Abstract
Purpose
Electroporation is known to enhance the efficiency of gene transfer through a transient increase in cell membrane permeability. The aim of this study was to determine the optimal conditions for in vivo electroporation-mediated gene delivery into mouse corpus cavernosum.
Materials and Methods
Diabetes was induced in C57BL/6 mice by intraperitoneal injections of streptozotocin. After intracavernous injection of pCMV-Luc (100 µg/40 µL), different electroporation settings (5-50 V, 8-16 pulses with a duration of 40-100 ms) were applied to the penis to establish the optimal conditions for electroporation. Gene expression was evaluated by luciferase assay. We also assessed the undesired consequences of electroporation by visual inspection and hematoxylin-eosin staining of penile tissue.
Results
Electroporation profoundly induced gene expression in the corpus cavernosum tissue of normal mice in a voltage-dependent manner. We observed electrical burn scars in the penis of normal mice who received electroporation with eight 40-ms pulses at a voltage of 50 V and sixteen 40-ms pulses, eight 100-ms pulses, and sixteen 100-ms pulses at a voltage of 30 V. No detectable burn scars were noted in normal mice stimulated with eight 40-ms pulses at a voltage of 30 V. Electroporation also significantly induced gene expression in diabetic mice stimulated with 40-ms pulse at a voltage of 30 V without injury to the penis.
Conclusions
We have established the optimal electroporation conditions for maximizing gene transfer into the corpus cavernosum of mice while avoiding damage to the erectile tissue. The electroporation-mediated gene delivery technique will be a valuable tool for gene therapy in the field of erectile dysfunction.
Although oral phosphodiesterase (PDE)-5 inhibitors are generally an effective and well-tolerated treatment modality for patients with erectile dysfunction (ED), PDE-5 therapy cannot cure the underlying disorder in the corpus cavernosum and has limitations such as reduced efficacy in patients with diabetes or radical prostatectomy, lack of spontaneity of the sexual act, and a contraindication in those who take nitrates [1,2,3,4,5].
Gene therapy was introduced in the field of ED in the late 1990s with various therapeutic genes. However, most of these studies remain at a preclinical level, even though the preclinical results are promising [6,7,8,9]. Despite the well-known safety of plasmid DNA as a gene delivery vector in humans [10], the extremely low transfection efficiency greatly limits its clinical application [11]. Viral vectors exert high gene transfer efficiency but have many drawbacks including systemic toxicity, induction of host immune responses, and possible integration into the host genome [12,13]. These obstacles have led to the development of new gene delivery methods, such as electroporation-, ultrasound-, and polymer-mediated gene transfer techniques [14].
Electroporation technology has emerged as a simple, nontoxic, safe, and effective method for delivering foreign genes into target cells or tissues [15,16,17]. A transient and reversible increase in cell membrane permeability with local delivery of electrical pulses to target cells by using electrodes is a major mechanism for electroporation-mediated enhanced gene delivery. In preclinical experiments, in vivo electroporation has been shown to significantly enhance gene transfer efficiency while maintaining the safety properties of plasmid DNA [18]. The phase I clinical trial of electroporation-mediated delivery of interleukin-12 plasmid with dose escalation in patients with metastatic melanoma showed the technique to be safe, effective, and reproducible [19]. A DNA vaccine against the hepatitis B virus delivered by in vivo electroporation was also shown to be effective in patients with chronic hepatitis B [20].
In the ED field, however, electroporation-mediated gene delivery is in its infancy. Electroporation has been applied to deliver adenovirus encoding neuronal nitric oxide synthase into penis in vivo [21]. Those authors used a single electroporation parameter (100 V, 8 pulses with a duration of 40 ms, and 1 second between pulses). It was reported that electroporation of skeletal muscle induces myofiber damage and a transient decrease in contractile function [22]. Because the electroporation threshold may differ between cell types and tissues, this threshold should be determined for specific cells or tissues. Parameters of the electrical pulses used for electroporation also affect the initial steps of plasmid gene translocation through the plasma membrane by regulating membrane permeability and are the key factor in determining transfection efficiency [18]. However, optimal electroporation conditions for delivering plasmid DNA into the corpus cavernosum and the adverse effects of electroporation on the erectile tissue have not yet been documented in detail.
