Abstract
Purpose
Curcumin is a nontoxic, chemopreventive agent possessing multifaceted functions. Our previous study showed that curcumin inhibits androgen receptor (AR) through modulation of Wnt/β-catenin signaling in LNCaP cells. Therefore, we investigated the in vivo effects of curcumin by using LNCaP xenografts.
Materials and Methods
LNCaP cells were subcutaneously inoculated in Balb/c nude mice. When the tumor volume reached greater than 100 mm3, either curcumin (500 mg/kg body weight) or vehicle was administered through oral gavage three times weekly for 4 weeks. The expression of AR and intermediate products of Wnt/β-catenin were assessed.
Results
Curcumin had an inhibitory effect on tumor growth during the early period, which was followed by a slow increase in growth over time. Tumor growth was delayed about 27% in the curcumin group. The mean prostate-specific antigen (PSA) doubling time in the curcumin group was approximately twice that in the untreated group. Curcumin significantly decreased AR expression at both the mRNA and protein level. The PSA levels tended to be reduced in the curcumin group. However, there were no significant changes in expression of Wnt/β-catenin pathway intermediates.
Androgen receptor (AR) is known to play a crucial role in the development and progression of prostate cancer. Upon binding to androgen, the AR undergoes translocation to the nucleus and acts as a transcription factor [12]. Beta-catenin, one of the potent coactivators of the AR, interacts directly with the receptor to stimulate AR-mediated gene transcription. A mutual synergistic relationship between the AR and β-catenin pathways has been well documented [345]. Recent studies have demonstrated that Wnt/β-catenin signaling plays an important role in prostate cancer. In addition, growing evidence indicates the aberrant activation or localization of β-catenin as a prognostic biomarker in prostate cancer [678910].
Chemoprevention with dietary compounds is an alternative, nontoxic modality in prostate cancer treatment because of a long latency period, a high prevalence rate in Western countries, tumor marker availability, and identifiable precancerous lesions [11]. Recently, the issue regarding the burden of prostate cancer treatment and active surveillance has emerged as a major concern. Therefore, the use of chemopreventive agents appears to be an attractive strategy to decrease the incidence and slow the progression of this disease.
Curcumin, a major yellow pigment of turmeric (Curcuma longa), has been actively studied for its chemopreventive property in several types of cancers. Numerous studies have confirmed the anticarcinogenic activity and potential chemoprophylactic value of curcumin [12131415]. Curcumin was shown to down-regulate AR gene expression as well as prostate-specific antigen (PSA) in the LNCaP cell line [111617]. This antiandrogenic action is expected to serve as a promising chemopreventive effect. However, the mechanism by which curcumin regulates AR expression in prostate cancer is poorly understood. We previously demonstrated that curcumin modulates the Wnt/β-catenin pathway and androgen signaling in vitro [18]. However, whether curcumin regulates the expression of AR and Wnt/β-catenin target genes in in vivo remains unclear. In this study, we assessed the effects of curcumin on the interaction between the AR and Wnt/β-catenin pathways by using an androgen-dependent LNCaP prostate cancer model.
LNCaP cells (American Type Culture Collection, Rockville, MD, USA) were routinely cultured in Rosewell Park Memorial Institute 1640 medium supplemented with 10% fetal bovine serum. Cell cultures were maintained at 37℃ in a humidified atmosphere of 5% CO2. The cells were grown until 80% to 90% confluence was achieved. Cells for subcutaneous injection were used when a single cell suspension was greater than 90% viability as determined by trypan blue exclusion. Curcumin (≥95% purity) was obtained from Sigma-Aldrich (St. Louis, MO, USA). Matrigel was purchased from BD Biosciences (Bedford, MA, USA). Antibodies against AR, β-catenin, and β-actin were purchased from Cell Signaling (Beverly, MA, USA). Antibody for cyclin D1 was a product of Santa Cruz Biotech (Santa Cruz, CA, USA).
