Abstract
Tissue engineering and stem cell transplantation are two important options that may help overcome limitations in the current treatment strategy for bladder dysfunction. Stem cell therapy holds great promise for treating pathophysiology, as well as for urological tissue engineering and regeneration. To date, stem cell therapy in urology has mainly focused on oncology and erectile dysfunction. The therapeutic potency of stem cells (SCs) was originally thought to derive from their ability to differentiate into various cell types including smooth muscle. The main mechanisms of SCs in reconstituting or restoring bladder function are migration, differentiation, and paracrine effects. Nowadays, paracrine effects of stem cells are thought to be more prominent because of their stimulating effects on stem cells and adjacent cells. Studies of stem cell therapy for bladder dysfunction have been limited to experimental models and have been less focused on tissue engineering for bladder regeneration. Bladder outlet obstruction is a representative model. Adipose-derived stem cells, bone marrow stem cells (BMSCs), and skeletal muscle-derived stem cells or muscle precursor cells are used for transplantation to treat bladder dysfunction. The aim of this study is to review stem cell therapy and updated tissue regeneration as treatments for bladder dysfunction and to provide the current status of stem cell therapy and tissue engineering for bladder dysfunction including its mechanisms and limitations.
Stem cells (SCs) are defined as cells with an ability to propagate through self-renewal and generate mature cells of multiple lineages through differentiation [1]. Various medical fields have studied SCs because of their unique abilities, including site-specific migration, plasticity, and potential for tissue repair or regeneration.
Bladder outlet obstruction (BOO) is a well-known bladder dysfunction model induced by benign prostatic hyperplasia or urethral stricture. Other bladder dysfunction models, including chronic ischemia, hyperlipidemia, and diabetes, are still in early stages of development.
When there is mild-to-moderate BOO and conservative treatments fail to protect the upper urinary tract, the only remaining treatment option is surgical reconstruction of the bladder. Unfortunately, the potential complications of metabolic imbalance from the use of a portion of the gastrointestinal tract to perform augmentation cystoplasty are well characterized [2].
Tissue engineering and SC transplantation are two important options that may help overcome the limitations of current treatment. Tissue engineering is an alternative reconstruction method for generating bladder tissue. Bladder cells are harvested from the diseased organ by autograft, separated into cell lines of interest in vitro, and expanded to sufficient quantities. These engineered cells can be seeded onto matrices ex vivo to create tissue for implantation back into the host [2]. Direct transplantation of SCs is a promising alternative when autologous cells from the bladder cannot be harvested.
SCs improve healing through cell replacement and repopulation with stimulation of cell proliferation and angiogenesis. While numerous reports have shown the ability of SC transplantation or engraftment in lung, liver, heart, and brain, data is scarce regarding SC transplantation for recovery of bladder function [3,4,5].
Studies of the use of SC therapy to treat bladder dysfunction have generally involved adult multipotent SCs. Although induced pluripotent stem cells (iPSCs) have been studied in the urologic oncology field, these cells have yet to be investigated for other urological conditions, such as bladder dysfunction.
The use of adult multipotent SCs circumvents some issues regarding the ethical use and acquisition of SCs and the potential for tumorigenesis or rejection by the recipient's immune system [6,7]. Mesenchymal SCs (MSCs), a group of adult SCs that can be harvested from almost every tissue of the body, hold particular promise for the treatment of urologic disease including bladder dysfunction [8]. These cells have great differentiation capabilities and stimulate paracrine effects to secrete a host of bioactive factors that are beneficial for tissue repair. Furthermore, MSCs, in conjunction with various scaffolds, can be used to regenerate bladder tissues for reconstructive engineering.
To date, there have been few studies on SC therapy in bladder dysfunction, as most studies have focused on oncology, stress urinary incontinence, and erectile dysfunction. Authors have reviewed SC therapy for bladder dysfunction before [9], but more recent in vivo and ex vivo studies have clarified the proposed mechanism.
In this review, we provide an overview of the use of SC therapy for bladder dysfunction induced by BOO and by other causes including chronic ischemia or hyperlipidemia. We identify SC sources and review several potential mechanisms of action. We provide a summary of the current status of SC therapy of bladder dysfunction, its limitations, and opportunities for further investigation. This review contains only preclinical studies, so we will not discuss the isolation and harvesting of SCs.
MSCs are self-renewing cells with the pluripotent property to differentiate into various cell types, including osteoblasts, chondrocytes, myocytes, adipocytes, and neurons [10].
While all MSCs including bone marrow-derived stem cells (BM-MSCs), skeletal muscle-derived stem cells (SkMSCs), and adipose tissue-derived stem cells (ADSCs) exhibit similar biological properties and capabilities, their availability differs greatly depending on the therapeutic purpose. For example, while BM-MSCs and SkMSCs require a long expansion time with complicated isolation procedure, ADSCs can be prepared in hours.
BM-MSCs are rare in bone marrow, and harvesting these cells can induce significant morbidity to patients [11]. Bone marrow contains two types of SCs, which are hematopoietic stem cells (HSCs) and MSCs. MSCs can easily be separated from HSCs using their adherence to glass or plastic [12,13]. Their multipotentiality, relative ease of isolation, and high capacity for in vitro expansion enable a wide range of uses in tissue engineering, in vitro, ex vivo, and in vivo functional studies, and therapeutic trials [14,15].
ADSCs are mesenchymal stromal cells found in the perivascular space of adipose tissue. ADSCs have the advantage of abundant and easy access when compared with other SC types [16]. ADSCs express similar SC surface markers and differentiation potentials as MSCs [17]. ADSCs have demonstrated efficacy in experimental studies of urologic fields [18,19]. Muscle precursor cells (MPCs) or SkMSCs that are predecessors of satellite cells are considered not to be restricted to myogenic or mesenchymal tissues [20].
In a recent study, muscle-derived multipotent SCs (CD34-/45- and CD34-/CD45-) from green fluorescent protein (GFT) transgenic mouse muscles were transplanted into a nude rat model for regeneration of bladder dysfunction [21]. After SC transplantation, a significantly higher functional recovery was noted. In another study, muscle-derived SCs were reported to differentiate into bladder smooth muscle cells (SMCs) [22] and demonstrate contractility after seeding [23]. SkMSCs were reported to be useful mainly in artificial injury models including pelvic nerve injury [21,24]. The main advantage of SkMSCs is that they are source of autologous transplantation. Skeletal muscle is the largest organ in the body, and SkMSCs can be obtained relatively easily and safely during surgery.
Transplantation of SkMSCs can cause significant functional recovery through cellular differentiation into skeletal muscle cells, vascular cells (vascular SMCs, pericytes, and endothelial cells), and peripheral nervous cells (Schwann cells and perineurium) [25,26].
Despite significant progress in characterizing MSCs in vitro, the in vivo characteristics of MSCs remain inconclusive. The location and cell surface marker expression of MSCs are similar to those of perivascular cells, and a subset of pericytes maintains the in vitro differentiation capacity of MSCs [27]. MSCs could be a subset of pericytes, which would explain their presence in almost every organ of the body. However, human BMSCs and ADSCs have pericyte-like marker expression and phenotype in hypoxic and inflammatory conditions [28]. These findings suggest that MSCs hold pericyte-like functions in such conditions to reduce inflammatory damage and improve vascularity.