In the present study, we determined electroporation-mediated transfection efficiency by using reporter genes in different stimulation settings in normal or diabetic mice in vivo. We used a surface-type plate platinum electrode rather than a needle-type electrode to deliver electrical pulses into the erectile tissue because the plate electrode may reduce electroporation-related pain in the clinical situation. We also examined gross and microscopic changes after electroporation.
pCMV-Luc was purchased from Promega (Madison, WI, USA) and was introduced into Escherichia coli strain JM109 (Promega) and purified by use of QIAfilter Plasmids Giga Kits (Qiagen, Valencia, CA, USA). The purity of the plasmids was certified by measuring the OD260/OD280 ratio. The concentration of plasmid DNA was determined by using 1 (OD260)=50 µg of DNA. Plasmids were stored at -20℃ until used.
Specific pathogen-free C57BL/6 mice were purchased from Orient Bio (Seongnam, Korea) and bred in our pathogen-free animal facility. Four-month-old male mice were used in this study. The experiments were approved by the Institutional Animal Care and Use Subcommittee of our university. The mice were anesthetized with ketamine (100 mg/kg) and xylazine (5 mg/kg) intramuscularly and placed on a thermoregulated surgical table. With the use of sterile technique, the penile skin was incised and the tunica albuginea was exposed. The pCMV-Luc (100 µg/40 µL) was injected into the midportion of the corpus cavernosum. Immediately after plasmid DNA injection, square wave-pulse electroporation (ECM 830, Harvard Apparatus, Holliston, MA, USA) was delivered to the injection sites by using two parallel 3-mm plate platinum electrodes (Tweezertrodes, Harvard Apparatus). To determine the optimal in vivo electroporation-mediated gene delivery condition into the corpus cavernosum, different electroporation conditions were set at 5-50 V, 8-16 pulses with a duration of 40-100 ms, and 1 second between pulses. We compressed the penis at the base with an elastic band immediately before injection, and the compression was maintained for 30 minutes after injection to minimize blood drainage via the dorsal veins. This maneuver has been shown to increase gene transfection efficiency [23]. The incision was closed with 6-0 Vicryl (polyglactin 910) sutures. At 3 days after injection, the penis was harvested and gene expression was measured by luciferase assay.
On the basis of these initial results, we also examined the efficacy of electroporation-mediated gene delivery into the penis in diabetic mice, in which diabetes was induced in 2-month-old male mice by intraperitoneal injections of streptozotocin (50 mg/kg) for 5 days consecutively as previously described [24]. At 8 weeks after the induction of diabetes, pCMV-Luc (100 µg/40 µL) was injected into the corpus cavernosum of diabetic mice. Fasting and postprandial blood glucose levels were determined with an Accu-Check blood glucose meter (Roche Diagnostic, Mannheim, Germany) before the mice were sacrificed.
For the in vivo luciferase assay, the corpus cavernosum tissues were quick-frozen in liquid nitrogen. The tissues were homogenized in 200 µL of 1× reporter lysis buffer (Promega). After incubation for 30 minutes at room temperature, the homogenates were centrifuged at 11,000 rpm for 10 minutes. The lysates were transferred to fresh tubes. The protein concentrations of the extracts were determined by using a PRO-MEASURE kit (iNtRON Biotechnology Inc., Seongnam, Korea). An amount of 100 µL of the luciferase assay reagents (Promega) was dispensed into luminometer tubes. Then 50 µL of the lysates were added to a luminometer tube containing the luciferase assay reagent, and the tubes were briefly mixed by vortexing. The luminometer was programmed to perform a 3-second measurement delay followed by a 10-second measurement read for luciferase activity. Luciferase activity was measured in terms of relative light units (RLUs) by using a luminometer (Turner BioSystems, Sunnyvale, CA, USA). The final values of luciferase were reported in terms of RLU/mg total protein.
