Abstract
Purpose
To evaluate tumor-specific immunity and define the mechanisms involved in the cryoimmunologic response, we compared the tumor control efficacy and immunologic responses of cryoablation with those of surgical excision in a tumor rechallenge model.
Materials and Methods
Sixty BALB/c mice with RENCA tumors that were generated in the left flank area underwent cryoablation or radical excision. The mice successfully treated were rechallenged with RENCA or an undifferentiated colon carcinoma cell line, CT26, in the contralateral right flank area. The recurrence rate after tumor rechallenge in each group was then observed. To assess the immunologic response of each treatment modality, fluorescent-activated cell sorting (FACS) analysis and a cytotoxicity assay using 51Cr release were performed.
Results
After reinoculation of the RENCA cells, the rate of tumor growth was significantly higher in the surgical excision group than in the cryoablation group (94.4% vs. 11.1%, p=0.001). In the cryoablation group, the tumor growth rate was significantly increased after rechallenge of CT26 cells compared with RENCA (94.1% vs. 11.1%, p=0.001). The cryoablation group showed an elevated CD3, CD4, CD8 T, and natural killer cell count in the FACS analysis and also showed significantly increased cytotoxicity in the 51Cr release assay compared with the excision group.
Historical evidence demonstrates that renal cell carcinoma (RCC) is immunogenic; this knowledge has stimulated intensive efforts to harness the immune system to improve the outcomes of patients with RCC, especially for those with advanced disease. RCC has been shown to express a variety of tumor-associated antigens that contribute to its immunogenicity [1]. Moreover, spontaneous regression, mainly of pulmonary lesions, has been observed in patients with metastatic RCC [2]. Despite the debate about the actual percentage of response, consensus exists that spontaneous regression does occur and that immune mechanisms are thought to play an important role in these events [3].
Among the surgical treatment options for RCC, cryoablation is receiving attention owing to the advantages of its minimal invasiveness and nephron sparing. Furthermore, different from other ablation techniques using hyperthermia, which cause melting and fusing of cell membranes and protein denaturation, cryoablation leaves tumor proteins and tumor-associated antigens relatively intact. The residual tumor antigens, in the inflammatory microenvironment, could stimulate antitumor immune responses [4,5].
If cryoablation of RCC can induce antitumor immune responses capable of reducing both local and distant recurrences, then this approach may be superior not only to other ablative technologies but also to surgical excisions. Therefore, to examine the possibilities of the cryoimmunologic response, we compared the immunological responses of cryoablation with those of surgical excision in a murine model of RCC, which is known as a highly immunogenic tumor.
Sixty BALB/c mice aged 6 to 8 weeks were used. They were acclimated for at least 1 week. The animal quarters were strictly maintained at 22℃±2℃ with 50% relative humidity and followed a 12-hour light-dark cycle. All experiments in the present study were carried out in a pathogen-free animal laboratory at the Korea University School of Life Sciences and Biotechnology, Seoul, Korea, and were performed in accordance with the National Institutes of Health Guidelines for the Care and Use of Laboratory Animals.
RENCA is a murine RCC of spontaneous origin in the BALB/c mouse. CT26 is an N-nitroso-N-methylurethane-induced undifferentiated colon carcinoma cell line from the BALB/c mouse. The tumors were generated in the left flank area in vivo by subcutaneous injection of all 60 BALB/c mice with 1×106 viable RENCA cells. On day 7 after RENCA inoculation in the left flank area, the tumors were selected for either cryoablation or surgical excision when the diameter reached about 6 mm.