Six-week-old male Balb/c nude mice (Charles River Laboratories Japan, Yokohama, Japan) were maintained in accordance with the Guide for the Care and Use of Laboratory Animals of the US National Research Council. The animal research was performed after receiving approval of the Institutional Animal Care and Use Committee of the Samsung Biomedical Research Institute. LNCaP tumors were grown subcutaneously in the mice as previously described [19]. Briefly, LNCaP cells (3×107 cells/mL) were suspended in equal volumes of Matrigel and sterile Dulbecco's phosphate-buffered saline. Mice were injected subcutaneously with 150 µL of the cell suspension into the flank region. LNCaP xenograft mice were allowed to grow for 7 weeks after inoculation. The volume of each tumor was measured biweekly when the tumors were palpable according to the following formula: 4/3π×1/2×r12×r2 (r1<r2). Animals with an accidental death or insufficient tumor development were excluded from the calculations. When the tumor volume reached about 100 mm3, mice were randomly assigned into two groups receiving either curcumin or the vehicle control. Curcumin was emulsified in a 0.9% saline solution and Tween 20 at a dose of 500 mg/kg body weight. Curcumin or the vehicle was administered by oral gavage three times weekly for 4 weeks. Four weeks after treatment, the mice were sacrificed. Tumors were excised and weighed. Each tumor specimen was divided into three approximately equal portions: one part was fixed in buffered formalin and the other parts were frozen in liquid nitrogen and maintained at -80℃ until further processing. Serum was consecutively obtained through the dorsal tail vein immediately before tumor cell implantation, at randomization, and finally at the time of tumor harvest. The serum samples were also stored. Tumor growth delay was calculated as follows: [(T-C)/C]×100, where T and C are the median times to specified tumor size for the treated (T) and control (C) groups, respectively [20].
Total RNA was isolated from each tumor tissue sample by using TRIzol Reagent (Invitrogen, Carlsbad, CA, USA). The ImProm-II Reverse Transcription System (Promega, Madison, WI, USA) was used to prepare cDNA. We designed the polymerase chain reaction (PCR) primers (Table 1). To estimate absolute quantification of mRNA expression, standard calibration curves were used from dilution series of the control plasmids as previously described [21]. Then, to obtain constructs for plasmid controls to quantify the targeted mRNA (pβ-catenin, pcyclin D1, pAR, pPSA, and pβ-actin), partial cDNAs were amplified and then cloned into pGEM-T Easy Vector (Promega) according to the manufacturer's instructions. Finally, the sequences of the cloned plasmids were checked with automatic sequencing processes.
Two microliters of the standard plasmid was added to the 25-µL reaction mixture containing forward primer (300nM), reverse primer (300nM), 0.5 units of uracil-N-glycosylase (Sigma-Aldrich), and 2× QuantiTech SYBR Green PCR Master Mix (Qiagen GmbH, Hilden, Germany). Real-time PCR was performed in Rotor Gene 6.0 (Corbett, Sydney, Australia) according to the manufacturer's instructions. Amplification consisted of 2 minutes at 50℃ for carryover prevention, 15 minutes at 95℃, 40 cycles through a denaturation step at 95℃ for 10 seconds, and an annealing-elongation step at 52℃ for 15 seconds and 72℃ for 20 seconds. Melting curve analysis and the desired value of concentration was achieved. Negative controls were also included. The detected critical threshold values were interpolated into calibrated equations from the standard curves of plasmid constructs, and the concentration of each mRNA in the samples was calculated.
Tumor samples were lysed in radio immunoprecipitation assay buffer (Pierce, Rockford, IL, USA) with protease inhibitor. The protein concentration was measured with the Bradford assay (Bio-Rad, Hercules, CA, USA). Sixty micrograms of total protein was loaded onto each lane and electrophoresis was carried out. After electrophoresis, protein was transferred to a polyvinylidene difluoride membrane (Millipore, Bedford, MA, USA). The membrane was incubated overnight at 4℃ with one of the following primary antibodies: anti-AR antibody (1:700), anti-β-catenin antibody (1:1000), or anti-cyclin D1 antibody (1:700). Specific antibody binding was detected by use of antirabbit IgG antibody conjugated with horseradish peroxidase diluted 1:2000 in blocking buffer. The protein of interest was detected by enhanced chemiluminescence using enhanced chemiluminescence plus (Amersham, Arlington, IL, USA) followed by autoradiography.