To determine the undesired consequences of electroporation, the penis was carefully inspected. A midportion of each penile segment was harvested and immediately fixed in 10% formalin phosphate-buffered solution before paraffin embedding. The specimens were stained with hematoxylin-eosin (H&E).
Intracavernous pressure (ICP) and systemic blood pressure were measured during electrical stimulation of the cavernous nerve as previously described [24]. Bipolar platinum wire electrodes were placed around the cavernous nerve. Stimulation parameters were 5 V at a frequency of 12 Hz, a pulse width of 1 ms, and a duration of 1 minute. During tumescence, the maximal ICP was recorded. The total ICP was determined by the area under the curve from the beginning of cavernous nerve stimulation to a point 20 seconds after stimulus termination. Systemic blood pressure was measured by using a noninvasive tail-cuff system (Visitech systems, Apex, NC, USA). The ratios of maximal ICP and total ICP (area under the curve) to mean systolic blood pressure (MSBP) were calculated to adjust for variations in systemic blood pressure.
Results are expressed as means±standard deviations. The group comparisons of parametric data were made by one-way analysis of variance followed by Newman-Keuls post hoc tests. We used Mann-Whitney U tests or Kruskal-Wallis tests for nonparametric data. We performed statistical analysis with SigmaStat 3.5 software (Systat Software Inc., Richmond, CA, USA). We tested the data for normality and variance. P values less than 5% were considered significant.
Immediately after injection of pCMV-Luc (100 µg/40 µL) into the corpus cavernosum of normal mice, each animal received electroporation of the injection sites with eight 40-ms pulses at voltages of 5, 10, 30, or 50 V. Electroporation profoundly induced gene expression in the corpus cavernosum tissue of normal mice in a voltage-dependent manner. At voltages of 30 and 50 V, gene expression was significantly higher in the normal mice that received electroporation than in those treated with pCMV-Luc alone. The highest gene expression was noted in the mice stimulated at 50 V (Fig. 1).
We further determined transfection efficiency at different electroporation settings: eight 40-ms pulses, sixteen 40-ms pulses, eight 100-ms pulses, and sixteen 100-ms pulses at a voltage of 30 V. We observed significantly higher gene expression in the mice stimulated with sixteen 40-ms pulses and eight 100-ms pulses than in those stimulated with eight 40-ms pulses (Fig. 2).
Undesired consequences of electroporation were determined by gross inspection of the stimulated area and by H&E staining. We found an extensive electrical burn scar in the penis of mice that received electroporation with eight 40-ms pulses at a voltage of 50 V. Some degree of burn scarring was noted in the penis of mice stimulated with sixteen 40-ms pulses, eight 100-ms pulses, and sixteen 100-ms pulses at a voltage of 30 V. No detectable burn scarring was noted in mice stimulated with eight 40-ms pulses at a voltage of 20 or 30 V (Fig. 3). Thus, electroporation-mediated target organ damage may occur in proportion to the intensity, duration, and frequency of the electrical pulse. H&E staining revealed an area of extensive fibrosis in the corpus cavernosum of normal mice that received electroporation at 50 V (Fig. 4).
These findings suggest that eight 40-ms pulses at a voltage of 30 V is the optimal in vivo electroporation condition into the corpus cavernosum, which induces good transfection efficiency with no adverse events related to electroporation.
