Abstract
Purpose
Existing data supports a relationship between pelvic floor dysfunction and lower urinary tract symptoms. We developed a survival model of pelvic floor dysfunction in the rabbit and evaluated cystometric (CMG), electromyographic (EMG) and ambulatory voiding behavior.
Materials and Methods
Twelve female adult virgin rabbits were housed in metabolic cages to record voiding and defecation. Anesthetized CMG/EMG was performed before and after treatment animals (n=9) received bilateral tetanizing needle stimulation to the pubococcygeous (PC) muscle and controls (n=3) sham needle placement. After 7 days all animals were subjected to tetanizing transvaginal stimulation and CMG/EMG. After 5 days a final CMG/EMG was performed.
Results
Of rabbits that underwent needle stimulation 7 of 9 (78%) demonstrated dysfunctional CMG micturition contractions versus 6 of 12 (50%) after transvaginal stimulation. Needle stimulation of the PC musculature resulted in significant changes in: basal CMG pressure, precontraction pressure change, contraction pressure, interval between contractions and postvoid residual; with time to 3rd contraction increased from 38 to 53 minutes (p=0.008 vs. prestimulation). Vaginal noxious stimulation resulted in significant changes in: basal CMG pressure and interval between contractions; with time to 3rd contraction increased from 37 to 46 minutes (p=0.008 vs. prestimulation). Changes in cage parameters were primarily seen after direct needle stimulation.
Voiding dysfunction due to pelvic floor overactivity in the human may be characterized by a dysfunctional inability to relax or a paradoxical tightening when attempting to void [1]. In this setting, a hypertonic pelvic floor may result in increased bladder outlet resistance, bladder outlet obstruction and ultimately bladder sensitization [2]. In the setting of bladder sensitization and dysfunctional behavior of the pelvic floor, dysfunction may be mediated by crosstalk along the sacral nerves and further mediated by the central nervous system [34]. Pelvic floor dystonia is often seen in the clinical setting of overactive bladder, and treatment of the pelvic floor is becoming more widely accepted in addressing the overall clinical phenotype of overactive bladder, however the literature is still emerging [5].
Transvaginal electrical stimulation and pelvic floor muscle training are commonly used in humans as conservative therapies for both female stress urinary incontinence and overactive bladder symptoms [6] and appear to be effective at various levels of electrical stimulation [7]. When used to treat overactive bladder related to a hypertonic pelvic floor, the therapies aim at relaxing the levators [8]. Low intensity pelvic floor stimulation appears to improve pelvic floor dystonia as well as postpartum dyspareunia [9].
There has been a paucity of animal models for chronic pelvic floor dystonia in which to examine the mechanisms for the levator, bladder and bowel relationship seen in the human clinical phenotype of pelvic floor dysfunction. Previous studies have demonstrated that multiparity in rabbits is associated with uncoordinated activity of the pelvic muscles [10], as well as stress urinary incontinence [11] which may be a result of pelvic muscle stretch injury [12]. More recently, we developed a short term model of pelvic floor dystonia in the rabbit [13]. This experiment demonstrated that direct noxious tetanizing electrical stimulation of the pubococcygeous (PC) muscle using an electromyographic (EMG) needle resulted in cystometric (CMG) and EMG changes suggestive of voiding dysfunction; a larger bladder capacity, longer interval between contractions and prolonged contraction duration [13]. Our current experiment aims to further develop our acute model into a survival model and explore the long term effects of needle and transvaginal noxious tetanizing stimulation on rabbit voiding and defecatory behavior.