Formalin-fixed, paraffin-embedded serial sections (5 µm) were obtained from tumor samples. The slides were dewaxed and rehydrated and antigen retrieval was performed by microwaving sections with Target Retrieval Solution (pH 6.0; Dako, Carpinteria, CA, USA). Following washing with Tris-buffered saline, sections were incubated with specific primary antibodies. Rabbit polyclonal antibodies against the AR (1:50), PSA (1:80), β-catenin (1:200), and cyclin D1 (1:25) were used. The slide sections were then studied by using an EnVision+Dual Link Kit (Dako, Glostrup, Denmark) and were counterstained with hematoxylin. Immunohistochemical staining was evaluated by an experienced pathologist (D.H.K.) without any knowledge of the experimental data. Positive staining was defined as a dark brown reaction on nuclear or cytoplasmic staining. The intensity of staining was scored on a scale of 0 to 5 (0, no staining; 1, minimal staining; increasing to 5 for maximum intensity). For clarity of interpretations, the staining was then semiquantitatively classified into absent (0), weak (1-2), moderate (3), and strong (4-5) intensity in each subcellular compartment [9]. Three nonoverlapping measurements, including the most intense staining area, were made at high magnification (×400) in each slide.
Serum levels of total PSA were determined by enzyme-linked immunosorbent assay by following the procedures provided by the manufacturer (Diagnostic Systems Laboratory, Webster, TX, USA). Briefly, 2.5 µL of serum diluted to a final volume of 75 µL was added to duplicate wells in a 96-well plate that had been coated with anti-PSA antibody. Following 1 hour of incubation at 25℃, each well was treated with a second anti-PSA antibody labeled with horseradish peroxidase. The reaction was stopped and the absorbance was measured at 450 nm by using a VERSAMax microplate reader (Molecular Devices, Sunnyvale, CA, USA). PSA doubling time (PSA DT) was calculated by using the log-slope method, which uses a linear model to estimate the slope of the log-transformed PSA levels and time, as follows: PSA DT=log2×(t2-t1)/logPSA(t2)-logPSA(t1), with PSA obtained at times t1 and t2, respectively (t2>t1) [2223].
Data are presented as the median with 95% confidence interval (CI) or mean±standard error of mean. All experiments were carried out in triplicate. The statistical differences were analyzed by Mann-Whitney U test, Wilcoxon signed rank test, and Fisher exact test. A p-value less than 0.05 was considered significant. Analysis of the data was performed by using IBM SPSS Statistics ver. 21.0 (IBM Co., Armonk, NY, USA).
A total of 40 mice were used when the experiment was initiated. Mice in which the tumors failed to reach a sufficient volume (n=13) or mice that failed to survive during the study period (n=7) were excluded. Therefore, 20 mice (n=10 in each group) were finally analyzed. The median tumor volumes in the control and curcumin groups at baseline were 283.0 mm3 (95% CI, 162.1-390.8) and 256.9 mm3 (95% CI, 149.3-349.3), respectively. Tumor volume was analyzed weekly. The tumor growth over time for each mouse in the control and treated groups is shown in Fig. 1A. The growth pattern seemed to differ between the two groups during the first half of the period. To assess significant differences in tumor volumes at each time point, the slopes of the tumor growth curves are presented (Fig. 1B). Tumor volume did not differ significantly between the control and curcumin groups. However, curcumin had a suppressive effect on tumor growth during the early period of treatment, which was followed by a slow increase in tumor growth in the remaining period. Conversely, in the control group, the tumor consistently increased compared with the baseline volume at week 7. Although the effect of curcumin on the growth of tumor xenografts was not statistically significant, a trend toward initial inhibition of tumor growth was observed. We then determined whether curcumin would affect the tumor growth delay once the tumors had already grown. The median tumor volume of the total mice at the midpoint of treatment was 349 mm3. Therefore, we defined a specific tumor size as 350 mm3 to calculate tumor growth delay. Median times to reach a volume of 350 mm3 for the control and curcumin-treated groups were 7.7 and 9.8 weeks, respectively. Tumor growth delay was calculated to be 26.9%. This means that the tumor growth was delayed about 26.9% in the treated group compared with the control group.