A total of 40 mice underwent cryosurgery and 20 mice underwent surgical excision. The mice were placed in sanitized laminar flow hoods and anesthetized with ketamine by inhalation. They were then placed in a prone position and the tumor site was prepared with alcohol. Surgical excision of the tumors was performed widely to obtain grossly negative surgical margins. Cryoablation was performed by using liquid nitrogen spray equipment (Cryogun, Sanarus Technologies Inc., Pleasanton, CA, USA) designed to create temperatures below -40℃ to effectively kill malignant cells. Anesthetized mice were placed in a prone position on a heating pad to avoid hypothermia, and the tumor site was prepared with alcohol. The overlying skin was divided and retracted away from the tumor. The freezing nozzle of the spray was positioned 1 to 1.5 cm from the tumor mass and aimed at the center of the mass. Spraying of 10 seconds accomplished freezing of about 1 mm of normal tissue surrounding the tumor mass. The mass was then allowed to thaw completely. Such freeze-thaw cycles were repeated to achieve effective tumor killing. The skin was then closed over the ablated tumor by use of interrupted sutures. All mice were placed under a warming lamp during the recovery period.
At 14 days after the cryoablation or surgical excision, a secondary tumor rechallenge was performed in the right flank area in mice that were free of tumor recurrence at the previously treated left flank area (Fig. 1). For the surgical excision group, the same dose of RENCA cells (1×106) was injected subcutaneously to the right flank area. The mice treated by cryoablation were divided into two groups: half of them received 1×106 RENCA cells as a rechallenge, subcutaneously in the right flank area, and the other half received the same amount of CT26 cells for rechallenge in the right flank area. The growth patterns of the tumors were carefully evaluated for 28 days. Fluorescent-activated cell sorting (FACS) analysis and cytotoxicity assay were performed and compared between the RENCA rechallenge group after either excision or cryoablation (Fig. 1).
Blood sampling from the RENCA rechallenge groups was performed before the mice were sacrificed on the 28th day after RENCA rechallenge. The blood was suspended in tubes containing 25 µL of heparin (5,000 IU/mL). The erythrocytes were lysed in a buffer containing 0.84% NH4Cl at pH 7.4 for 3 to 5 minutes. The remaining cells were then washed twice in saline. After washing in saline, the cells were resuspended and fixed in 500 µL saline. The blood lymphocyte suspension was screened for CD3, CD4, CD8, CD19, and natural killer (NK) cells. Each 0.1-mL sample of peripheral lymphocytes was incubated for 30 minutes at 4℃ in the dark, with a solution consisting of 6 µL 0.01 mol/L phosphate buffered saline as well as the appropriate concentration of antibodies. After washing in saline, the cells were resuspended and fixed in 500-µL saline. The cells were then analyzed on a FACS system (Beckman Coulter Inc., Brea, CA, USA).
To evaluate the cytotoxic activity of the lymphocytes from the cryoablation group and the surgical excision group, a 51Cr release assay was performed as an additional experiment. We prepared another 15 BALB/c mice (5 for each of three groups: cryoablation group, surgical excision group, and tumor control group) of the same age for the 51Cr release assay. The mice with RECNA tumors were treated by either cryoablation or surgical excision on the 7th day after RENCA inoculation and then the groups were sacrificed on the 14th day after the operation. The tumor control group that had not undergone RENCA inoculation was sacrificed on the same day as the other groups. Spleens were harvested for a cytotoxicity assay against the RENCA cell line. Briefly, RENCA cells were plated as target cells at a density of 5,000 cells/well in 96-well plates. The cells were incubated with 1 µCi/well of Na2 51CrO4 for 24 hours. Mouse lymphocytes as effector cells were obtained from the spleen in both groups. The lymphocytes were then cultured with RENCA cells, which were irradiated with 50 Gy in order to serve as stimulator cells. The target cells were added to various numbers of effecter cells in 96-well plates. After 4 hours of incubation at 37℃, the supernatants were collected and the radioactivity released during incubation from lysed target cells was measured. The mean percentage of specific lysis in triplicate wells was calculated by using the following formula: cytotoxicity (%)=[(experimental release-spontaneous release)/(maximal release-spontaneous release)]×100%.