Recently, multipotential differentiation of human urine-derived SCs (USCs) was reported. USCs were able to differentiate into endothelial, osteogenic, chondrogenic, adipogenic, skeletal myogenic, and neurogenic lineages, which implies the possibility of USCs for urological application including reconstruction [29].
Regardless of their critical location within tissues, MSCs hold niche specificity. MSCs from different tissues require different niches for in vitro differentiation and also have different gene expression profiles [30,31]. This variance implies the potential of MSCs to differentiate and secrete bioactive factors. As a consequence, certain types of MSCs might be more appropriate for treating particular dysfunctions.
SCs are self-renewing adult stem cells with multipotent differentiation potentials. SCs can become many types of tissues through transdifferentiation or cell fusion, resulting in regeneration and functional restoration [32]. Moreover, SCs are an important source for cell replacement [33]. The mechanism of bladder recovery is a restoration including cell differentiation, paracrine activity of SCs, and interaction with microenvironment signals [34]. Homing and migration of other hematopoietic MSCs to injured sites are achieved by chemoattraction through microenvironment niche conditions, resulting in acceleration of bladder regeneration [8].
To date, the potential role of SCs in functional recovery in bladder dysfunction is incompletely understood. Animal studies have not yet elucidated the distinct mechanisms of action, and the current results are also conflicting. Most studies demonstrated that SCs are not preserved in the target organ for specific periods of time after acute injury. Differentiation is also not consistently present during recovery. Thus, paracrine effects via bioactive factors are more responsible for the improvements in functional parameters.
The role of SCs in functional recovery is dependent not only on inherent cellular and regional characteristics, but also on the nature of the pathology. A comprehensive understanding of the mechanism of SCs should be elucidated.
Recruitment of SCs to the bladder in BOO is associated with tissue hypoxia, which contributes to improvement in histopathological and functional parameters [35].
SCs derived from the bone marrow circulate throughout the blood and migrate to injured tissue. Their migration is affected by expression of cytokines released by damaged tissues [36]. Homing cytokines, such as stromal derived factor-1, have been shown to improve functional outcomes when delivered directly to hearts with ischemic cardiomyopathy in rat models [37]. Recruitment of SCs occurs in sites of inflammation, ischemia, or tissue damage [38]. A large body of literature exists on recruited SCs in a number of different organ systems including injured bladder [39,40,41,42].
Although local transplantation of SCs results in immediate recruitment to the affected tissue, the homing of SCs may also be observed after systemic delivery. With systemic delivery, SCs can be recruited to target tissues with lower levels of focal injury and can be modulated by the immune response via splenic localization. After an acute injury, however, homing of transplanted SCs may not be achieved because homing can be impaired by a lack of developing tissue damage and sequent cytokine expression [43].
Differentiation is an ideal treatment mechanism of SC therapy, but only a few studies have demonstrated true differentiation into bladder muscle (Table 1). Differentiation or transdifferentiation into specific tissues such as muscle or nerve is still being studied in challenging trials. To date, the differentiation pathway is known to play at most a minor role in the therapeutic effect of SC transplantation. One explanation is the immortal strand hypothesis in which labeled DNA in dividing cells are quickly diluted by cell divisions whereas dilution takes much more time for dividing SCs [47].
Successful differentiation of SCs into smooth muscle for bladder repair and replacement has been reported in several studies of bladder regeneration with tissue engineering [48,49].
SC therapy in urology has largely focused on the induced differentiation of SCs in the field of stress urinary incontinence and urothelial reconstruction [50]; however, several studies have demonstrated that MSCs can differentiate into epithelial-like cells under appropriate conditions [51,52]. To date, the ex vivo differentiation of SCs is a vital step in the use of tissue engineering for reconstruction.
Two major structural proteins in SMCs are smooth muscle actin (SMA) and smoothelin. They have been used to verify the regeneration of muscle tissue. SMA is an early and general marker of developing smooth muscle, and smoothelin is a marker of the differentiated phenotype. The expression of SMA is restricted to functional smooth muscle, but the expression of smoothelin is not restricted to smooth muscle [53]. In BOO models, bladder dysfunction treated with ADSCs and MPCs showed high levels of both SMA and smoothelin expression. Smooth muscle regeneration was mainly achieved by paracine factors or by direct differentiation into new smooth muscle tissue by injecting SCs [54]. The interaction of cell surface receptors, ligands, and short-range-acting molecules between differentiated and undifferentiated cells significantly contributes to SC differentiation [55].
While differentiation has long been considered the main mechanism, newer studies support the more prominent role of the paracrine effect in the mechanism for observed positive functional effects after SC transplantation. Paracrine effects include the direct paracrine release of cytokines and growth factors by transplanting MSCs or the indirect release through neighboring cells. Recently, a paradigm shift in SC biology has focused attention on the paracrine, autocrine, and growth factor effects of SCs.
The therapeutic effects of SC secretory factors include modulation of local and systematic inflammatory responses and stimulation of local tissue regeneration. MSCs themselves cannot substitute for damaged tissue directly or totally, but they can secrete growth factors or cytokines and contribute to reducing fibrosis [56].
SCs may secrete many growth factors, including hepatic growth factor (HGF), nerve growth factor (NGF), brain-derived growth factor, glial-derived growth factor, insulin-like growth factor, vascular endothelial growth factor, and ciliary neurotrophic growth factor. They play an essential part in the reduction of fibrosis in injured organs, which implies that paracrine effects play a more crucial role in the restoration of the injured organ by reducing fibrosis rather than by cell incorporation [39,57,58]. Among the growth factors, HGF is currently receiving intense research interest because of its potent antifibrotic characteristics. HGF is a mitogen for hepatocytes, is secreted by MSCs, and plays an essential part in the angiogenesis and regeneration of the tissue [56,59].
In addition to antifibrotic mechanisms, BM-MSCs or ADSCs may provide antioxidant chemicals, free radical scavengers, and heat shock proteins to ischemic tissue [60].
MSCs are known to have defensive actions against apoptosis and scarring, as well as neovascularization effects. MSCs have systemic and local immunomodulatory properties, including the inhibition of T-cell and B-cell proliferation [61]. MSCs also activate the endogenous stem and progenitor cells in areas of injured tissue by secreting cytokines and chemokines.
The tissue engineering approach to urinary bladder regeneration requires a favorable environment. Embryoid body-derived SCs, or BMSCs seeded on small intestinal submucosa (SIS), facilitated the regeneration of partially cystectomized bladder [62,63,64]. Recently, hair SCs and ADSCs seeded on bladder acellular matrix (BAM) have demonstrated the potential to regenerate bladder [65,66]. SCs are effective not only in SIS or BAM but also as part of synthetic scaffolds. Sharma et al. [67] reported that BMSCs seeded on poly (1,8-octanediol-co-citrate) thin film supported partial bladder regeneration. Tian et al. [68] showed that myogenically differentiated BMSCs seeded on poly-l-lactic acid scaffold exhibited bladder engineering potential. A synthetic material of poly-lactic-glycolic acid seeded with myogenically differentiated human ADSC showed the recovery of bladder capacity and compliance when grafted in hemicystectomized rats [48].
