Abstract
Purpose
To evaluate the efficacy of low-dose computed tomography (LDCT) for detecting urinary stones with the use of an iterative reconstruction technique for reducing radiation dose and image noise.
Materials and Methods
A total of 101 stones from 69 patients who underwent both conventional nonenhanced computed tomography (CCT) and LDCT were analyzed. Interpretations were made of the two scans according to stone characteristics (size, volume, location, Hounsfield unit [HU], and skin-to-stone distance [SSD]) and radiation dose by dose-length product (DLP), effective dose (ED), and image noise. Diagnostic performance for detecting urinary stones was assessed by statistical evaluation.
Results
No statistical differences were found in stone characteristics between the two scans. The average DLP and ED were 384.60±132.15 mGy and 5.77±1.98 mSv in CCT and 90.08±31.80 mGy and 1.34±0.48 mSv in LDCT, respectively. The dose reduction rate of LDCT was nearly 77% for both DLP and ED (p<0.01). The mean objective noise (standard deviation) from three different areas was 23.0±2.5 in CCT and 29.2±3.1 in LDCT with a significant difference (p<0.05); the slight increase was 21.2%. For stones located throughout the kidney and ureter, the sensitivity and specificity of LDCT remained 96.0% and 100%, with positive and negative predictive values of 100% and 96.2%, respectively.
Urinary stones are one of the most prevalent diseases in the field of urology, but about 34% of ureteral stones cannot be detected by simple x-rays, and radiopaque stones are often difficult to locate within the exact lesion [1]. Intravenous pyelography (IVP) was previously used in diagnosing urinary stones; however, the inevitable intravenous injection of contrast media can cause infrequent but potentially lethal complications including acute allergic reactions or renal toxicity. IVP also has a limitation in the evaluation of renal stones or radiolucent ureter stones with reported sensitivity of 52% and specificity of 94% [2]. For these reasons, nonenhanced computed tomography (NECT), which shows higher sensitivity and specificity than IVP, has widely replaced conventional IVP for the examination of patients who present with symptoms of renal colic in the Emergency Department [3,4]. NECT allows for effective detection of conditions besides urinary stones that are known to cause unilateral flank pain. NECT is less time consuming than IVP, especially in patients with obstructing calculi, and reduces the patient's risk for complications due to intravenous contrast media [5]. However, despite the advantages of CT for the diagnosis of ureteral stones, the inherent radiation exposure applied to relatively young patients presenting with symptoms of urinary stones continues to raise concerns, particularly in patients undergoing repetitive studies [6,7]. The incidence of urinary stones is increasing, especially among children and young adults, and urinary stones have a tendency to recur. This leads to an accumulation of radiation through the patient's lifetime owing to frequent and repetitive radiologic evaluation. Radiation exposure might thus become a critical limitation of this examination [8]. Exposure to a very small amount of radiation can cause cancer, especially in younger patients, in whom exposure to radiation has been shown to increase the likelihood of cancer incidence [9,10].
Recently, the medical field and the public have become interested in the occurrence of cancer due to radiation exposure. Efforts to minimize radiation exposure from medical procedures have already begun, with special emphasis on the total accumulative dose in a lifetime, intensive exposure in the short term, and possible effects on cancer occurrence [11,12]. Reducing radiation exposure during the evaluation of renal colic is now one of the main interests in the field of urology, and low-dose CT (LDCT) is a most promising option for diagnosing urinary stones.
The recently introduced iterative reconstruction (IR) algorithms for CT have shown promising prospects with regard to dose reduction compared with standard filtered back projection (FBP). This is mainly due to the reduced image noise achieved by IR in the acquired scanning images[13].
Herein, we evaluated the efficacy of LDCT with the use of the IR technique for reducing radiation dose and image noise while maintaining clinical usefulness.
Between May and July of 2013, a total of 69 patients who had a diagnosis of urinary stones in our hospital by use of conventional NECT (CCT) underwent an additional scan by LDCT. Patients were included in the study after they provided detailed informed consent. Exclusion criteria were steinstrasse in which it was hard to determine the characteristics of urinary stones, possibility of pregnancy, a previously implanted device that could affect the quality of the results, previous history of malignancies, and urologic abnormalities such as horseshoe kidney, medullary sponge kidney, single kidney, and double collecting systems. This study was approved by the Chung-Ang University Hospital Institutional Review Board.