We performed a preliminary experiment separate from the above main study on 10 mice to confirm the local efficacy of the cryoablation by using the liquid nitrogen spray equipment. On the 7th day after the injection of 1×106 RENCA cells in the flank area, the tumors of about 6 mm in diameter underwent cryoablation in the same manner as in the main study. Pathological examination of the cryoablated site was performed in all 10 mice 14 days after the cryoablation. The tumor and the surrounding tissue were removed in an en block manner. The resected tissue was fixed in formalin, and histological examination under H&E stain was performed.
For the statistical analysis, the local tumor growth rate after tumor rechallenge was analyzed by using chi-square tests (Table 1). The results of the FACS analysis were analyzed by using the Mann-Whitney U test (Fig. 2A). The cytotoxic activity of lymphocytes among the cryoablation, surgical excision, and tumor control groups was compared by using the Kruskal-Wallis and Mann-Whitney U tests (Fig. 2B). All statistical analyses were processed by using SPSS ver. 17.0 (SPSS Inc., Chicago, IL, USA) and p-values below 0.05 were considered significant.
The local recurrence rate at the left flank area was 12.5% (5/40) at 14 days after cryoablation. This result was comparable to the recurrence rate of 10% (2/20) after surgical excision. After reinoculation with RENCA cells, the rate of tumor growth in the surgical excision group was significantly higher than in the cryoablation group (94.4% vs. 11.1%, p=0.001). In the cryoablation group, the tumor growth rate was significantly increased after rechallenge with CT26 cells compared with RENCA cells (94.1% vs. 11.1%, p=0.001) (Table 1).
The FACS analysis showed that the serum level of CD3 was higher in the cryoablation group than in the excision group. The CD4 and CD8 counts, indicators of helper T cell and cytotoxic T-cell activity, respectively, were also higher in the cryoablation group than in the excision group. NK-cell activity was low in both groups compared with the activity of other cytokines, but was higher in the cryoablation group than the activity in the excision group. Different from the other markers, the B-cell marker CD19 count was relatively higher in the excision group than in the cryoablation group, but this difference was not statistically significant (Fig. 2A).
The mice treated by cryosurgery demonstrated increased cytotoxicity at several ratios of effecter to target cells (E:T ratio) compared with the ratios in the mice treated by excision or in the tumor control group. The significantly increased cytotoxicity of the cryoablation group, compared with the surgical excision or tumor control group, was manifested at an E:T of 40:1 and maintained to 5:1. The cytotoxicity of the surgical excision group was also statistically increased compared with the control group from an E:T of 40:1 to 5:1 (Fig. 2B: *p<0.05 by Kruskal-Wallis test and Mann-Whitney U test for the cryoablation group compared with the surgical excision group or control group; †p<0.05 by Mann-Whitney U test for the excision group compared with the control group).
After 14 days of cryoablation with the liquid nitrogen spray, the 10 pathologic specimens showed central coagulative necrotic areas that were characterized by ghost cells with no apparent nuclei and infiltration of polymorphic neutrophils. There were no signs of viable tumor cells (Fig. 3).
Cryosurgery is currently the best documented and studied ablative procedure for kidney cancer [6]. Cryosurgery causes direct cellular damage by ice crystals, intracellular dehydration, and delayed tissue necrosis by injury to the local microvasculature. Not only that, it might induce apoptosis at the periphery of the ice ball [7,8]. Another theory of the mechanism of freezing injury is the cryoimmunologic response [8,9]. The majority of the evidence for cryoimmunologic responses comes from reports of distant disease resolving after ablation of a primary tumor [10,11]. Investigations with several animal models have suggested a positive immunological response to tumor tissue after cryosurgery. Neel 3rd and Ritts Jr [12] cultivated chemically induced mammary sarcomas in mice and compared four different treatments of the primary tumor, including cryosurgery, and the effect of the different treatments on a secondary tumor injected at a remote site. Of the four different treatment modalities, cryosurgery showed the lowest incidence and smallest size of tumor growth after the secondary challenge. Lubaroff et al. [13] reported similar results in Copenhagen rats by using the syngeneic Dunning R3327 tumor cell line. They found that the secondary tumor challenges were rejected. Recently, Sabel et al. [14] reported that cryoablation resulted in the induction of a tumor-specific T-cell response in the tumor-draining lymph nodes as well as increased systemic NK-cell activity, which correlated with the rejection of tumors on rechallenge in a breast cancer animal model. In our experiments, the results showed that 94.4% of mice treated by surgical excision had grown tumors, whereas only 11.1% of mice treated by cryosurgery showed tumor growth after tumor rechallenge (p=0.001). In the trial using the cell line, the CT26 cells, the tumor growth rate was 94.1%; this rate was similar to that of the surgical excision group. These results not only confirmed the immunological response to cryosurgery, compared with surgical excision, but also showed that the response was tumor-specific, because the response to the secondary non-RENCA cell line, CT26, was very different.