Bladder tissue engineering using MSCs may show better results than using differentiated SCs or direct transplantation of SCs. MSCs were shown to migrate to the bladder grafts and differentiate into SMCs [21,68], resulting in fast replacement of the grafts with appropriate neural function and less fibrosis [69].
Lai et al. [70] demonstrated that transplanting either healthy or diseased bladder tissue could be regenerated into functional muscle and urothelium tissue in vitro. For clinical studies, Atala et al. [71] reported the effects of autologous bladder biopsy-derived cells in patients with low compliance bladder treated with augmentation cystoplasty. The authors reported improved bladder function after cystoplasty over 5 years. Although this new approach enables the incorporation of SCs and scaffolds, it also has limitations. Diseased urothelium displays reduced proliferative and differentiation capacity in vitro [72].
Considering the risk of harvested autologous bladder tissue ambushing microscopic disease, alternative sources of cells, such as allogenic SCs or autologous SCs from other organs, should be investigated.
The optimal scaffold type for bladder tissue engineering has yet to be established. Results associated with the use of SC-seeded scaffolds for cystoplasty vary and are influenced by the character of the SIS scaffold setting [73]. Chung et al. [62] reconstructed rat bladder with SIS seeded with BMSCs and demonstrated the regeneration of organized urothelium, discrete distributed muscle fascicles, and nerve. Sharma et al. [74] reported bladder augmentation using MSC-seeded SIS in baboons and reported the resulting typical histoarchitecture of the bladder. The morphology of bladder architecture more closely matched normal bladder when SC-seeded scaffolds were used. Synthetic scaffolds have the advantage of avoiding vulnerable issues such as structure variability and host response that are commonly associated with biological alternatives. Sharma et al. [67] utilized the poly (1,8-octanediol-co-citrate; POC) to reconstruct partially cystectomized, athymic nude rats. POC scaffolds seeded with human MSCs and urothelial cells, showed partial regeneration of bladder tissue in vivo. Other alternative scaffolds, such as 3-dimentional nano scaffolds seeded with predifferentiated BMSCs [68] and synthetic bladder composites seeded with ADSCs, have potential for future use [48].
Human MSCs may express SMC-specific genes and proteins during the cocultured period. The MSC-seeded scaffold showed a significant improvement in cell contractility after myogenic differentiation [52] and can induce transdifferentiation, cell fusion, and paracrine signaling [13]. The presence of endogenous muscle cells provides a reasonable target for paracrine signaling by MSCs. Both mediators and direct cell-to-cell contacts induce the differentiation of muscle cells [75].
To date, the BOO model is the most prominent model for bladder dysfunction, and other pathologic models are still in early stages of development. BOO is one of the most common problems in elderly males. It is caused by collagen deposition in the bladder as a result of various pathological processes. Eventually, this collagen deposition results in bladder fibrosis and induces a flaccid, noncompliant bladder. Bladder fibrosis adversely affects the composition of the bladder wall by reducing the smooth muscle component, resulting in low bladder compliance [76]. In the acute stage, BOO induces bladder instability [77]. Compensated bladder dysfunction with an overactive bladder often occurs after 6 weeks [76]. Most studies that have dealt with models of decompensated bladder dysfunction carry a disease period of more than 6 weeks. SC therapy in BOO is described in Table 1, which demonstrates the detailed characteristics of each study.
To track the SCs after transplantation, most studies used the GFP labeling method. With this method, cell survival or migration could be identified after transplantation.
Recently, Lee et al. [40] have reported that transplantation of human MSCs labeled with nanoparticles containing superparamagnetic iron oxide into the bladder inhibited bladder fibrosis and induced improvement of bladder dysfunction.
Growth factors have been reported in the remodeling of the bladder wall after BOO [78]. Song et al. [41] demonstrated that transplantation of human MSCs with overexpressing HGF by pairing clonal human MSCs with HGF inhibited collagen deposition and improved functional outcomes in BOO.
The pathologic mechanism for bladder dysfunction is decreased local blood flow, which causes significant tissue ischemia. Increased intraluminal pressure causes vessel compression, and ischemia is further aggravated by fibrosis and hypertrophy [79,80].
Nishijima et al. [81] showed that transplantation of bone marrow stromal cells (BMSCs) into the bladder could improve bladder contractility by differentiating into smooth muscle-like cells in an underactive bladder model. This study demonstrated the differentiation of cells labeled with GFP into smooth muscle tissue, but the isolation techniques used in this study did not specifically sort for BMSCs [81]. This implies that other muscle progenitor cells, such as satellite cells, could have differentiated into smooth muscle tissue in vivo instead.
Woo et al. [42] reported that engrafted MSCs had improved compliance compared to those without engraftment. They performed the transplantation by systemic injection and observed evidence of histological and functional improvement. This study also demonstrated the benefits of SC transplantation for bladder remodeling and the significance of paracrine mechanisms by demonstrating a positive functional outcome after systemic injection of a few homing transplanted SCs [42,82].
Among the chemokines, CCL2 expression increased two folds after polymerase chain reaction, but there were no significant changes in other chemokines. CCL2 in the BOO model was identified by Tanaka et al. [83], as well. After direct transplantation of bone marrow derived cells into bladder, SCs were present in the urothelial and stromal layers after BOO. An activated epidermal growth factor receptor was present in cells associated with bone marrow derived cells [83].
Recently, Song et al. [84] reported that transplanted MSCs induce the stimulation of host endogenous SCs. They reported that MSC transplantation appeared to be superior to solifenacin, currently one of the most commonly used pharmacotherapies. The result of activating Oct4+/Sox2+/Stella+ primitive stem cells in host tissues implies the possibility of the emergence of the host's primitive pluripotent stem cells (PSCs). Accumulating evidence suggests that adult tissues contain a very small population of PSCs, which have been alternatively described as MSCs, multipotent adult progenitor-cells, marrow-isolated adult multilineage-inducible cells, multipotent adult stem cells, and very small embryonic-like stem cells [85,86].
Recently, there has been a general paradigm shift in SC biology toward utilizing MSCs as vehicles for the delivery of bioactive factors. Systemic injection of BMSCs or a concentrate of the conditioned media produced favorable functional and histologic outcomes in vitro [87].
Bladder ischemia models have been introduced to serve as a model for overactivity. The bladder ischemia model is established using bilateral iliac artery ligation [88] or hyperlipidemia [82]. Artery stenosis and vascular insufficiency can cause significant changes in the structure and function of the bladder [89]. The mechanism of ischemia-induced bladder dysfunction is complicated and related to ischemic denervation. This causes M-cholinergic receptor hypersensitivity to acetylcholine [90] and results in detrusor overactivity, which leads to aggravation of bladder ischemia. Considering the high rate of ischemic changes in the elderly, it is possible that the ischemic bladder model could also be used with BOO models for an aging bladder model.