All CT scans were performed under automated exposure control and were reconstructed by use of FBP in the CCT setting and IR (iDOSE, Phillips Healthcare, Best, The Netherlands) in the LDCT setting. Both CCT and LDCT were performed without any oral or intravenous contrast media (Fig. 1).
A total of 101 stones (56 renal stones and 45 ureteric stones) were diagnosed by CCT. The age, sex, and body mass index (BMI) of the subjects were reviewed. Interpretations were made in both CT scans for stone characteristics (size, volume, location, Hounsfield unit [HU], and skin-to-stone distance [SSD]), and radiation (dose-length product [DLP] and effective dose [ED]) by a radiologist. Image noise was investigated by measuring the standard deviations (SDs) from the region of interest (ROI) on the basis of measurements from the picture archiving and communication system. The radiation doses (mGy) were from the estimation of DLP that was generated by the CT scanner. The ED was calculated from the DLP by multiplying it by the conversion coefficient (0.015 mSv/mGy/cm). The stone height, width, HU, and SSD (45 degree posterior-lateral) were measured in the axial views of the CT images, and the length of the stone was measured in the coronal view. Stone height, width, and length were used to calculate the volume of the stone by using the ellipsoid formula (4πR1R2R3/3). Diagnostic performance for detecting urinary stones was evaluated by statistical analyses.
All parametric variables were compared by using paired t-tests between the two CT protocols and were expressed as mean±SD. All statistical analyses were performed with IBM SPSS Statistics ver. 21.0 (IBM Co., Armonk, NY, USA), and were considered statistically significant with a p-value of <0.05.
Of the 69 patients, 44 were males and 25 were females. The mean age of the patients was 48.7 years (range, 19-86 years; median, 51 years), and the patients' average BMI was 24.37 kg/m2 (range, 17.26-33.41 kg/m2; median, 24.22 kg/m2). A total of 39 stones were <3 mm in maximum diameter, 55 stones were 3 to 10 mm, and 7 stones were >10 mm. Table 1 shows the comparative stone characteristics between CCT and LDCT. The mean stone height, width, and length were 4.27±3.02, 2.92±1.75, and 3.82±2.75 in CCT, and 4.27±3.09, 3.02±1.80, and 3.94±2.77 in LDCT, respectively. We found no significant differences between CCT and LDCT in the three stone parameters (p-values of 0.959, 0.074, and 0.071, respectively). For CCT and LDCT, respectively, the stone volumes were 66.29±200.62 mm3 and 69.16±205.25 mm3, HU was 483.58±330.38 and 506.15±368.26, and SSD was 98.51±27.38 and 96.76±29.22. We found no significant differences between CCT and LDCT for the stone volumes, HU, and SSD (p-values of 0.118, 0.108, and 0.135, respectively). For LDCT, the sensitivity, specificity, positive predictive value (PPV), and negative predictive value (NPV) were investigated according to stone locations on the basis of the reference diagnosis of CCT (Table 2). Stones located in the kidney showed sensitivity and specificity of 94.6% and 100% with PPV and NPV of 100% and 93.8%, respectively; stones located in the ureter showed sensitivity and specificity of 97.8% and 100% with PPV and NPV of 100% and 98.2%, respectively (Fig. 2). Overall, for stones located throughout the kidney and ureter, the sensitivity and specificity of LDCT remained 96.0% and 100% with PPV and NPV of 100% and 96.2%, respectively.
Table 3 shows the average radiation exposure dose with DLP and ED. The DLP and ED were 384.60±132.15 mGy and 5.77±1.98 mSv with CCT and 90.08±31.80 mGy and 1.34±0.48 mSv with LDCT; the dose reduction rate of LDCT was nearly 77% in both DLP and ED (p<0.01).