Research in cryoimmunology has elucidated two specific areas of the immune system that could be stimulated with cryosurgery. One is the production of antitumor antibodies [15]. A second potential method by which the immune system influences tumor growth is by cytotoxic T lymphocyte (CTL)-mediated tumor cell killing [16]. The mechanism of CTL action has been shown to be the major form of tumor destruction in a normal functioning immune system [17]. Yet it has not received much attention in cryoimmunology research. Eskandari et al. [16] examined T-cell activation after cryoablation of the syngeneic R3327 tumor in Copenhagen rats. The T-cell activity was increased 2 weeks after cryoablation compared with the preoperative levels. Similarly, Sabel et al. [14] described that tumor-specific T-cell responses were evident after cryoablation in lymphocytes from tumor-draining lymph nodes. In their experiment, the NK-cell activity was also increased in the cryoablation group compared with the NK-cell activity in the surgery group (24.5% vs. 16.5%). Several subsets of lymphocytes belonging to both innate and acquired branches of the immune system have cytotoxic capabilities. Among them, NK cells are considered the major cytotoxic effector cells of innate immunity, whereas thymic-derived CD8-positive CTL is implicated in acquired responses. With regard to the increased number and activity of CTLs, these results are consistent with our findings in which the cryosurgery group showed an elevated CD3, CD4, CD8 T, and NK-cell count compared with the levels in the excision group in the FACS analysis. This finding is consistent with the current concept that in antitumor immunity, cell-mediated cytotoxicity plays a crucial role in the elimination of tumor cells, and that the killing of the tumor cells by CD8-positive CTLs plays a central role [18].
Although the increase in the number of T and NK cells observed in blood may suggest a heightened T- and NK-cell activity in our experiments, it is unclear whether the T and NK cells were activated against the RENCA cells. This is because the assay used to measure the cell count was not a functional assay. The cell count can increase nonspecifically by procedures such as excision as well as cryosurgery. Therefore, we performed a 51Cr release assay that assessed the cell-mediated cytotoxicity more specifically. The results of the 51Cr release assay showed that the CTLs from the cryosurgery group actually had higher cytotoxic activity against the RENCA cells than did the CTLs in the surgical excision group. The cytotoxicity assay proved that the increased number of CTLs induced by cryoablation was specifically activated against RENCA cells.
Precise positioning of the cryoprobe is critical, and the procedure should be monitored by means of thermocouples or ultrasound to confirm extension of the ice ball beyond the margins of the renal tumor for reliable tumor destruction in a real clinical setting [19,20]. However, our study used a liquid spray cryogun instead of cryoprobes. We wanted to ensure that the cryogun could induce definite tumor death, because remnant viable tumors would affect the results of our experiment. Through the preliminary study, we confirmed by pathologic examination that there were no signs of viable tumor cells 14 days after cryosurgery with a spray cryogun.