Chen et al. [88] reported that pathological and functional changes of the bladder ischemic model are similar to the aging human bladder. They reported that SC transplantation via common iliac artery in the ischemia bladder model could regenerate bladder tissue, improve the structure of smooth muscle, and improve functional outcomes. In their study, intragastric doxazosin mesylate was given after SC transplantation.
Huang et al. [82] showed that direct transplantation into the bladder or systemic intravenous transplantation of ADSCs improved functional outcomes in their hyperlipidemia-induced overactive bladder model. They demonstrated that SC transplantation could enhance angiogenesis and cholinergic innervation. They tracked the ADSCs and discovered that more are collected within the bladder following local injection compared to systemic injection. Azadzoi et al. [91] found that chronic ischemia induced by hyperlipidemia could increase transforming growth factor-β1 in the bladder, which leads to fibrosis.
Diabetic bladder dysfunction (DBD) typically results in a time-dependent progression of both storage and voiding problems. The early phase of DBD manifests as detrusor overactivity. Over time, DBD represents decompensation of the detrusor muscle and finally underactive or flaccid bladder. The main pathologic mechanism for DBD is oxidative stress and neuropathy. Indeed, the DBD, ischemic bladder, and BOO models share a similar pathologic mechanism of oxidative stress and progressive decompensation. In these fields, SC transplantation could restore injured bladder tissue by differentiation or improve ischemia by angiogenesis or activation of biomarkers.
Zhang et al. [92] reported that improved voiding function was noted in ADSC-treated rats with an underactive DBD model. Although some ADSCs differentiated into SMCs, paracrine activity seems to play a main role by reducing apoptosis and preserving the "suburothelial capillary network."
Until recently, there has been little research on gene therapy for the urinary tract. Most of the previous work focused on urinary tract malignancies, such as prostate and bladder cancer. The pioneer gene therapies performed in bladder dysfunction were for overactive bladder and DBD. A study of gene therapy for an overactive bladder was performed by intra-vesicular instillation of naked pcDNA/hSlo cDNA for K+ channel [94]. This approach increased the number of K+ channels in the smooth muscle of the bladder. Diabetic animals exhibit a decrease in NGF production in target tissues [95] and reduced retrograde axonal transport of NGF and other proteins [95].
Morris et al. [96] were among the first group to introduce the process of gene therapy in the bladder using adenoviral vectors. Gene transfer to rat bladders was accomplished via direct intra-vesicular instillation using a replication-defective adenoviral vector encoding the β-galactosidase gene.
Goins et al. [97] have designed herpes simplex virus (HSV)-1 vectors that have enabled long-term β-NGF expression in the dorsal root ganglion. They also performed preliminary experiments injecting HSV: latency active promoter 2 vectors carrying the NGF gene (SLN) into the bladder in a rat DBD model [97].
In gene therapy, effective gene transfer into SCs must be achieved without inducing detrimental effects on their biological properties. Although gene modification of MSCs to overexpress HGF showed favorable results in maintaining or enhancing the capacity of MSCs and efficacy in bladder fibrosis therapy [41], the choice of vector for cell transduction should be carefully considered. This problem is not confined to urologic fields but rather applies to the whole field of gene therapy. Ideal vectors do not evoke the host immune system or induce inflammation. Such vectors do not yet exist. To date, there are a few studies using gene therapy with viral or other vectors in bladder dysfunction with the exception of transfection of GFP or other tracking proteins. More studies are needed to evaluate the role of gene therapy using SCs in bladder dysfunction.
The biggest obstacle in current preclinical studies of bladder dysfunction is that there are no intermediary examinations. Without intermediary examination, such as survival urodynamics or bladder biopsy at a midpoint in the experiment, researchers cannot judge whether SCs may serve a more preventative or ameliorative role. It is hard to know whether the current BOO model could be later reversed or not.
Tumorigenesis is one of the big obstacles in SC therapy. All SC types cannot avoid the main issue of tumorigenesis, and the effect of SC therapy on encouraging the growth or metastasis of existing tumors has not yet been clarified.
Conventional SC therapy has focused mainly on the ability of cells to differentiate and replace the damaged or injured tissue. However, increasing evidence suggests that SCs also exert functional activity by secreting bioactive factors which stimulate local and systemic responses. For tissue engineering applications, SCs could be seeded onto scaffolds to facilitate the incorporation of the graft by native tissue. Neovascularization and reinnervation of engineered tissues are critical obstacles to overcome for future application to bladder reconstruction. Ingrowth of native vessels and nerves-which is stimulated by MSC bioactive factors-is acceptable for smaller grafts; however, complete regeneration with functional integration is far more challenging. Studies have shown the feasibility of nerve regeneration via the overexpression of neurotrophins, transcription factors, and cytoskeleton-related genes in patients with peripheral nerve injury [98].
Although preclinical data and clinical case reports have shown positive effects of SC treatment, including SC transplantation and tissue engineering for regeneration, more research is needed to elucidate the mechanisms of local and systemic effects of SCs before they can be fully integrated into clinical practice for bladder dysfunction.
The major limitation of homing or migration is the time delay between injury and the initiation of treatment. After an acute injury, homing signals are often diminished with time.
Research is needed to improve the accuracy of predictions regarding disease progression. It is important to determine the duration of time after injury that equates to the optimal release of homing bioactive factors and to develop innovative methods for up-regulating the expression of these cytokines. Methods such as electrical stimulation or local injection of homing cytokines could help direct the recruitment of systemically delivered SCs to the target organ.
Along with gene therapy, the selected vector should have high transduction efficiency and should ensure stability, which guarantees the long-term transgene expression from the cell vehicle and avoidance of any damaging effects on cell viability.
There are interesting results with experimental use of SCs to treat bladder dysfunction. The main mechanism for improved bladder function may be due to either prevention or resolution of fibrosis and restoration of bladder architecture. Although successful differentiation of SCs into smooth muscle for bladder repair and replacement was reported by several groups, paracrine activity with bioactive cytokines may be the crucial mechanism. The applicability of SCs to the treatment and/or prevention of bladder dysfunction by BOO would provide a new, potentially powerful addition to the limited armamentarium of existing therapies.
Although significant challenges are still needed to overcome the challenges for human application, this novel technology has the potential to become a major source of cells for treatment of bladder dysfunction. More studies are needed to investigate the clear mechanism of SC treatment, including stimulating the bioactive cytokines in donor SCs/host cells and also local or systemic proactive effects.
ACKNOWLEDGEMENTS
This research was supported by the Research Program through the National Research Foundation of Korea (NRF) funded by the Ministry of Education, Science and Technology (2012-R1A1A2039317) and the Soonchunhyang University Research Fund. We are indebted to Eun Ho Choi, a reference librarian at the Soonchunhyang University Medical Library, Seoul, Republic of Korea, for her help with the editing of this manuscript.
References
1. Masters JR, Kane C, Yamamoto H, Ahmed A. Prostate cancer stem cell therapy: hype or hope? Prostate Cancer Prostatic Dis. 2008; 11:316–319. PMID: 18427568.