We also examined electroporation-mediated transfection efficiency in streptozotocin-induced diabetic mice. At 8 weeks after the induction of diabetes, pCMV-Luc (100 µg/40 µL) was injected into the corpus cavernosum of diabetic mice. The animals then received electroporation at the injection sites with eight 40-ms pulses at a voltage of 30 V. Similar to the results in normal mice, electroporation significantly increased gene expression in the corpus cavernosum of diabetic mice compared with that in mice treated with pCMV-Luc alone (Fig. 5A). Also, no observable burn scarring was noted at this electroporation setting (Fig. 5B). Fasting and postprandial blood glucose concentrations were significantly higher in the diabetic mice than in the controls (fasting glucose, 396.5±49 mg/dL vs. 106.3±13.9 mg/dL; postprandial glucose, 550.8±14.0 mg/dL vs. 136.3±5.45 mg/dL, p<0.01, respectively). The ratios of maximal ICP and total ICP to MSBP were significantly lower in the diabetic mice than in the controls (maximal ICP/MSBP, 0.29±0.03 vs. 0.56±0.01; total ICP/MSBP, 14.4±1.5 vs. 31.2±0.8; p<0.01, respectively).
The main observation of the present study was that
in vivo electroporation profoundly enhanced the gene transfer efficiency of plasmid DNA in the corpus cavernosum in proportion to the intensity of the electrical pulse. However, we also observed damage to the erectile tissue as a result of the electroporation: the higher the intensity, duration, and frequency of the electrical pulse, the higher the possibility of target organ damage.
Some authors have shown that short-duration high-voltage pulses result in efficient DNA transfer [25], whereas others have suggested that longer intermediate-voltage pulses result in more efficient gene transfer [26]. The results of our study make clear that both voltage and duration of the electrical pulse affect the transfection efficiency. However, we must consider the tissue damage produced by electroporation in experimental settings when we apply this technique. Although the highest gene expression was noted at a voltage of 50 V with eight 40-ms pulses, we also observed critical burn scarring and necrosis of the penis at this stimulation parameter. Furthermore, an increase in frequency or duration of the electrical pulse also induced damage to the penis even at the same voltage (30 V). Specifically, we observed some degree of burn scarring in the penis of mice stimulated at sixteen 40-ms or eight 100-ms pulses but not at eight 40-ms pulses. In a phase I trial of electroporation-mediated delivery of interleukin-12 plasmid in patients with metastatic melanoma, there were no serious adverse events related to electroporation [19]. Most patients experienced pain during electroporation but it was transient and modest. The authors reported that topical application of 1% lidocaine was effective for minimizing pain [19].
Besides the parameters of the electrical pulses used for electroporation, it is also important to determine which type of electrode is ideal for electroporation-mediated gene delivery into the corpus cavernosum. In the present study, we applied a surface-type plate platinum electrode to penile skin to deliver electrical pulses into the underlying cavernous tissue. Further studies are needed to test whether direct application of electrical pulses into the corpus cavernosum by use of a needle-type electrode would result in different transfection efficiency or a different safety profile.
Functional and structural derangements in cavernous vasculature are one of the most important mechanisms involved in the pathogenesis of ED from vascular risk factors [27]. Recently, much attention has focused on therapeutic angiogenesis to recover cavernous angiopathy and to restore erectile function by using a variety of proangiogenic genes at a preclinical level [27]. A previous study reported that in vivo electroporation-mediated delivery of hypoxia-inducible factor-1α plasmid DNA significantly improves perfusion through increased capillary density and collateral vessel formation in the hind limb of mice compared with mice that received plasmid DNA alone [28]. Electroporation-mediated gene delivery of proangiogenic factors, such as fibroblast growth factor-2, vascular endothelial growth factor, and hepatocyte growth factor, have also demonstrated efficacy in a mouse model of hind limb ischemia [29,30], which further reinforces the usefulness of electroporation in gene delivery for therapeutic angiogenesis. Therefore, it is worthwhile to apply the electroporation technique in the field of ED with a target of therapeutic angiogenesis. Further studies are necessary to determine whether electroporation-mediated delivery of therapeutic plasmid genes can achieve better clinical outcomes than does conventional plasmid or virus-mediated gene therapy. A lack of study showing the long-term time course of gene transfection efficiency or electroporation-mediated adverse events with the use of a therapeutic gene is a limitation of this study.