Next, we compared the harvested tumor weight (Fig. 1C, D). The median tumor weights in the control and curcumin groups were 300.0 mg (95% CI, 160.0-490.0) and 265.0 mg (95% CI, 185.0-400.0), respectively. There was no significant difference in overall tumor weight between the groups. However, the interquartile range in the controls was comparatively wider than in the curcumin group. This means that half of the observed values around the median had more dispersion in distribution.
To determine whether curcumin could inhibit the expression of PSA in vivo, serum samples were collected from each mouse. PSA expression in the group treated with curcumin was not significantly lower than that in the control group at either time point (Fig. 2A). Our earlier data showed that mice treated with curcumin showed a tendency for slow tumor growth compared with control mice. Next, we determined whether curcumin affected PSA kinetics in the form of the PSA DT. We chose two measurements of PSA at the time of randomization and tumor harvest, each separated by 4 weeks. The mean time needed for the PSA value to double in the control and curcumin group was 2.9±0.8 weeks and 6.0±1.8 weeks, respectively (Fig. 2B). The mean PSA DT in the curcumin group was approximately two times that in the control group, although this difference was not significant (p=0.150). More time would be required for the PSA value to double in the curcumin group.
To determine whether curcumin had any effect on the AR pathway or changed the expression of the Wnt/β-catenin signaling products, we prepared tumor samples and subjected them to quantitative real-time PCR, Western blotting, and immunohistochemistry.
A good log-linear relationship between the cycle threshold and the corresponding cDNA levels of control plasmids was demonstrated (Fig. 3A). The mRNA expression of all samples was calculated following an adjustment of each β-actin mRNA expression level (Fig. 3B). Curcumin treatment significantly decreased the transcriptional activity of AR mRNA (p=0.011). Although it was not as clear as for the AR, the expression of PSA tended to be reduced in the curcumin-treated group (p=0.123). In contrast, the changes in mRNA expression of the Wnt/β-catenin products including β-catenin and cyclin D1 were not as distinct.
Immunoblot analysis demonstrated that the protein expression of AR was decreased in the curcumin-treated mice (Fig. 4). Therefore, curcumin significantly inhibited AR expression at both the mRNA and protein levels. However, no significant changes in the protein expression of Wnt/β-catenin pathway intermediates between the control and curcumin-treated mice were observed. Immunohistochemistry showed that there was no notable difference in the overall expression of AR, PSA, β-catenin, and cyclin D1 between the control and curcumin groups (Fig. 5). There was a small decrease in AR and PSA staining intensity in the curcumin-treated group. Thus, our in vivo study on the effect of curcumin showed that curcumin interferes with prostate cancer growth involving the inhibition of AR activity and a possible reduction of PSA expression. However, our results failed to verify directly the hypothesis that curcumin modulates the Wnt/β-catenin pathway through AR/β-catenin interaction.