2. Stanasel I, Mirzazadeh M, Smith JJ 3rd. Bladder tissue engineering. Urol Clin North Am. 2010; 37:593–599. PMID: 20955910.


3. Li JH, Zhang N, Wang JA. Improved anti-apoptotic and anti-remodeling potency of bone marrow mesenchymal stem cells by anoxic pre-conditioning in diabetic cardiomyopathy. J Endocrinol Invest. 2008; 31:103–110. PMID: 18362500.


4. Sakaida I, Terai S, Yamamoto N, Aoyama K, Ishikawa T, Nishina H, et al. Transplantation of bone marrow cells reduces CCl4-induced liver fibrosis in mice. Hepatology. 2004; 40:1304–1311. PMID: 15565662.


5. Zhao DC, Lei JX, Chen R, Yu WH, Zhang XM, Li SN, et al. Bone marrow-derived mesenchymal stem cells protect against experimental liver fibrosis in rats. World J Gastroenterol. 2005; 11:3431–3440. PMID: 15948250.


6. Bongso A, Fong CY, Gauthaman K. Taking stem cells to the clinic: major challenges. J Cell Biochem. 2008; 105:1352–1360. PMID: 18980213.


7. Choumerianou DM, Dimitriou H, Kalmanti M. Stem cells: promises versus limitations. Tissue Eng Part B Rev. 2008; 14:53–60. PMID: 18454634.


8. Kolf CM, Cho E, Tuan RS. Mesenchymal stromal cells. Biology of adult mesenchymal stem cells: regulation of niche, self-renewal and differentiation. Arthritis Res Ther. 2007; 9:204. PMID: 17316462.
9. Kim JH, Lee SR, Song YS, Lee HJ. Stem cell therapy in bladder dysfunction: where are we? And where do we have to go? Biomed Res Int. 2013; 2013:930713. PMID: 24151627.


10. Jiang Y, Jahagirdar BN, Reinhardt RL, Schwartz RE, Keene CD, Ortiz-Gonzalez XR, et al. Pluripotency of mesenchymal stem cells derived from adult marrow. Nature. 2002; 418:41–49. PMID: 12077603.


11. Furuta A, Carr LK, Yoshimura N, Chancellor MB. Advances in the understanding of sress urinary incontinence and the promise of stem-cell therapy. Rev Urol. 2007; 9:106–112. PMID: 17934567.
12. Colter DC, Class R, DiGirolamo CM, Prockop DJ. Rapid expansion of recycling stem cells in cultures of plastic-adherent cells from human bone marrow. Proc Natl Acad Sci U S A. 2000; 97:3213–3218. PMID: 10725391.


13. Pittenger MF, Mackay AM, Beck SC, Jaiswal RK, Douglas R, Mosca JD, et al. Multilineage potential of adult human mesenchymal stem cells. Science. 1999; 284:143–147. PMID: 10102814.


14. Campagnoli C, Roberts IA, Kumar S, Bennett PR, Bellantuono I, Fisk NM. Identification of mesenchymal stem/progenitor cells in human first-trimester fetal blood, liver, and bone marrow. Blood. 2001; 98:2396–2402. PMID: 11588036.


15. Strauer BE, Brehm M, Zeus T, Kostering M, Hernandez A, Sorg RV, et al. Repair of infarcted myocardium by autologous intracoronary mononuclear bone marrow cell transplantation in humans. Circulation. 2002; 106:1913–1918. PMID: 12370212.


16. Zuk PA, Zhu M, Mizuno H, Huang J, Futrell JW, Katz AJ, et al. Multilineage cells from human adipose tissue: implications for cell-based therapies. Tissue Eng. 2001; 7:211–228. PMID: 11304456.


17. Lin G, Garcia M, Ning H, Banie L, Guo YL, Lue TF, et al. Defining stem and progenitor cells within adipose tissue. Stem Cells Dev. 2008; 17:1053–1063. PMID: 18597617.


18. Albersen M, Fandel TM, Lin G, Wang G, Banie L, Lin CS, et al. Injections of adipose tissue-derived stem cells and stem cell lysate improve recovery of erectile function in a rat model of cavernous nerve injury. J Sex Med. 2010; 7:3331–3340. PMID: 20561166.


19. Huang YC, Ning H, Shindel AW, Fandel TM, Lin G, Harraz AM, et al. The effect of intracavernous injection of adipose tissue-derived stem cells on hyperlipidemia-associated erectile dysfunction in a rat model. J Sex Med. 2010; 7(4 Pt 1):1391–1400. PMID: 20141586.