With regard to determining specific aspects of an electroporation protocol, including transfection efficiency and adverse tissue damage, our study may provide important practical information for electroporation-mediated gene therapy in the field of ED. Electroporation-mediated gene therapy lacks the efficacy concerns or biohazard considerations implicit in plasmid or viral vectors. Moreover, our protocol will be useful in future refinement of electroporation-mediated gene delivery protocols for the corpus cavernosum.
We have established the optimal electroporation conditions for maximizing gene transfer into the corpus cavernosum of mice while avoiding damage to the erectile tissue. Our results suggest that electroporation with eight 40-ms pulses at a voltage of 30 V is not injurious to the penis and is an optimal in vivo electroporation parameter in the corpus cavernosum of mice. The electroporation-mediated gene delivery technique will be a valuable tool for gene therapy in the field of ED.
Figures and Tables
Fig. 1
Luciferase expression driven by pCMV-Luc with electroporation (EP) in the corpus cavernosum of normal mice. pCMV-Luc (100 µg/40 µL) was injected into the midportion of the corpus cavernosum and then each animal received EP at the injection sites with eight 40-ms pulses at voltages of 5, 10, 30, or 50 V. The penis was harvested and gene expression was measured by luciferase assay 3 days after injection. The luciferase activity of the no EP group was arbitrarily set equivalent to 1. The data are expressed as mean values (±standard deviation) for 5 animals per group. **p<0.01 vs. no EP group. RLU, relative light unit.
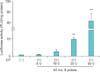
Fig. 2
Luciferase expression driven by pCMV-Luc with electroporation (EP) in the corpus cavernosum of normal mice. pCMV-Luc (100 µg/40 µL) was injected into the midportion of the corpus cavernosum and then each animal received EP at the injection sites with the following stimulation settings: eight 40-ms pulses, sixteen 40-ms pulses, eight 100-ms pulses, and sixteen 100-ms pulses at a voltage of 30 V. The penis was harvested and gene expression was measured by luciferase assay 3 days after injection. The luciferase activity of the eight 40-ms pulses group was arbitrarily set equivalent to 1. The data are expressed as mean values (±standard deviation) for 5 animals per group. **p<0.01 vs. eight 40-ms pulses group; *p<0.05 vs. eight 40-ms pulses group. RLU, relative light unit.
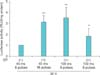
Fig. 3
The gross appearance of penis of normal mice after electroporation (EP). pCMV-Luc (100 µg/40 µL) was injected into the midportion of the corpus cavernosum and then each animal received EP into injection sites with following stimulation settings: no EP (A); eight 40-ms pulses at a voltage of 20 (B); eight 40-ms pulses (D), sixteen 40-ms pulses (E), eight 100-ms pulses (F), and sixteen 100-ms pulses at a voltage of 30 (G); and eight 40-ms pulses at a voltage of 50 (C). Note area of electrical burn scar in the penis (arrows).
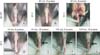
Fig. 4
Hematoxylin-eosin (H&E) staining of penis of normal mice after electroporation (EP). pCMV-Luc (100 µg/40 µL) was injected into the midportion of the corpus cavernosum and then each animal received EP into injection sites with following stimulation settings: no EP (A); eight 40-ms pulses at voltages of 30 (B); and eight 40-ms pulses at voltages of 50 (C). Note area of extensive necrosis in the corpus cavernosum of mice that received EP at 50 V (arrow).
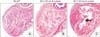
Fig. 5
Luciferase expression driven by pCMV-Luc with electroporation (EP) in the corpus cavernosum of streptozotocin-induced diabetic mice. At 8 weeks after the induction of diabetes, pCMV-Luc (100 µg/40 µL) was injected into the midportion of the corpus cavernosum of diabetic mice or their age-matched controls. The animals then each received EP at the injection sites with eight 40-ms pulses at a voltage of 30 V. (A) The penis was harvested and gene expression was measured by luciferase assay 3 days after injection. The luciferase activity of the normal mice without EP was arbitrary set equivalent to 1. The data are expressed as mean values (±standard deviation) for 5 animals per group. **p<0.01 vs. no EP group. (B) The gross appearance of the penis of diabetic mice after EP. RLU, relative light unit; DM, diabetes mellitus.
