarin SN, et al. Mechanism of IFN-gamma-induced endocytosis of tight junction proteins: myosin II-dependent vacuolarization of the apical plasma membrane. Mol Biol Cell. 2005; 16:5040–5052.
112. Wang F, Graham WV, Wang Y, Witkowski ED, Schwarz BT, Turner JR. Interferon-gamma and tumor necrosis factoralpha synergize to induce intestinal epithelial barrier dysfunction by up-regulating myosin light chain kinase expression. Am J Pathol. 2005; 166:409–419.
Fig. 1.
Inflammasome signaling pathways. Nucleotide-binding oligomerization domain-like receptor (NLRP) within the cytosol of intestinal epithelial cells recognizes pathogen-associated molecular patterns (PAMPs) of the enteric microbiota and forms a multiprotein complex with apoptosis-associated speck-like protein containing ‘a caspase recruitment domain (CARD)' and caspase-1 called the ‘inflammasome.' NLRP3 is capable of sensing both PAMPs and endogenous damage-associated molecular patterns (DAMPs). The inflammasome plays a central role in the inflammatory process by activating caspase-1 and mediating production of proinflammatory cytokine interleukin (IL)-1β and IL-18. In addition, caspase-1 activation mediates pyroptosis, a specific form of early cell death induced by intracellular pathogens that promotes cellular lysis and the release of intracellular inflammatory contents to stimulate additional inflammatory signaling pathways.
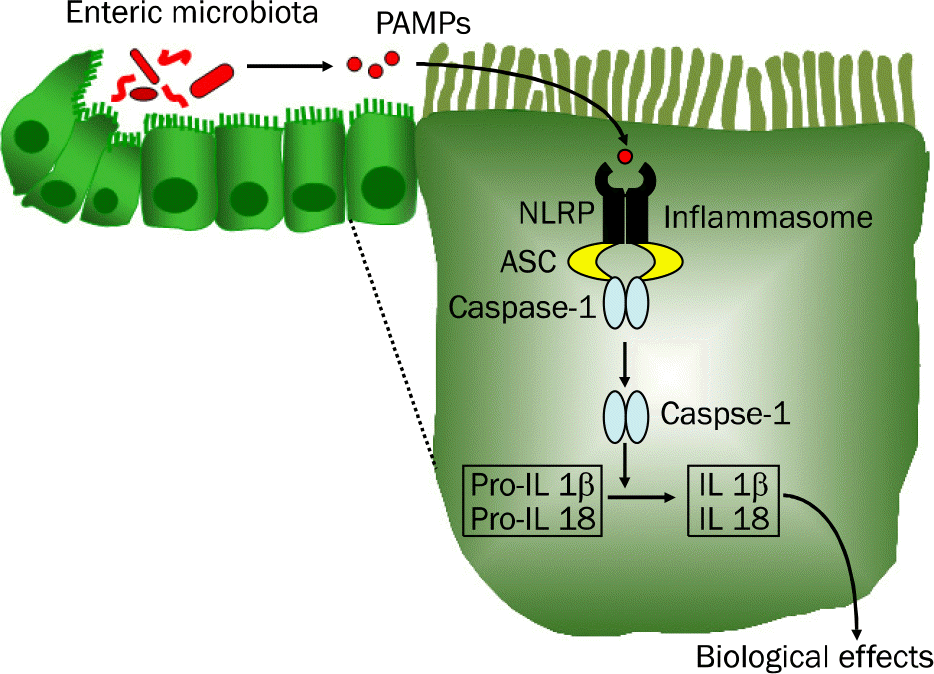
Fig. 2.
Hypothesis of nucleotide-binding oligomerization domain-like receptor (NLRP) 3 inflammasome-mediated protection against dextran sodium sulfate (DSS)-induced colitis and colitis-associated colon cancer (CAC) in mice model. DSS causes direct intestinal epithelial cell (IEC) injury, increasing permeability and translocation of bacteria into the mucosa, leading to inflammatory responses that include the recruitment of immune cells such as neutrophils and macrophages. In the stage of acute colitis, the production of interleukin (IL)-18 by the NLRP3 inflammasome may be involved in IEC repair. Chronic inflammation can lead to carcinogenesis via production of DNA-damaging oxygen radical species, proinflammatory mediators that promote cellular survival, and increases of epithelial proliferation and angiogenesis. In a murine model, the carcinogen azoxymethane is used to introduce genomic mutations by methylation. Although the mechanism still remains unclear, the downstream signaling of IL-18 and interferon-γ may play a role in inhibiting epithelial proliferation.