All methods were approved by our Institutional Animal Care and Use Committee (IACUC, Stratton VA Medical Center, Albany, NY, USA). Female adult virgin white New Zealand rabbits, aged 4 to 5 months and approximately 3.5-kg body weight, were chosen for their well developed pelvic floor musculature. Twelve rabbits were housed in metabolic cages to record baseline voiding and defecation for 3 days as outlined by the treatment timeline (Fig. 1). Metabolic cage floors measured 74 cm by 74 cm, with a cage screen to collect feces and a sloped funnel below the cage to collect urine into a computerized recording collection container. After completing baseline metabolic cage measurements, on day 4 animals were anesthetized using ketamine/xylazine sedation and a baseline CMG/EMG #1 was obtained using XLTEC NeuroMax 1002 machine (Natus Medical Inc., Oakville, ON, Canada). Continuous filling of the bladder was performed at a rate of 4 mL/min until the rabbit's third productive contraction, with subsequent measurement of postvoid residual (Fig. 2). Contractions were graded as either normal or dysfunctional (Fig. 3). Both left and right PC muscle groups were located using EMG needles with a single confirmatory test stimulation (7.5 mA, 0.1 ms) to demonstrate the characteristic ipsilateral abduction of the tail, vagina, and rectum, thus confirming placement as described by other investigators [14]. Left and right needles were then used to obtain EMG data as well as administer treatment stimulation. Animals were previously randomized by cage order to receive either treatment or sham needle placement. Treatment animals (n=9) received bilateral tetanizing noxious needle stimulation (4 trains 10 seconds apart, 15 mA, 25 Hz, 0.2 ms, 10 pulses/train) to the PC muscle and controls (n=3) bilateral sham needle placement. After completing treatment, all animals were then subjected to a postnoxious stimulation CMG/EMG #2 as previously described.
Animals were then placed back into their metabolic cages for 7 days to record defecation and voiding parameters (Fig. 1). At the end of this period, on day 11 animals were then anesthetized and a baseline CMG/EMG #3 was obtained as previously described. All animals were then subjected to tetanizing transvaginal stimulation (5 minutes, 6.5 mA, 10 Hz, 0.1 ms repetitive) using a smooth insulated vaginal probe to localize electrical current to the deep vagina with characteristic production of rhythmic vaginal wall and pelvic floor contractions (Fig. 2). After completing transvaginal stimulation a postvaginal stimulation CMG/EMG #4 was obtained. Contractions were graded as either normal or dysfunctional (Fig. 3).
Animals were then placed back into their metabolic cages for 5 days to record defecation and voiding parameters. At the completion of cage measurements animals were then anesthetized and a final CMG/EMG #5 was performed. Animals were then euthanized using phenobarbital in accordance with local IACUC guidelines.
Metabolic cage data was recorded for each animal and included measurements of voided volume (mL), time of void, number of voids in 24 hours and daily fecal weight (g). Descriptive statistics were used to calculate mean, standard error of mean (SEM) and define 95% confidence intervals. Animal cage data was grouped according to the day 4 treatment allocation (needle noxious stimulation [n=9] versus sham needle placement [n=3]), and evaluated using repeated measures analysis of variance (ANOVA) with a least squares estimation method.
Cystometry data was recorded for each animal and included measurement of the baseline CMG pressure (basal CMG pressure [cmH2O]) at the start of bladder filling, the change from baseline pressure to the pressure at which spontaneous micturition occurred (precontraction pressure change [cmH2O]), the maximum CMG pressure during productive micturition (contraction pressure [cmH2O]), the intercontractile interval (interval between contractions [min]), the time from start of bladder filling until the third productive contraction (time to 3rd contraction [min]), volume infused into bladder at time of the first productive contraction (capacity [mL]) and the catheterized volume of urine remaining in the bladder after the completion of the third productive contraction (postvoid residual [mL]). Mean, SEM and Student t-test were used to make comparisons between animals and treatment groups. A p-value of less than 0.05 was defined as statistically significant.
Throughout the experiment, both the total 24-hour fecal weight and total 24-hour urine volume were similar between animals. Cage data was grouped according to the day 4 treatment allocation with a summary of 24-hour urinary frequency and mean volume per void presented as a 17-day box plot for sham needle placement versus noxious PC stimulation groups (Fig. 4). At baseline from day 1 to 3, there was no significant difference between treatment groups noted for the number of voids in 24 hours or mean volume per void.