Objective image noise was also compared between the two CT protocols. We performed the measurement of SD from ROIs positioned in three different areas of the liver, spleen, and psoas muscle to obtain information related to objective noise as SD. The mean objective noise (SD) from the three different areas was 23.0±2.5 in CCT and 29.2±3.1 in LDCT with a significant difference (p<0.05); objective noise was only slightly increased (21.2%) compared with the reduction of radiation dose (77%) (Fig. 3).
Within the last few decades, nonenhanced CT has become an essential tool for the evaluation of urinary stones with high sensitivity and specificity of 94%-100% and 97%, respectively [14]. The larger doses and increased lifetime radiation risks in a young population have produced a sharp increase in estimated risk from radiation, however [9,15,16]. Recent studies have shown that the incidence of urinary stone disease may be increasing, with a lifetime incidence of 5% to 10% in the United States. It is estimated that 75% of patients with a single stone episode subsequently experience a recurrence during their lifetime [17,18]. Among other adverse effects, exposure to ionizing radiation can result in carcinogenesis, which has stochastic and unpredictable effects [19]. Carcinogenesis may transpire many years following exposure, and the risk of tumor induction is magnified [20]. Low-dose protocols for chest CT or abdomen CT for appendicitis are already established, and similar efforts are urgently required in the field of urology [11,12].
The recent advances in imaging technology have greatly improved the chances to decrease the radiation dose while reducing image noise in CT examination. IR and model-based iterative reconstruction (MBIR) can improve image quality and reduce image noise, which were the weak points of conventional LDCT reconstructed with FBP [13]. Although FBP shows results after the raw data are reconstructed from a CT scan, IR corrects the initial image after repeated comparisons of the measured raw data and the reconstructed image from the CT scan. MBIR is the advanced-generation IR technique, but the major limitation of MBIR is the time required to obtain multiple iterations. In a recent study, 35 to 40 minutes were required for image reconstruction with an MBIR algorithm [21]. This limits the use of this technique in emergency situations, which is unlike the case with the IR algorithm. Hence, MBIR/IR is widely used in various medical institutions.
Previous reports on IR in CT have demonstrated its efficacy in detecting urinary stone disease with reduced noise and radiation dose. Dose reduction by use of the IR technique does not seem to affect stone characteristics such as stone size, volume, SSD, and HU, which are essential parameters in making treatment decisions [22,23,24,25]. Stone size in CT is a major factor that can affect treatment options. Although improved image quality can be achieved by image noise reduction, the imaging will not be suitable for detection of ureteral stone disease if stone characteristics are not accurately reflected, i.e., the exact stone size comparable to CCT. Our results suggested that IR can reduce the radiation dose sufficiently without compromising the measurement of stone characteristics, including stone size. As shown in Table 2, the diagnostic performance of LDCT was also excellent for both kidney and ureteral stones.
Application of LDCT is suitable for the detection of urinary stone disease because of the high contrast between almost all urinary tract stones and the adjacent lower-density soft tissue, whereas the IR technique causes a reduction in radiation dose and image noise. Several reports have demonstrated that intraindividual dose reductions of up to 50% are feasible for abdominal CT while reducing the image noise by applying the IR technique compared with conventional CT [25,26]. However, note that both of those studies evaluated the IR technique with respect to low-contrast abdominal soft tissue structures such as liver parenchyma, and thus this technique is not applicable for the diagnosis of high-contrast structures such as urinary calculi, as in our study. In a pilot study consisting of 25 patients, Kulkarni et al. [24] found that application of the IR technique in LDCT for detection of urinary stones significantly reduced the radiation dose by about 82% on average compared with the conventional FBP protocol with CCT. In this study, LDCT provided a radiation dose reduction of about 77% on average, and the objective image noise was increased significantly by 21.2% on average compared with CCT. The average ED was only 1.39 mSv, and diagnostic performance was maintained.