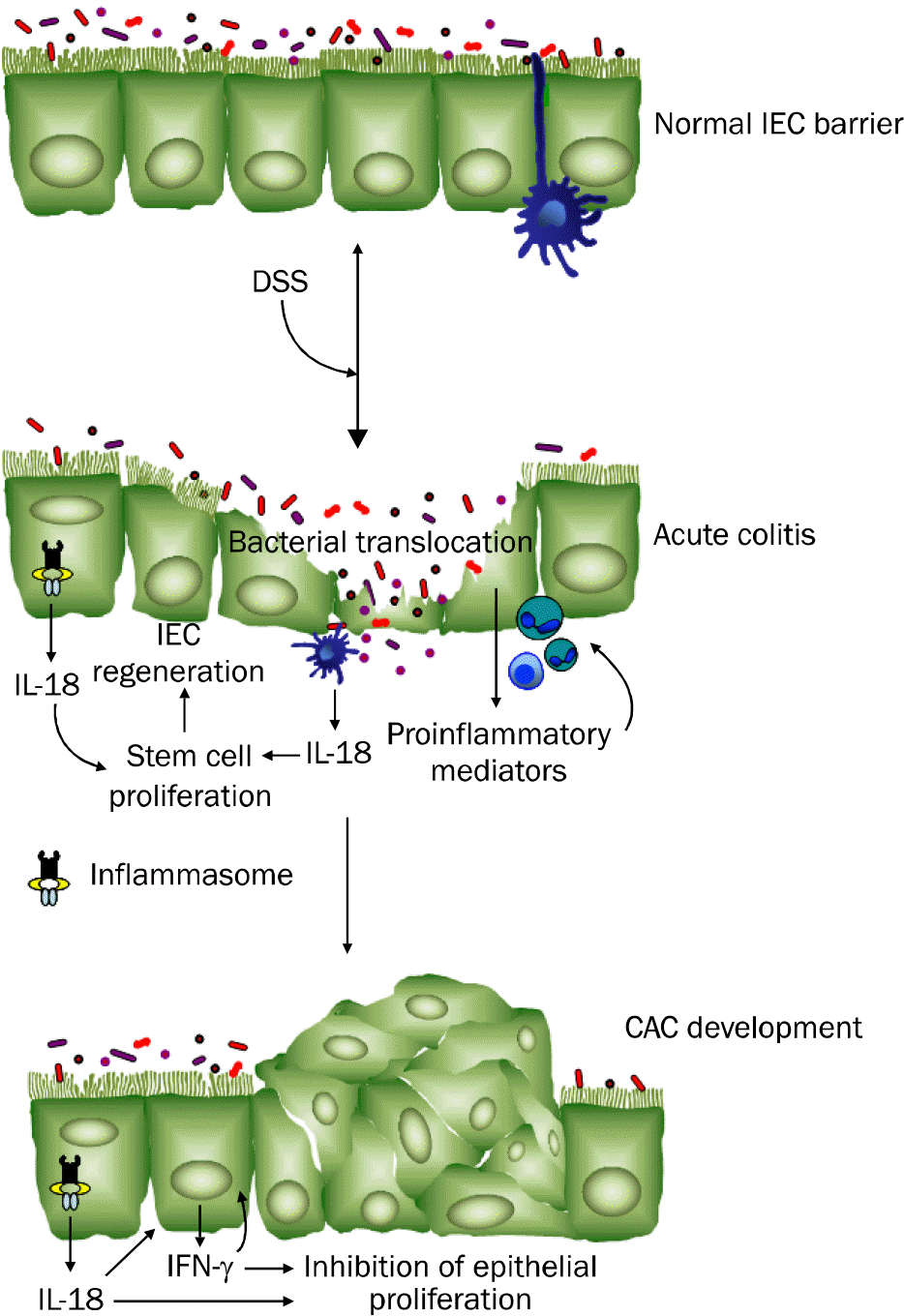