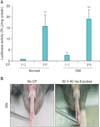
ACKNOWLEDGMENTS
This study was supported by a grant of the Korea Healthcare technology R&D Project, Ministry for Health, Welfare & Family Affairs, Republic of Korea (Jun-Kyu Suh and Ji-Kan Ryu, A110076).
References
1. Goldstein I, Lue TF, Padma-Nathan H, Rosen RC, Steers WD, Wicker PA. Sildenafil Study Group. Oral sildenafil in the treatment of erectile dysfunction. N Engl J Med. 1998; 338:1397–1404.
2. Rendell MS, Rajfer J, Wicker PA, Smith MD. Sildenafil Diabetes Study Group. Sildenafil for treatment of erectile dysfunction in men with diabetes: a randomized controlled trial. JAMA. 1999; 281:421–426.
3. Choi HK, Kim JJ, Kim SC, Suh J, Park YK, Choi S, et al. A randomised, double-blind, parallel, placebo-controlled study of the efficacy and safety of tadalafil administered on-demand to men with erectile dysfunction in Korea. Korean J Urol. 2006; 47:852–858.
4. Martinez-Jabaloyas JM, Gil-Salom M, Villamon-Fort R, Pastor-Hernandez F, Martinez-Garcia R, Garcia-Sisamon F. Prognostic factors for response to sildenafil in patients with erectile dysfunction. Eur Urol. 2001; 40:641–646.
5. Gresser U, Gleiter CH. Erectile dysfunction: comparison of efficacy and side effects of the PDE-5 inhibitors sildenafil, vardenafil and tadalafil: review of the literature. Eur J Med Res. 2002; 7:435–446.
6. Garban H, Marquez D, Magee T, Moody J, Rajavashisth T, Rodriguez JA, et al. Cloning of rat and human inducible penile nitric oxide synthase. Application for gene therapy of erectile dysfunction. Biol Reprod. 1997; 56:954–963.
7. Christ GJ, Rehman J, Day N, Salkoff L, Valcic M, Melman A, et al. Intracorporal injection of hSlo cDNA in rats produces physiologically relevant alterations in penile function. Am J Physiol. 1998; 275(2 Pt 2):H600–H608.
8. Champion HC, Bivalacqua TJ, Hyman AL, Ignarro LJ, Hellstrom WJ, Kadowitz PJ. Gene transfer of endothelial nitric oxide synthase to the penis augments erectile responses in the aged rat. Proc Natl Acad Sci U S A. 1999; 96:11648–11652.
9. Harraz A, Shindel AW, Lue TF. Emerging gene and stem cell therapies for the treatment of erectile dysfunction. Nat Rev Urol. 2010; 7:143–152.
10. Henry TD, Hirsch AT, Goldman J, Wang YL, Lips DL, McMillan WD, et al. Safety of a non-viral plasmid-encoding dual isoforms of hepatocyte growth factor in critical limb ischemia patients: a phase I study. Gene Ther. 2011; 18:788–794.
11. Ferraro B, Morrow MP, Hutnick NA, Shin TH, Lucke CE, Weiner DB. Clinical applications of DNA vaccines: current progress. Clin Infect Dis. 2011; 53:296–302.
12. Young LS, Searle PF, Onion D, Mautner V. Viral gene therapy strategies: from basic science to clinical application. J Pathol. 2006; 208:299–318.
13. Campos SK, Barry MA. Current advances and future challenges in Adenoviral vector biology and targeting. Curr Gene Ther. 2007; 7:189–204.
14. Wells DJ. Electroporation and ultrasound enhanced non-viral gene delivery in vitro and in vivo. Cell Biol Toxicol. 2010; 26:21–28.