During the last decades, epidemiologic studies have indicated the importance of dietary patterns in the prevention of prostate cancer. With this in mind, the role of chemoprevention by phytochemicals has come to the forefront. Several naturally occurring compounds are currently being investigated for their chemopreventive potential [24]. Curcumin has been used as an empirical remedy in the treatment of a variety of inflammatory conditions and chronic diseases. Many of its traditional properties, including its anticarcinogenic effect, have been studied [12131415]. Curcumin is remarkably well tolerated in animals and humans, even at high doses. Several phase I studies of curcumin have been reported, and no dose-limiting toxicities were observed at single daily oral doses ranging from 500 to 12,000 mg [1213]. Although the mechanism associated with curcumin in prostate cancer has not been fully elucidated, it is plausible that the effects are at least in part on the AR [1618]. The Wnt signaling pathway is physiologically implicated in tissue development and maintenance. Dysregulation or aberrant activation of the Wnt/β-catenin pathway is responsible for tumorigenesis of various types of cancer. Activation of the AR depends on several coactivators including β-catenin, a well-known downstream effector of the Wnt pathway. Reciprocal interaction of the AR and β-catenin has been clearly demonstrated [345]. Previous studies have described that prostate cancer progression may be associated with altered β-catenin expression [678910]. But, so far, very little research has focused on Wnt/β-catenin signaling to identify the mechanism of action of curcumin in prostate cancer [182526]. Moreover, little is known regarding the effect of curcumin on the Wnt/β-catenin pathway in vivo. We previously found that curcumin inhibits AR expression in LNCaP cells in a dose-dependent manner. We also identified that curcumin interrupts Wnt signaling by decreasing β-catenin activity, which in turn suppresses the expression of β-catenin target genes (cyclin D1, c-myc). This may be one mechanism by which curcumin can affect LNCaP cell proliferation through modulation of Wnt/β-catenin and androgen signaling [18]. Therefore, we further investigated whether oral curcumin intake would repress prostate cancer growth by interfering with AR and Wnt/β-catenin signaling in an LNCaP xenograft model.
Assessment of the antitumor activity of a tested agent in a tumor xenograft experiment can be demonstrated by using a variety of mathematical approaches such as growth curve pattern, tumor growth delay, tumor doubling time, and log10 cell kill [27]. Our results showed that curcumin had an inhibitory effect on tumor growth during the early period of treatment followed by a slow increase. A trend toward suppression of tumor growth was observed during the whole period, but it was not statistically significant. In the curcumin-treated group, tumor growth was delayed about 27% to reach the targeted volume of 350 mm3. Thus, curcumin appeared to interfere with tumor growth at least during the early phase, but then its influence was somewhat reduced. PSA (also known as kallikrein III) is a 34-kD glycoprotein produced almost exclusively by the prostate gland. PSA was first measured quantitatively in the blood in 1980 and has been used as a clinical biomarker of prostate cancer. Measurement of PSA at follow-up after therapy is an exquisitely sensitive prognosticator for residual or recurrent tumors. Predictors of prognosis have been investigated so that patients who have undergone definitive treatment might be stratified in terms of possible biochemical recurrence.
PSA kinetics in the form of the PSA DT is defined as the time needed for the PSA to double. The PSA DT has been shown to be a reliable predictor of prognosis for the follow-up of prostate cancer after curative therapy [23]. Therefore, we investigated whether curcumin affected PSA kinetics. We introduced the PSA DT calculation to adjust the PSA change in both treatment arms. The mean PSA DT in the curcumin-treated group was approximately two times that in the control group. This means that it would take more time for the PSA to double in the curcumin group. These findings suggest that curcumin may slow down prostate tumor growth and result in incremental changes in the PSA value initially.
To identify whether curcumin had any effect on the products of the AR and Wnt/β-catenin signaling pathways, further experiments were performed. Our study revealed that curcumin significantly decreased AR expression at both the mRNA and protein levels. In addition, PSA tended to be reduced in the curcumin-treated group. However, there were no distinct changes in the expression of Wnt pathway intermediates including β-catenin and cyclin D1. Given these results, it seems likely that the main antiandrogenic mechanism of curcumin may not be directly linked with the AR/β-catenin interaction.