On day 4, animals were anesthetized and CMG/EMG #1 was performed with baseline CMG data revealing no significant difference in cystometry parameters between treatment groups (Table 1). Following noxious needle stimulation of the pelvic floor PC muscle, CMG/EMG #2 demonstrated a significant change in basal CMG pressure, precontraction pressure change, contraction pressure, interval between contractions, time to 3rd contraction and postvoid residual (Table 1). CMG response to needle noxious stimulation was heterogeneous when comparing animal groups, however when evaluating each animal individually there was an obvious trend towards an increased interval between contractions with a longer interval noted after needle tetanizing stimulation of the PC muscle and minimal change noted after sham needle placement alone (Table 1). After grouping all animals according to treatment group, needle tetanizing stimulation of the PC muscle significantly prolonged interval between CMG contractions with mean time to third contraction rising from 38 to 53 minutes (p=0.008 vs. prestimulation) (Table 1).
On day 11, animals were anesthetized and CMG/EMG #3 was performed and data was initially analyzed according to day 4 treatment allocation. Two animals from the day 4 treatment group only demonstrated one prolonged broad contraction that was evaluable. Despite this finding, baseline CMG data revealed no significant difference in cystometry parameters between treatment groups when grouped according to day 4 treatment allocation (Table 2). Following noxious transvaginal stimulation in all animals on day 11, the poststimulation CMG/EMG #4 did not demonstrate three productive contractions in two animals. In these two animals, which were previously in the day 4 control group, only a single evaluable spiking contraction was noted in one animal and and two broad prolonged contractions in the other. Data for the available demonstrated and evaluable contractions from all 12 animals was included for analysis (Table 2). Following vaginal noxious stimulation, CMG/EMG #4 demonstrated a significant change in basal CMG pressure, interval between contractions and time to 3rd contraction (Table 2).
Animals were also grouped into a single cohort (n=12) for evaluation and comparison of baseline CMG/EMG #3 to the post stimulation CMG/EMG #4 following noxious vaginal stimulation (Table 3). This analysis was consistent with Tables 1, 2, and in a manner similar to pelvic floor tetanizing stimulation, transvaginal stimulation signif icantly increased time to third contraction from 37 to 46 minutes (p=0.008 vs. prestimulation baseline CMG/EMG #3) (Table 3). Postvoid residual urine volume was not significantly different between groups before and after needle or vaginal stimulation.
Heterogeneous voided volume and frequency behavior was noted at baseline and after both direct needle pelvic floor PC muscle stimulation and vaginal noxious electrical stimulation. Using repeated measures ANOVA to calculate changes within each animal and between treatment groups a statistically significant increase in the number of voids was noted for 24 hours following the day 4 needle noxious PC pelvic floor stimulation (ANOVA p-value=0.011 vs. control) (Fig. 4). No statistically significant change was seen in cage parameters beyond one day following needle noxious stimulation of the pelvic floor however there was a trend towards the treatment animals exhibiting increased urinary frequency and smaller voided volumes (Fig. 4). Following the day 11 noxious vaginal stimulation there was also a trend towards increased urinary frequency and decreased mean voided volume, however data was heterogeneous and these changes were not statistically significant between animals or treatment groups.
On day 18 animals CMG/EMG #5 was performed and animals were euthanized. One animal from the day 4 treatment group had only one CMG contraction available for interpretation. This final CMG evaluation revealed a statistically significant increase in contraction duration and postvoid residual in the noxious pelvic floor stimulation animals when grouped according to day 4 treatment allocation (Table 4).
Despite the amount of heterogeneity among animals, of those that underwent needle stimulation, seven of nine animals (78%) demonstrated CMG contractile patterns of voiding dysfunction versus six of twelve animals (50%) after transvaginal stimulation, with little durable change in cage parameters several days following either modality of stimulation.
A major challenge with diagnosing pelvic floor dysfunction can be the variability of the clinical presentation. Pelvic floor dysfunction can either be a result of a hyper- or hypotonic pelvic floor and can manifest as symptoms related to the lower urinary tract, chronic pelvic pain, dysfunctional voiding or dyspareunia [15]. The lower urinary tract symptoms in patients with dystonia of the pelvic muscles is also quite variable and may present as storage symptoms in one patient with a complaint of urinary frequency and urgency, whereas another patient might paradoxically complain of obstructive voiding symptoms.