20. Jankowski RJ, Deasy BM, Huard J. Muscle-derived stem cells. Gene Ther. 2002; 9:642–647. PMID: 12032710.


21. Nitta M, Tamaki T, Tono K, Okada Y, Masuda M, Akatsuka A, et al. Reconstitution of experimental neurogenic bladder dysfunction using skeletal muscle-derived multipotent stem cells. Transplantation. 2010; 89:1043–1049. PMID: 20150836.


22. Yokoyama T, Huard J, Pruchnic R, Yoshimura N, Qu Z, Cao B, et al. Muscle-derived cell transplantation and differentiation into lower urinary tract smooth muscle. Urology. 2001; 57:826–831. PMID: 11306423.


23. Lu SH, Cannon TW, Chermanski C, Pruchnic R, Somogyi G, Sacks M, et al. Muscle-derived stem cells seeded into acellular scaffolds develop calcium-dependent contractile activity that is modulated by nicotinic receptors. Urology. 2003; 61:1285–1291. PMID: 12809930.


24. Kwon D, Minnery B, Kim Y, Kim JH, de Miguel F, Yoshimura N, et al. Neurologic recovery and improved detrusor contractility using muscle-derived cells in rat model of unilateral pelvic nerve transection. Urology. 2005; 65:1249–1253. PMID: 15922415.


25. Tamaki T, Okada Y, Uchiyama Y, Tono K, Masuda M, Wada M, et al. Synchronized reconstitution of muscle fibers, peripheral nerves and blood vessels by murine skeletal muscle-derived CD34(-)/45(-) cells. Histochem Cell Biol. 2007; 128:349–360. PMID: 17762938.


26. Tamaki T, Uchiyama Y, Okada Y, Ishikawa T, Sato M, Akatsuka A, et al. Functional recovery of damaged skeletal muscle through synchronized vasculogenesis, myogenesis, and neurogenesis by muscle-derived stem cells. Circulation. 2005; 112:2857–2866. PMID: 16246946.


27. Crisan M, Yap S, Casteilla L, Chen CW, Corselli M, Park TS, et al. A perivascular origin for mesenchymal stem cells in multiple human organs. Cell Stem Cell. 2008; 3:301–313. PMID: 18786417.


28. Amos PJ, Mulvey CL, Seaman SA, Walpole J, Degen KE, Shang H, et al. Hypoxic culture and in vivo inflammatory environments affect the assumption of pericyte characteristics by human adipose and bone marrow progenitor cells. Am J Physiol Cell Physiol. 2011; 301:C1378–C1388. PMID: 21865587.


29. Bharadwaj S, Liu G, Shi Y, Wu R, Yang B, He T, et al. Multipotential differentiation of human urine-derived stem cells: potential for therapeutic applications in urology. Stem Cells. 2013; 31:1840–1856. PMID: 23666768.


30. Estes BT, Wu AW, Guilak F. Potent induction of chondrocytic differentiation of human adipose-derived adult stem cells by bone morphogenetic protein 6. Arthritis Rheum. 2006; 54:1222–1232. PMID: 16572454.


31. Silva WA Jr, Covas DT, Panepucci RA, Proto-Siqueira R, Siufi JL, Zanette DL, et al. The profile of gene expression of human marrow mesenchymal stem cells. Stem Cells. 2003; 21:661–669. PMID: 14595126.


32. Zubko R, Frishman W. Stem cell therapy for the kidney? Am J Ther. 2009; 16:247–256. PMID: 19092639.


33. Sharma AD, Cantz T, Manns MP, Ott M. The role of stem cells in physiology, pathophysiology, and therapy of the liver. Stem Cell Rev. 2006; 2:51–58. PMID: 17142887.


34. Hsiao ST, Asgari A, Lokmic Z, Sinclair R, Dusting GJ, Lim SY, et al. Comparative analysis of paracrine factor expression in human adult mesenchymal stem cells derived from bone marrow, adipose, and dermal tissue. Stem Cells Dev. 2012; 21:2189–2203. PMID: 22188562.


35. Ortiz LA, Gambelli F, McBride C, Gaupp D, Baddoo M, Kaminski N, et al. Mesenchymal stem cell engraftment in lung is enhanced in response to bleomycin exposure and ameliorates its fibrotic effects. Proc Natl Acad Sci U S A. 2003; 100:8407–8411. PMID: 12815096.


36. Roufosse CA, Direkze NC, Otto WR, Wright NA. Circulating mesenchymal stem cells. Int J Biochem Cell Biol. 2004; 36:585–597. PMID: 15010325.


37. Sundararaman S, Miller TJ, Pastore JM, Kiedrowski M, Aras R, Penn MS. Plasmid-based transient human stromal cell-derived factor-1 gene transfer improves cardiac function in chronic heart failure. Gene Ther. 2011; 18:867–873. PMID: 21472007.


38. Chamberlain G, Wright K, Rot A, Ashton B, Middleton J. Murine mesenchymal stem cells exhibit a restricted repertoire of functional chemokine receptors: comparison with human. PLoS One. 2008; 3:e2934. PMID: 18698345.


39. Abdel Aziz MT, Atta HM, Mahfouz S, Fouad HH, Roshdy NK, Ahmed HH, et al. Therapeutic potential of bone marrow-derived mesenchymal stem cells on experimental liver fibrosis. Clin Biochem. 2007; 40:893–899. PMID: 17543295.


40. Lee HJ, Won JH, Doo SH, Kim JH, Song KY, Lee SJ, et al. Inhibition of collagen deposit in obstructed rat bladder outlet by transplantation of superparamagnetic iron oxide-labeled human mesenchymal stem cells as monitored by molecular magnetic resonance imaging (MRI). Cell Transplant. 2012; 21:959–970. PMID: 22449414.


41. Song YS, Lee HJ, Doo SH, Lee SJ, Lim I, Chang KT, et al. Mesenchymal stem cells overexpressing hepatocyte growth factor (HGF) inhibit collagen deposit and improve bladder function in rat model of bladder outlet obstruction. Cell Transplant. 2012; 21:1641–1650. PMID: 22506988.


42. Woo LL, Tanaka ST, Anumanthan G, Pope JC 4th, Thomas JC, Adams MC, et al. Mesenchymal stem cell recruitment and improved bladder function after bladder outlet obstruction: preliminary data. J Urol. 2011; 185:1132–1138. PMID: 21255803.


43. Vaegler M, Lenis AT, Daum L, Amend B, Stenzl A, Damaser MS, et al. Stem cell therapy for voiding and erectile dysfunction. Nat Rev Urol. 2012; 6. 19. [Epub]. http://dx.doi.org/10.1038/nrurol.2012.111.