Previously, we showed that curcumin can interfere with LNCaP cell growth through a Wnt/β-catenin and AR interaction. However, subsequent in vivo experiments showed that the antiproliferative effect on tumor growth was not striking and failed to replicate the in vitro results. This may be explained as follows. All inoculated mice developed visible tumors, confirming the high rate of tumor uptake in this study. Even so, an analysis of antitumor activity has some limitations. First, because a relatively small number of mice were selected for testing, skewed distributions of tumor volume and various patterns of tumor growth were observed. In addition, data may have been missing for death or insufficient tumor development before termination could be issued. Excluding animals with missing observations may result in a biased conclusion. Second, study of the role of curcumin has focused on its chemopreventive action rather than its therapeutic properties. Therefore, even if curcumin has some inhibitory effects on tumor growth, its influence may be minimal or weaker than expected after a significant tumor volume is attained. If the experiment is aimed at studying the prevention of tumor development for an unproven chemopreventive agent, it may be reasonable to treat the mice before documentation of tumor growth. However, the need for large numbers of mice with high rates of tumor development should be considered. Third, among the factors affecting the anticancer effect, both bioavailability and required exposure times are important [27]. Although curcumin has attracted attention because of its safety, multitargeted anticancer effects, and easy accessibility, the actual application of curcumin is currently compromised by its poor absorption and low systemic bioavailability [1213]. Intake of curcumin at a dietary level of 0.1%, approximately equal to 150 mg/kg per day, lacks overall efficacy, whereas intake at 0.2%, which equates to 300 mg/kg per day, has been shown to retard adenomas in a mouse model of familiar adenomatous polyposis [28]. We chose an oral dose of curcumin at a concentration of 500 mg/kg three times weekly. Reduced bioavailability of orally administered curcumin has been observed in the extremely low nanomolar serum levels even after an administration of a high dose (2% in the diet, equating to 1.2 g curcumin/kg) [13]. Therefore, it was difficult to achieve adequate plasma concentrations throughout the experimental period. Modified pharmaceutical preparations including the combination of curcumin with adjuvants (e.g., piperine) or the use of delivery particles (e.g., liposome, nanoparticle) or phospholipid formulations would be expected to be more effective [2930]. Fourth, even if curcumin is a potent AR inhibitor, the main mechanism of action by curcumin may not be associated with the Wnt/β-catenin pathway. It may be difficult to demonstrate the exact antiandrogenic mechanism for curcumin, because there are multiple, cross-talk steps of the AR signaling pathway within activated tumor cells.
In conclusion, our results demonstrate that oral curcumin intake could inhibit AR expression and probably PSA activity in part in the LNCaP xenograft model. Further studies are required to identify the antiandrogenic mechanism(s) of curcumin through which its bioavailability could be enhanced and to explore possible combination regimens for the prevention and treatment of prostate cancer.
Figures and Tables
![]() | Fig. 1The effect of curcumin on the tumor growth of LNCaP xenografts. (A) Each line represents the tumor growth over time for an individual mouse. (B) The curcumin-treated mice had a tendency to exhibit slow tumor growth during the initial treatment period. *p<0.05 compared with the tumor volume at week 7. Data are plotted as median±95% confidence interval. (C) The average tumor weights at the time of tumor harvest. Values are expressed as box and whisker plots. The solid line and cross mark inside the rectangle indicate the median and the mean of the sample distribution, respectively. (D) Established tumor growth in the LNCaP cell-derived xenograft model. |