In our experiment, we observed dysfunctional CMG contractions in a majority of animals as accompanied by a larger bladder capacity, longer interval between contractions and prolonged contraction duration. However not all animals developed this phenotype, with only half of animals demonstrating these voiding changes after transvaginal stimulation. The importance of the pelvic floor in modulating voiding function cannot be understated. In our experiment we found that both direct PC needle and as well as transvaginal electrical stimulation resulted in prolonged intervals between CMG contractions. Most of the changes in cage parameters were primarily seen after direct needle stimulation of the pelvic floor. This was likely due to the mechanism that needle stimulation delivered electrical current directly to the pelvic floor when compared to the transvaginal route.
Our study has several limitations including small sample size, heterogeneity between animals and difficulty in delivering stimulation exactly the same between animals. Despite the small sample size and heterogeneity of voiding patterns between animals, the majority of subjects had excellent internal consistency. An additional consideration is the degree of sedation and analgesia while under anesthesia. Ketamine was used to sedate animals during cystometry and is recognized to alter CMG parameters, however the relative difference in CMG parameters should have been effectively the same since all animals were sedated according to body weight during each cystometrogram. Pelvic pain was also difficult to assess in our experiment and may be a symptom of pelvic floor spasm and other inciting events [4].
The exact mechanism of action in our experiment can only be hypothesized. Similar to pain, in our experiment we were not able to directly measure pelvic floor tone. Coordinated pelvic floor muscle tone in rabbits has been previously demonstrated to modulate micturition [16]. Based on our CMG findings of increased EMG activity noted in the dysfunctionally voiding animals, it is postulated that either detrusor contractile force increased versus an increase in bladder outlet resistance, however no increase in contraction pressure was noted following noxious stimulation so it is suspected that noxious stimulation of the PC muscle directly affected the pelvic floor and thus altered urethral pressure. This hypothesis into the mechanism of our experiment is consistent with the findings of Rajasekaran et al. [17] whereupon a dose dependent increase in urethral pressure was demonstrated following electrical stimulation of the puborectalis muscle of the rabbit pelvic floor. However despite our EMG evidence of markedly increased paradoxical activation of the PC muscle during voiding, as an additional limitation to our study, we did not directly measure urethral pressure during voiding and can only speculate that pelvic floor tone was increased during the voiding phase of micturition in a pattern consistent with dysfunctional voiding. Despite this limitation to the generalizability of our findings, these results build upon those of previous investigators and provide one more clue to the role of the pelvic floor following noxious stimulation.
Future research into our model of pelvic floor dysfunction after noxious stimulation of the vagina and PC should be directed at addressing the aforementioned limitations and investigate pathways to further elucidate the therapeutic effects of subnoxious levels of stimulation, much like would be used in transvaginal electrical stimulation in humans.
In a majority of animals, tetanizing electrical stimulation of the rabbit pelvic floor PC musculature resulted in voiding changes suggestive of pelvic floor dysfunction as characterized by a larger bladder capacity, longer interval between contractions and prolonged contraction duration.
Figures and Tables
Fig. 3
Representative cystometric bladder contractions. (A) Normal contraction with slow rise in pressure accompanied by productive void and (B) dysfunctional contraction with high pressure and prolonged duration poorly productive void.
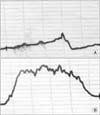
Fig. 4
Box plot of day 1 to day 17 mean 24-hour urinary frequency and mean volume per void for animals grouped according to day 4 treatment allocation (sham needle placement [control, n=3] versus needle noxious PC pelvic floor stimulation [treatment, n=9]). **p-value=0.011 versus control (repeated measures analysis of variance).
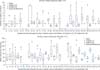
Table 1
Day 4 CMG parameters before and after PC pelvic floor stimulation
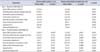
Table 2
Subgroup analysis of day 11 CMG parameters before and after vaginal stimulation
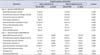
Table 3
Day 11 CMG parameters before and after vaginal noxious stimulation
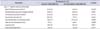
Table 4
Subgroup analysis of Day 18 CMG parameters immediately prior to euthanasia
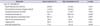
ACKNOWLEDGMENTS
This project was funded by the Capital Region Medical Research Foundation & Stratton VA Research and Development Office.