44. Hammerick KE, Longaker MT, Prinz FB. In vitro effects of direct current electric fields on adipose-derived stromal cells. Biochem Biophys Res Commun. 2010; 397:12–17. PMID: 20452327.


45. Li L, El-Hayek YH, Liu B, Chen Y, Gomez E, Wu X, et al. Direct-current electrical field guides neuronal stem/progenitor cell migration. Stem Cells. 2008; 26:2193–2200. PMID: 18556511.


46. Zhang J, Calafiore M, Zeng Q, Zhang X, Huang Y, Li RA, et al. Electrically guiding migration of human induced pluripotent stem cells. Stem Cell Rev. 2011; 7:987–996. PMID: 21373881.


47. Rando TA. The immortal strand hypothesis: segregation and reconstruction. Cell. 2007; 129:1239–1243. PMID: 17604710.


48. Jack GS, Zhang R, Lee M, Xu Y, Wu BM, Rodriguez LV. Urinary bladder smooth muscle engineered from adipose stem cells and a three dimensional synthetic composite. Biomaterials. 2009; 30:3259–3270. PMID: 19345408.


49. Shukla D, Box GN, Edwards RA, Tyson DR. Bone marrow stem cells for urologic tissue engineering. World J Urol. 2008; 26:341–349. PMID: 18654786.


50. Drost AC, Weng S, Feil G, Schafer J, Baumann S, Kanz L, et al. In vitro myogenic differentiation of human bone marrow-derived mesenchymal stem cells as a potential treatment for urethral sphincter muscle repair. Ann N Y Acad Sci. 2009; 1176:135–143. PMID: 19796241.


51. Anumanthan G, Makari JH, Honea L, Thomas JC, Wills ML, Bhowmick NA, et al. Directed differentiation of bone marrow derived mesenchymal stem cells into bladder urothelium. J Urol. 2008; 180(4 Suppl):1778–1783. PMID: 18721942.


52. Tian H, Bharadwaj S, Liu Y, Ma PX, Atala A, Zhang Y. Differentiation of human bone marrow mesenchymal stem cells into bladder cells: potential for urological tissue engineering. Tissue Eng Part A. 2010; 16:1769–1779. PMID: 20020816.


53. Owens GK, Kumar MS, Wamhoff BR. Molecular regulation of vascular smooth muscle cell differentiation in development and disease. Physiol Rev. 2004; 84:767–801. PMID: 15269336.


54. Zhang R, Jack GS, Rao N, Zuk P, Ignarro LJ, Wu B, et al. Nuclear fusion-independent smooth muscle differentiation of human adipose-derived stem cells induced by a smooth muscle environment. Stem Cells. 2012; 30:481–490. PMID: 22213158.


55. Alexanian AR, Kurpad SN. Quiescent neural cells regain multipotent stem cell characteristics influenced by adult neural stem cells in co-culture. Exp Neurol. 2005; 191:193–197. PMID: 15589526.


56. Schmidt C, Bladt F, Goedecke S, Brinkmann V, Zschiesche W, Sharpe M, et al. Scatter factor/hepatocyte growth factor is essential for liver development. Nature. 1995; 373:699–702. PMID: 7854452.


57. Kinnaird T, Stabile E, Burnett MS, Shou M, Lee CW, Barr S, et al. Local delivery of marrow-derived stromal cells augments collateral perfusion through paracrine mechanisms. Circulation. 2004; 109:1543–1549. PMID: 15023891.


58. Matsuda-Hashii Y, Takai K, Ohta H, Fujisaki H, Tokimasa S, Osugi Y, et al. Hepatocyte growth factor plays roles in the induction and autocrine maintenance of bone marrow stromal cell IL-11, SDF-1 alpha, and stem cell factor. Exp Hematol. 2004; 32:955–961. PMID: 15504551.
59. Nakamura T, Mizuno S. The discovery of hepatocyte growth factor (HGF) and its significance for cell biology, life sciences and clinical medicine. Proc Jpn Acad Ser B Phys Biol Sci. 2010; 86:588–610.


60. Spees JL, Olson SD, Whitney MJ, Prockop DJ. Mitochondrial transfer between cells can rescue aerobic respiration. Proc Natl Acad Sci U S A. 2006; 103:1283–1288. PMID: 16432190.


61. da Silva Meirelles L, Caplan AI, Nardi NB. In search of the in vivo identity of mesenchymal stem cells. Stem Cells. 2008; 26:2287–2299. PMID: 18566331.


62. Chung SY, Krivorov NP, Rausei V, Thomas L, Frantzen M, Landsittel D, et al. Bladder reconstitution with bone marrow derived stem cells seeded on small intestinal submucosa improves morphological and molecular composition. J Urol. 2005; 174:353–359. PMID: 15947689.


63. Frimberger D, Morales N, Shamblott M, Gearhart JD, Gearhart JP, Lakshmanan Y. Human embryoid body-derived stem cells in bladder regeneration using rodent model. Urology. 2005; 65:827–832. PMID: 15833555.


64. Zhang Y, Lin HK, Frimberger D, Epstein RB, Kropp BP. Growth of bone marrow stromal cells on small intestinal submucosa: an alternative cell source for tissue engineered bladder. BJU Int. 2005; 96:1120–1125. PMID: 16225540.


65. Drewa T, Joachimiak R, Kaznica A, Sarafian V, Pokrywczynska M. Hair stem cells for bladder regeneration in rats: preliminary results. Transplant Proc. 2009; 41:4345–4351. PMID: 20005396.


66. Zhu WD, Xu YM, Feng C, Fu Q, Song LJ, Cui L. Bladder reconstruction with adipose-derived stem cell-seeded bladder acellular matrix grafts improve morphology composition. World J Urol. 2010; 28:493–498. PMID: 20091038.


67. Sharma AK, Hota PV, Matoka DJ, Fuller NJ, Jandali D, Thaker H, et al. Urinary bladder smooth muscle regeneration utilizing bone marrow derived mesenchymal stem cell seeded elastomeric poly(1,8-octanediol-co-citrate) based thin films. Biomaterials. 2010; 31:6207–6217. PMID: 20488535.


68. Tian H, Bharadwaj S, Liu Y, Ma H, Ma PX, Atala A, et al. Myogenic differentiation of human bone marrow mesenchymal stem cells on a 3D nano fibrous scaffold for bladder tissue engineering. Biomaterials. 2010; 31:870–877. PMID: 19853294.


69. Sakuma T, Matsumoto T, Kano K, Fukuda N, Obinata D, Yamaguchi K, et al. Mature, adipocyte derived, dedifferentiated fat cells can differentiate into smooth muscle-like cells and contribute to bladder tissue regeneration. J Urol. 2009; 182:355–365. PMID: 19457498.