![]() | Fig. 2Effect of curcumin on the expression of prostate-specific antigen (PSA). (A) PSA activity was determined by enzyme-linked immunosorbent assay. Values are shown as the mean±standard error of the mean for all samples in each group. (B) Calculation of PSA doubling time (DT). The solid line and cross mark inside the rectangle indicate the median and the mean of the sample distribution, respectively. The average PSA DT in the curcumin group was roughly twice that in the control group, although the difference was not statistically significant (p=0.150). |
![]() | Fig. 3Quantitative real-time polymerase chain reaction (qRT-PCR) for androgen receptor (AR), prostate-specific antigen (PSA), β-catenin, and cyclin D1. (A) The initial template quantities were plotted against the critical threshold values for the standards. A linear relationship was shown between the cycle threshold values and the corresponding cDNA levels of control plasmids. (B) Relative quantification data where the expression levels of treated samples are compared with a control sample after gene normalization. qRT-PCR results show that the mRNA expression of the AR was significantly decreased in the curcumin group. Results are shown as the mean±standard error of the mean for all samples in each group. *p<0.05 compared with the control group (p=0.011). |
![]() | Fig. 4Western blot analysis of androgen receptor (AR), β-catenin, and cyclin D1. The effect of curcumin on the expression of AR, β-catenin, and cyclin D1 proteins in the grown tumor tissues is shown. The curcumin group showed less AR expression than did the control group. |
![]() | Fig. 5Immunohistochemistry for androgen receptor (AR), prostate-specific antigen (PSA), β-catenin, and cyclin D1. (A) Tumor tissue sections were stained with antibodies against AR, PSA, β-catenin, and cyclin D1. Nuclei were counterstained (blue) with hematoxylin (×400 in each panel). (B) Positive immunohistochemical reactions are presented as the total staining intensity: no staining (0), low intensity (1), moderate intensity (2), and high intensity (3). Results are presented as the mean±standard error of the mean for all samples in each group. |
Table 1
Primers for reverse transcription polymerase chain reaction
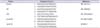
ACKNOWLEDGMENTS
We would like to acknowledge professor Dong Hoon Kim, Department of Pathology of the Hallym University, for kindly providing the pathologic preparation and interpretation. We would also like to thank to Joung Eun Lim, Samsung Biomedical Research Institute, and Hee Yoon Park, Dankook Medical Science Research Institute, for their technical support. This research was conducted with financial support provided by the OTTOGI Corporation, Anyang, Korea.
References
1. Heinlein CA, Chang C. Androgen receptor in prostate cancer. Endocr Rev. 2004; 25:276–308.
2. Richter E, Srivastava S, Dobi A. Androgen receptor and prostate cancer. Prostate Cancer Prostatic Dis. 2007; 10:114–118.
3. Yang F, Li X, Sharma M, Sasaki CY, Longo DL, Lim B, et al. Linking beta-catenin to androgen-signaling pathway. J Biol Chem. 2002; 277:11336–11344.
4. Terry S, Yang X, Chen MW, Vacherot F, Buttyan R. Multifaceted interaction between the androgen and Wnt signaling pathways and the implication for prostate cancer. J Cell Biochem. 2006; 99:402–410.
5. Yang X, Chen MW, Terry S, Vacherot F, Bemis DL, Capodice J, et al. Complex regulation of human androgen receptor expression by Wnt signaling in prostate cancer cells. Oncogene. 2006; 25:3436–3444.
6. Chesire DR, Isaacs WB. Beta-catenin signaling in prostate cancer: an early perspective. Endocr Relat Cancer. 2003; 10:537–560.
7. Yardy GW, Brewster SF. Wnt signalling and prostate cancer. Prostate Cancer Prostatic Dis. 2005; 8:119–126.
8. Verras M, Sun Z. Roles and regulation of Wnt signaling and beta-catenin in prostate cancer. Cancer Lett. 2006; 237:22–32.
9. Whitaker HC, Girling J, Warren AY, Leung H, Mills IG, Neal DE. Alterations in beta-catenin expression and localization in prostate cancer. Prostate. 2008; 68:1196–1205.
10. Kypta RM, Waxman J. Wnt/β-catenin signalling in prostate cancer. Nat Rev Urol. 2012; 9:418–428.
11. Horie S. Chemoprevention of prostate cancer: soy isoflavones and curcumin. Korean J Urol. 2012; 53:665–672.
12. Duvoix A, Blasius R, Delhalle S, Schnekenburger M, Morceau F, Henry E, et al. Chemopreventive and therapeutic effects of curcumin. Cancer Lett. 2005; 223:181–190.
13. Hatcher H, Planalp R, Cho J, Torti FM, Torti SV. Curcumin: from ancient medicine to current clinical trials. Cell Mol Life Sci. 2008; 65:1631–1652.