Our study had a limitation in that only limited numbers of patient were enrolled. It was difficult to recruit volunteers for our study because of the patients' substantial concerns about additional radiation exposure. Despite patient agreement to undergo an additional CT scan, LDCT was not performed in patients without evidence of urinary stones in CCT. Because there might be an ethical problem with additional exposure of patients to radiation via CT by this approach, we made efforts to reduce radiation exposures in follow-up studies by checking symptoms with alternative methods such as urine chemistry or microscopic analysis and kidney ultrasonography or simple x-ray study, if possible. Also, informed consent was obtained only after thorough explanation of the possible harm of additional radiation exposure to all patients. Only patients who fully agreed were involved in this study. The economic effect of LDCT remains to be determined. In addition, the monetary cost for wide use of CT could negatively impact the overall cost in already financially strained health care systems. Although difficult to calculate, the cost considerations should include the potential carcinogenic sequels from exposure to the conventional radiation dose.
Our study also had some strengths. Unlike other previous studies, our study examined the same stones simultaneously with CCT and LDCT. The reference diagnosis was based on CCT and not subjective or indefinite symptoms. Thus, the results more precisely reflected the diagnostic performance. The approach of comparison between follow-up CT scans has a potential problem originating from differences in stone location. This could affect the transparency of radiation results at different attenuation levels and actual scanning plains, which could affect reported stone characteristics if the stone location is changed. Our data may suggest a helpful method to reduce radiation exposure and could be valuable as a pilot study for further detailed studies.
Figures and Tables
FIG. 1
Comparison of conventional nonenhanced computed tomography with filtered back projection (A, C) and low-dose computed tomography with iterative reconstruction technique (B, D) on a left proximal ureter stone (arrow).
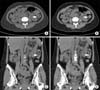
FIG. 2
Two tiny (<1 mm) right renal stones (arrow) were shown in conventional nonenhanced computed tomography (A, C) while not being detected in low-dose computed tomography (B, D).
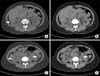
FIG. 3
Image qualities were compared on conventional nonenhanced computed tomography (A) and low-dose filtered back projection (B) with iterative reconstruction low-dose computed tomography (C).
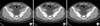
References
1. Chua ME, Gatchalian GT, Corsino MV, Reyes BB. Diagnostic utility of attenuation measurement (Hounsfield units) in computed tomography stonogram in predicting the radio-opacity of urinary calculi in plain abdominal radiographs. Int Urol Nephrol. 2012; 44:1349–1355.
2. Yilmaz S, Sindel T, Arslan G, Ozkaynak C, Karaali K, Kabaalioglu A, et al. Renal colic: comparison of spiral CT, US and IVU in the detection of ureteral calculi. Eur Radiol. 1998; 8:212–217.
3. Dalrymple NC, Verga M, Anderson KR, Bove P, Covey AM, Rosenfield AT, et al. The value of unenhanced helical computerized tomography in the management of acute flank pain. J Urol. 1998; 159:735–740.
4. Lim GS, Jang SH, Son JH, Lee JW, Hwang JS, Lim CH, et al. Comparison of non-contrast-enhanced computed tomography and intravenous pyelogram for detection of patients with urinary calculi. Korean J Urol. 2014; 55:120–123.
5. Abramson S, Walders N, Applegate KE, Gilkeson RC, Robbin MR. Impact in the emergency department of unenhanced CT on diagnostic confidence and therapeutic efficacy in patients with suspected renal colic: a prospective survey. 2000 ARRS President's Award. American Roentgen Ray Society. AJR Am J Roentgenol. 2000; 175:1689–1695.
6. John BS, Patel U, Anson K. What radiation exposure can a patient expect during a single stone episode? J Endourol. 2008; 22:419–422.
7. Brenner DJ, Hall EJ. Computed tomography: an increasing source of radiation exposure. N Engl J Med. 2007; 357:2277–2284.
8. Lopez M, Hoppe B. History, epidemiology and regional diversities of urolithiasis. Pediatr Nephrol. 2010; 25:49–59.
9. Brenner D, Elliston C, Hall E, Berdon W. Estimated risks of radiation-induced fatal cancer from pediatric CT. AJR Am J Roentgenol. 2001; 176:289–296.