15. Andreason GL, Evans GA. Introduction and expression of DNA molecules in eukaryotic cells by electroporation. Biotechniques. 1988; 6:650–660.
16. Somiari S, Glasspool-Malone J, Drabick JJ, Gilbert RA, Heller R, Jaroszeski MJ, et al. Theory and in vivo application of electroporative gene delivery. Mol Ther. 2000; 2:178–187.
17. Mir LM, Moller PH, Andre F, Gehl J. Electric pulse-mediated gene delivery to various animal tissues. Adv Genet. 2005; 54:83–114.
18. Satkauskas S, Ruzgys P, Venslauskas MS. Towards the mechanisms for efficient gene transfer into cells and tissues by means of cell electroporation. Expert Opin Biol Ther. 2012; 12:275–286.
19. Daud AI, DeConti RC, Andrews S, Urbas P, Riker AI, Sondak VK, et al. Phase I trial of interleukin-12 plasmid electroporation in patients with metastatic melanoma. J Clin Oncol. 2008; 26:5896–5903.
20. Yang FQ, Yu YY, Wang GQ, Chen J, Li JH, Li YQ, et al. A pilot randomized controlled trial of dual-plasmid HBV DNA vaccine mediated by in vivo electroporation in chronic hepatitis B patients under lamivudine chemotherapy. J Viral Hepat. 2012; 19:581–593.
21. Magee TR, Ferrini M, Garban HJ, Vernet D, Mitani K, Rajfer J, et al. Gene therapy of erectile dysfunction in the rat with penile neuronal nitric oxide synthase. Biol Reprod. 2002; 67:20–28.
22. Roche JA, Ford-Speelman DL, Ru LW, Densmore AL, Roche R, Reed PW, et al. Physiological and histological changes in skeletal muscle following in vivo gene transfer by electroporation. Am J Physiol Cell Physiol. 2011; 301:C1239–C1250.
23. Lee M, Ryu JK, Oh SM, Lee E, Shin HY, Song SU, et al. Water-soluble lipopolymer as a gene carrier to corpus cavernosum. Int J Impot Res. 2005; 17:326–334.
24. Jin HR, Kim WJ, Song JS, Choi MJ, Piao S, Shin SH, et al. Functional and morphologic characterizations of the diabetic mouse corpus cavernosum: comparison of a multiple low-dose and a single high-dose streptozotocin protocols. J Sex Med. 2009; 6:3289–3304.
25. Vicat JM, Boisseau S, Jourdes P, Laine M, Wion D, Bouali-Benazzouz R, et al. Muscle transfection by electroporation with high-voltage and short-pulse currents provides high-level and long-lasting gene expression. Hum Gene Ther. 2000; 11:909–916.
26. Mir LM, Bureau MF, Gehl J, Rangara R, Rouy D, Caillaud JM, et al. High-efficiency gene transfer into skeletal muscle mediated by electric pulses. Proc Natl Acad Sci U S A. 1999; 96:4262–4267.
27. Ryu JK, Suh JK. Therapeutic angiogenesis as a potential future treatment strategy for erectile dysfunction. World J Mens Health. 2012; 30:93–98.
28. Ouma GO, Rodriguez E, Muthumani K, Weiner DB, Wilensky RL, Mohler ER 3rd. In vivo electroporation of constitutively expressed HIF-1α plasmid DNA improves neovascularization in a mouse model of limb ischemia. J Vasc Surg. 2014; 59:786–793.
29. Ferraro B, Cruz YL, Baldwin M, Coppola D, Heller R. Increased perfusion and angiogenesis in a hindlimb ischemia model with plasmid FGF-2 delivered by noninvasive electroporation. Gene Ther. 2010; 17:763–769.
30. Makarevich P, Tsokolaeva Z, Shevelev A, Rybalkin I, Shevchenko E, Beloglazova I, et al. Combined transfer of human VEGF165 and HGF genes renders potent angiogenic effect in ischemic skeletal muscle. PLoS One. 2012; 7:e38776.