14. Oyagbemi AA, Saba AB, Ibraheem AO. Curcumin: from food spice to cancer prevention. Asian Pac J Cancer Prev. 2009; 10:963–967.
15. Hutchins-Wolfbrandt A, Mistry AM. Dietary turmeric potentially reduces the risk of cancer. Asian Pac J Cancer Prev. 2011; 12:3169–3173.
16. Nakamura K, Yasunaga Y, Segawa T, Ko D, Moul JW, Srivastava S, et al. Curcumin down-regulates AR gene expression and activation in prostate cancer cell lines. Int J Oncol. 2002; 21:825–830.
17. Tsui KH, Feng TH, Lin CM, Chang PL, Juang HH. Curcumin blocks the activation of androgen and interlukin-6 on prostate-specific antigen expression in human prostatic carcinoma cells. J Androl. 2008; 29:661–668.
18. Choi HY, Lim JE, Hong JH. Curcumin interrupts the interaction between the androgen receptor and Wnt/β-catenin signaling pathway in LNCaP prostate cancer cells. Prostate Cancer Prostatic Dis. 2010; 13:343–349.
19. Sato N, Gleave ME, Bruchovsky N, Rennie PS, Beraldi E, Sullivan LD. A metastatic and androgen-sensitive human prostate cancer model using intraprostatic inoculation of LNCaP cells in SCID mice. Cancer Res. 1997; 57:1584–1589.
20. Reynolds CP, Sun BC, DeClerck YA, Moats RA. Assessing growth and response to therapy in murine tumor models. Methods Mol Med. 2005; 111:335–350.
21. Kyung YS, Park HY, Lee G. Preservation of uroplakins by 2-mercaptoethanesulfonate in cyclophosphamide-induced rat cystitis. Arch Toxicol. 2011; 85:51–57.
22. Patel A, Dorey F, Franklin J, deKernion JB. Recurrence patterns after radical retropubic prostatectomy: clinical usefulness of prostate specific antigen doubling times and log slope prostate specific antigen. J Urol. 1997; 158:1441–1445.
23. Maffezzini M, Bossi A, Collette L. Implications of prostate-specific antigen doubling time as indicator of failure after surgery or radiation therapy for prostate cancer. Eur Urol. 2007; 51:605–613.
24. Bommareddy A, Eggleston W, Prelewicz S, Antal A, Witczak Z, McCune DF, et al. Chemoprevention of prostate cancer by major dietary phytochemicals. Anticancer Res. 2013; 33:4163–4174.
25. Teiten MH, Gaascht F, Cronauer M, Henry E, Dicato M, Diederich M. Anti-proliferative potential of curcumin in androgen-dependent prostate cancer cells occurs through modulation of the Wingless signaling pathway. Int J Oncol. 2011; 38:603–611.
26. Sundram V, Chauhan SC, Ebeling M, Jaggi M. Curcumin attenuates β-catenin signaling in prostate cancer cells through activation of protein kinase D1. PLoS One. 2012; 7:e35368.
27. Hollingshead MG. Antitumor efficacy testing in rodents. J Natl Cancer Inst. 2008; 100:1500–1510.
28. Perkins S, Verschoyle RD, Hill K, Parveen I, Threadgill MD, Sharma RA, et al. Chemopreventive efficacy and pharmaco-kinetics of curcumin in the min/+ mouse, a model of familial adenomatous polyposis. Cancer Epidemiol Biomarkers Prev. 2002; 11:535–540.
29. Kurita T, Makino Y. Novel curcumin oral delivery systems. Anticancer Res. 2013; 33:2807–2821.
30. Aras A, Khokhar AR, Qureshi MZ, Silva MF, Sobczak-Kupiec A, Pineda EA, et al. Targeting cancer with nano-bullets: curcumin, EGCG, resveratrol and quercetin on flying carpets. Asian Pac J Cancer Prev. 2014; 15:3865–3871.