The limitations of our study include that our cryosurgery group did not include non-tumor-bearing mice as a control group. However, Matin et al. [21] reported previously that immunologic changes occur in both tumor-bearing kidneys and non-tumor-bearing kidneys after cryoablation. Drawing from this result, despite the absence of a non-tumor-bearing control group in our study, we assume that the cryoablation may provide an initial in situ immune reaction in a non-tumor-bearing control group. In addition, compared to the study of Matin et al. [21], our study not only confirmed the immunological response to cryosurgery compared with surgical excision, but also showed that the response was tumor-specific by use of the secondary non-RENCA cell line, CT26. Our results from an animal model with a small size tumor cannot promise that the same cryoimmunologic response will occur in patients with larger localized RCC or in patients with metastatic RCC. However, Osada et al. [22] identified that tumor necrosis and reduction in size were shown for not only the treated tumor but also the untreated tumor in 2 of 5 patients with unresectable liver tumors after cryosurgery. Several animal model studies have demonstrated that the immune response initiated by cryoablation can be amplified to protect from tumor rechallenge by other adjunctive treatment such as injecting Bacillus Calmette-Guerin into the lesion [23]. Further research on the optimum method for increasing the cryoimmunologic response to improve the clinical outcomes of patients with RCC is needed.
In this murine RCC model, cryosurgery resulted in the induction of a tumor-specific immune response, which correlated with rejection of tumors upon rechallenge, and it was proven to have superior results compared with excision. Such results imply that there is a possibility of improving tumor control with lowered recurrence and metastasis rates in RCC by inducing a cryoimmunologic response. However, more research is mandatory to determine the definite role of the immunologic response to cryosurgery.
Figures and Tables
FIG. 1
Flowchart of tumor rechallenges. After 14 days of each treatment including surgical excision and cryoablation on the left side of the tumors induced by RENCA injection, the RENCA cells were reinjected on the right side of the BALB/c mice, which was free of tumor recurrence. To verify tumor specific immunity, for 20 mice treated with cryoablation and free of tumor recurrence, CT26 cells were injected in a similar manner. FACS, fluorescent-activated cell sorting.
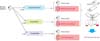
FIG. 2
Result of the fluorescent-activated cell sorting (FACS) analysis of the cryoablation and surgical excision. (A) The CD3, CD4, CD8, and natural killer cell counts measured by the FACS assay were significantly increased in the cryoablation group compared to the surgical excision group. The CD19 cell count was higher in the surgical excision group, but this difference was not statistically significant. *p<0.05, by Mann-Whitney U test. (B) The cytotoxic activity of lymphocyte after cryoablation. Mice with RECNA tumors treated by either cryoablation or surgical excision were sacrificed and spleens were harvested for a cytotoxicity assay against RENCA cell line. The significantly increased cytotoxicity of cryoablation group, compared with surgical excision or tumor control group, was manifested at E:T ratio of 40:1, and maintained to 5:1. The cytotoxicity of surgical excision was statistically increased also compared to control group, from E:T ratio of 40:1 to 10:1 (*p<0.05, by Kruskal-Wallis test and Mann-Whitney U test compared cryoablation group to surgical excision group or control group. †p<0.05, by Mann-Whitney U test compared excision group to control group). E:T ratio, ratios of effecter to target cells.

ACKNOWLEDGMENTS
This study was supported by a research grant from the Korea University College of Medicine (Seoul, Korea).
References
1. deKernion JB. Treatment of advanced renal cell carcinoma: traditional methods and innovative approaches. J Urol. 1983; 130:2–7.
2. Rabinovitch RA, Zelefsky MJ, Gaynor JJ, Fuks Z. Patterns of failure following surgical resection of renal cell carcinoma: implications for adjuvant local and systemic therapy. J Clin Oncol. 1994; 12:206–212.
3. Skinner DG, Colvin RB, Vermillion CD, Pfister RC, Leadbetter WF. Diagnosis and management of renal cell carcinoma. A clinical and pathologic study of 309 cases. Cancer. 1971; 28:1165–1177.