References
1. Messelink B, Benson T, Berghmans B, Bo K, Corcos J, Fowler C, et al. Standardization of terminology of pelvic floor muscle function and dysfunction: report from the pelvic floor clinical assessment group of the International Continence Society. Neurourol Urodyn. 2005; 24:374–380.
2. Ustinova EE, Fraser MO, Pezzone MA. Cross-talk and sensitization of bladder afferent nerves. Neurourol Urodyn. 2010; 29:77–81.
3. Malykhina AP. Neural mechanisms of pelvic organ crosssensitization. Neuroscience. 2007; 149:660–672.
4. Rudick CN, Chen MC, Mongiu AK, Klumpp DJ. Organ cross talk modulates pelvic pain. Am J Physiol Regul Integr Comp Physiol. 2007; 293:R1191–R1198.
5. Abramowitch SD, Feola A, Jallah Z, Moalli PA. Tissue mechanics, animal models, and pelvic organ prolapse: a review. Eur J Obstet Gynecol Reprod Biol. 2009; 144:Suppl 1. S146–S158.
6. Barroso JC, Ramos JG, Martins-Costa S, Sanches PR, Muller AF. Transvaginal electrical stimulation in the treatment of urinary incontinence. BJU Int. 2004; 93:319–323.
7. Alves PG, Nunes FR, Guirro EC. Comparison between two different neuromuscular electrical stimulation protocols for the treatment of female stress urinary incontinence: a randomized controlled trial. Rev Bras Fisioter. 2011; 15:393–398.
8. Brubaker L, Benson JT, Bent A, Clark A, Shott S. Transvaginal electrical stimulation for female urinary incontinence. Am J Obstet Gynecol. 1997; 177:536–540.
9. Dionisi B, Senatori R. Effect of transcutaneous electrical nerve stimulation on the postpartum dyspareunia treatment. J Obstet Gynaecol Res. 2011; 37:750–753.
10. Martinez-Gomez M, Mendoza-Martinez G, Corona-Quintanilla DL, Fajardo V, Rodriguez-Antolin J, Castelan F. Multiparity causes uncoordinated activity of pelvic- and perinealstriated muscles and urodynamic changes in rabbits. Reprod Sci. 2011; 18:1246–1252.
11. Gill BC, Moore C, Damaser MS. Postpartum stress urinary incontinence: lessons from animal models. Expert Rev Obstet Gynecol. 2010; 5:567–580.
12. Fajardo V, Pacheco P, Hudson R, Jimenez I, Martinez-Gomez M. Differences in morphology and contractility of the bulbospongiosus and pubococcygeus muscles in nulliparous and multiparous rabbits. Int Urogynecol J Pelvic Floor Dysfunct. 2008; 19:843–849.
13. Spettel S, Levin R, Dubin A, De E. Animal model of pelvic floor dystonia and cystometric effects on urinary dysfunction. In: Society for Urodynamics and Female Urology, 2012 Winter Meeting; 2012 Feb 28-Mar 3; New Orleans (LA), USA. Neurourol Urodyn. 2012; 31(2):234.
14. Martinez-Gomez M, Lucio RA, Carro M, Pacheco P, Hudson R. Striated muscles and scent glands associated with the vaginal tract of the rabbit. Anat Rec. 1997; 247:486–495.
15. Butrick CW. Pelvic floor hypertonic disorders: identification and management. Obstet Gynecol Clin North Am. 2009; 36:707–722.
16. Corona-Quintanilla DL, Castelan F, Fajardo V, Manzo J, Martinez-Gomez M. Temporal coordination of pelvic and perineal striated muscle activity during micturition in female rabbits. J Urol. 2009; 181:1452–1458.
17. Rajasekaran MR, Sohn D, Salehi M, Bhargava V, Fritsch H, Mittal RK. Role of puborectalis muscle in the genesis of urethral pressure. J Urol. 2012; 188:1382–1388.