10. Committee to Assess Health Risks from Exposure to Low Levels of Ionizing Radiation. Board on Radiation Effects Research (BRER). Division on Earth and Life Studies (DELS). National Research Council. Health risks from exposure to low levels of ionizing radiation: BEIR VII phase 2. Washington, D.C.: The National Academies Press;2006.
11. Gorycki T, Lasek I, Kamiński K, Studniarek M. Evaluation of radiation doses delivered in different chest CT protocols. Pol J Radiol. 2014; 79:1–5.
12. Kim K, Kim YH, Kim SY, Kim S, Lee YJ, Kim KP, et al. Low-dose abdominal CT for evaluating suspected appendicitis. N Engl J Med. 2012; 366:1596–1605.
13. Hansmann J, Schoenberg GM, Brix G, Henzler T, Meyer M, Attenberger UI, et al. CT of urolithiasis: comparison of image quality and diagnostic confidence using filtered back projection and iterative reconstruction techniques. Acad Radiol. 2013; 20:1162–1167.
14. Heneghan JP, McGuire KA, Leder RA, DeLong DM, Yoshizumi T, Nelson RC. Helical CT for nephrolithiasis and ureterolithiasis: comparison of conventional and reduced radiation-dose techniques. Radiology. 2003; 229:575–580.
15. Chargari C, Cosset JM. The issue of low doses in radiation therapy and impact on radiation-induced secondary malignancies. Bull Cancer. 2013; 100:1333–1342.
16. Rabes HM, Klugbauer S. Radiation-induced thyroid carcinomas in children: high prevalence of RET rearrangement. Verh Dtsch Ges Pathol. 1997; 81:139–144.
17. Bartoletti R, Cai T, Mondaini N, Melone F, Travaglini F, Carini M, et al. Epidemiology and risk factors in urolithiasis. Urol Int. 2007; 79:Suppl 1. 3–7.
18. Curhan GC. Epidemiology of stone disease. Urol Clin North Am. 2007; 34:287–293.
19. Koenig TR, Wolff D, Mettler FA, Wagner LK. Skin injuries from fluoroscopically guided procedures: part 1, characteristics of radiation injury. AJR Am J Roentgenol. 2001; 177:3–11.
20. Cardis E, Vrijheid M, Blettner M, Gilbert E, Hakama M, Hill C, et al. The 15-Country Collaborative Study of Cancer Risk among Radiation Workers in the Nuclear Industry: estimates of radiation-related cancer risks. Radiat Res. 2007; 167:396–416.
21. Vardhanabhuti V, Ilyas S, Gutteridge C, Freeman SJ, Roobottom CA. Comparison of image quality between filtered back-projection and the adaptive statistical and novel model-based iterative reconstruction techniques in abdominal CT for renal calculi. Insights Imaging. 2013; 4:661–669.
22. Hara AK, Paden RG, Silva AC, Kujak JL, Lawder HJ, Pavlicek W. Iterative reconstruction technique for reducing body radiation dose at CT: feasibility study. AJR Am J Roentgenol. 2009; 193:764–771.
23. Kambadakone AR, Chaudhary NA, Desai GS, Nguyen DD, Kulkarni NM, Sahani DV. Low-dose MDCT and CT enterography of patients with Crohn disease: feasibility of adaptive statistical iterative reconstruction. AJR Am J Roentgenol. 2011; 196:W743–W752.
24. Kulkarni NM, Uppot RN, Eisner BH, Sahani DV. Radiation dose reduction at multidetector CT with adaptive statistical iterative reconstruction for evaluation of urolithiasis: how low can we go? Radiology. 2012; 265:158–166.
25. Prakash P, Kalra MK, Kambadakone AK, Pien H, Hsieh J, Blake MA, et al. Reducing abdominal CT radiation dose with adaptive statistical iterative reconstruction technique. Invest Radiol. 2010; 45:202–210.
26. May MS, Wust W, Brand M, Stahl C, Allmendinger T, Schmidt B, et al. Dose reduction in abdominal computed tomography: intraindividual comparison of image quality of full-dose standard and half-dose iterative reconstructions with dual-source computed tomography. Invest Radiol. 2011; 46:465–470.