4. Oosterwijk E, Divgi CR, Brouwers A, Boerman OC, Larson SM, Mulders P, et al. Monoclonal antibody-based therapy for renal cell carcinoma. Urol Clin North Am. 2003; 30:623–631.
5. Jacobsohn KM, Wood CG. Adjuvant therapy for renal cell carcinoma. Semin Oncol. 2006; 33:576–582.
6. Mabjeesh NJ, Avidor Y, Matzkin H. Emerging nephron sparing treatments for kidney tumors: a continuum of modalities from energy ablation to laparoscopic partial nephrectomy. J Urol. 2004; 171(2 Pt 1):553–560.
7. Hoffmann NE, Bischof JC. The cryobiology of cryosurgical injury. Urology. 2002; 60:2 Suppl 1. 40–49.
8. Sidana A, Rodriguez R. Urologic applications of cryo-immunology. Korean J Urol. 2009; 50:629–634.
9. Johnson JP. Immunologic aspects of cryosurgery: potential modulation of immune recognition and effector cell maturation. Clin Dermatol. 1990; 8:39–47.
10. Gage AA. Cryosurgery for oral and pharyngeal carcinoma. Am J Surg. 1969; 118:669–672.
11. Soanes WA, Ablin RJ, Gonder MJ. Remission of metastatic lesions following cryosurgery in prostatic cancer: immunologic considerations. J Urol. 1970; 104:154–159.
12. Neel HB 3rd, Ritts RE Jr. Immunotherapeutic effect of tumor necrosis after cryosurgery, electrocoagulation, and ligation. J Surg Oncol. 1979; 11:45–52.
13. Lubaroff DM, Reynolds CW, Canfield L, McElligott D, Feldbush T. Immunologic aspects of the prostate. Prostate. 1981; 2:233–248.
14. Sabel MS, Nehs MA, Su G, Lowler KP, Ferrara JL, Chang AE. Immunologic response to cryoablation of breast cancer. Breast Cancer Res Treat. 2005; 90:97–104.
15. Ablin RJ, Soanes WA, Gonder MJ. Elution of in vivo bound antiprostatic epithelial antibodies following multiple cryotherapy of carcinoma of prostate. Urology. 1973; 2:276–279.
16. Eskandari H, Ablin RJ, Bhatti RA. Immunologic responsiveness & tumour growth of the Dunning R3327 rat prostatic adenocarcinoma following cryosurgery & orchiectomy. Indian J Exp Biol. 1982; 20:872–874.
17. Playfair JH, Chain BM. Immunity to tumours. In : Playfair JH, Chain BM, editors. Immunology at a glance. 8th ed. Malden: Blackwell;2005. p. 70–71.
18. Abbas AK, Lichtman AH. Cellular and molecular immunology. 5th ed. Singapore: Elsevier;2004.
19. Aron M, Gill IS. Minimally invasive nephron-sparing surgery (MINSS) for renal tumours. Part II: probe ablative therapy. Eur Urol. 2007; 51:348–357.
20. Onik GM, Reyes G, Cohen JK, Porterfield B. Ultrasound characteristics of renal cryosurgery. Urology. 1993; 42:212–215.
21. Matin SF, Sharma P, Gill IS, Tannenbaum C, Hobart MG, Novick AC, et al. Immunological response to renal cryoablation in an in vivo orthotopic renal cell carcinoma murine model. J Urol. 2010; 183:333–338.
22. Osada S, Imai H, Tomita H, Tokuyama Y, Okumura N, Matsuhashi N, et al. Serum cytokine levels in response to hepatic cryoablation. J Surg Oncol. 2007; 95:491–498.
23. Udagawa M, Kudo-Saito C, Hasegawa G, Yano K, Yamamoto A, Yaguchi M, et al. Enhancement of immunologic tumor regression by intratumoral administration of dendritic cells in combination with cryoablative tumor pretreatment and Bacillus Calmette-Guerin cell wall skeleton stimulation. Clin Cancer Res. 2006; 12:7465–7475.