70. Lai JY, Yoon CY, Yoo JJ, Wulf T, Atala A. Phenotypic and functional characterization of in vivo tissue engineered smooth muscle from normal and pathological bladders. J Urol. 2002; 168(4 Pt 2):1853–1857. PMID: 12352375.


71. Atala A, Bauer SB, Soker S, Yoo JJ, Retik AB. Tissue-engineered autologous bladders for patients needing cystoplasty. Lancet. 2006; 367:1241–1246. PMID: 16631879.


72. Subramaniam R, Hinley J, Stahlschmidt J, Southgate J. Tissue engineering potential of urothelial cells from diseased bladders. J Urol. 2011; 186:2014–2020. PMID: 21944117.


73. Feil G, Christ-Adler M, Maurer S, Corvin S, Rennekampff HO, Krug J, et al. Investigations of urothelial cells seeded on commercially available small intestine submucosa. Eur Urol. 2006; 50:1330–1337. PMID: 16820260.


74. Sharma AK, Bury MI, Marks AJ, Fuller NJ, Meisner JW, Tapaskar N, et al. A nonhuman primate model for urinary bladder regeneration using autologous sources of bone marrow-derived mesenchymal stem cells. Stem Cells. 2011; 29:241–250. PMID: 21732482.


75. Valiunas V, Doronin S, Valiuniene L, Potapova I, Zuckerman J, Walcott B, et al. Human mesenchymal stem cells make cardiac connexins and form functional gap junctions. J Physiol. 2004; 555(Pt 3):617–626. PMID: 14766937.


76. Elbadawi A, Yalla SV, Resnick NM. Structural basis of geriatric voiding dysfunction. IV. Bladder outlet obstruction. J Urol. 1993; 150(5 Pt 2):1681–1695. PMID: 8411456.


77. Steers WD, De Groat WC. Effect of bladder outlet obstruction on micturition reflex pathways in the rat. J Urol. 1988; 140:864–871. PMID: 3418824.


78. Baskin LS, Sutherland RS, Thomson AA, Hayward SW, Cunha GR. Growth factors and receptors in bladder development and obstruction. Lab Invest. 1996; 75:157–166. PMID: 8765316.
79. Ghafar MA, Anastasiadis AG, Olsson LE, Chichester P, Kaplan SA, Buttyan R, et al. Hypoxia and an angiogenic response in the partially obstructed rat bladder. Lab Invest. 2002; 82:903–909. PMID: 12118092.


80. Levin RM, O'Connor LJ, Leggett RE, Whitbeck C, Chichester P. Focal hypoxia of the obstructed rabbit bladder wall correlates with intermediate decompensation. Neurourol Urodyn. 2003; 22:156–163. PMID: 12579634.


81. Nishijima S, Sugaya K, Miyazato M, Kadekawa K, Oshiro Y, Uchida A, et al. Restoration of bladder contraction by bone marrow transplantation in rats with underactive bladder. Biomed Res. 2007; 28:275–280. PMID: 18000341.


82. Huang YC, Shindel AW, Ning H, Lin G, Harraz AM, Wang G, et al. Adipose derived stem cells ameliorate hyperlipidemia associated detrusor overactivity in a rat model. J Urol. 2010; 183:1232–1240. PMID: 20096880.


83. Tanaka ST, Martinez-Ferrer M, Makari JH, Wills ML, Thomas JC, Adams MC, et al. Recruitment of bone marrow derived cells to the bladder after bladder outlet obstruction. J Urol. 2009; 182(4 Suppl):1769–1774. PMID: 19692058.


84. Song M, Heo J, Chun JY, Bae HS, Kang JW, Kang H, et al. The paracrine effects of mesenchymal stem cells stimulate the regeneration capacity of endogenous stem cells in the repair of a bladder-outlet-obstruction-induced overactive bladder. Stem Cells Dev. 2014; 23:654–663. PMID: 24192209.


85. Kucia M, Reca R, Campbell FR, Zuba-Surma E, Majka M, Ratajczak J, et al. A population of very small embryonic-like (VSEL) CXCR4(+)SSEA-1(+)Oct-4+ stem cells identified in adult bone marrow. Leukemia. 2006; 20:857–869. PMID: 16498386.


86. Ratajczak MZ, Machalinski B, Wojakowski W, Ratajczak J, Kucia M. A hypothesis for an embryonic origin of pluripotent Oct-4(+) stem cells in adult bone marrow and other tissues. Leukemia. 2007; 21:860–867. PMID: 17344915.


87. Dissaranan C, Cruz MA, Couri BM, Goldman HB, Damaser MS. Stem cell therapy for incontinence: where are we now? What is the realistic potential? Curr Urol Rep. 2011; 12:336–344. PMID: 21842258.


88. Chen S, Zhang HY, Zhang N, Li WH, Shan H, Liu K, et al. Treatment for chronic ischaemia-induced bladder detrusor dysfunction using bone marrow mesenchymal stem cells: an experimental study. Int J Mol Med. 2012; 29:416–422. PMID: 22109789.


89. Azadzoi KM. Effect of chronic ischemia on bladder structure and function. Adv Exp Med Biol. 2003; 539(Pt A):271–280. PMID: 15088910.


90. Abrams P, Andersson KE. Muscarinic receptor antagonists for overactive bladder. BJU Int. 2007; 100:987–1006. PMID: 17922784.


91. Azadzoi KM, Tarcan T, Kozlowski R, Krane RJ, Siroky MB. Overactivity and structural changes in the chronically ischemic bladder. J Urol. 1999; 162:1768–1778. PMID: 10524933.


92. Zhang H, Qiu X, Shindel AW, Ning H, Ferretti L, Jin X, et al. Adipose tissue-derived stem cells ameliorate diabetic bladder dysfunction in a type II diabetic rat model. Stem Cells Dev. 2012; 21:1391–1400. PMID: 22008016.


93. Lee JY, Piao S, Kim IG, Byun SS, Hwang JH, Hong SH, et al. Effect of human muscle-derived stem cells on cryoinjured mouse bladder contractility. Urology. 2012; 80:224.e7–224.e11. PMID: 22748891.


94. Christ GJ, Rehman J, Day N, Salkoff L, Valcic M, Melman A, et al. Intracorporal injection of hSlo cDNA in rats produces physiologically relevant alterations in penile function. Am J Physiol. 1998; 275(2 Pt 2):H600–H608. PMID: 9683449.
95. Hellweg R, Hartung HD. Endogenous levels of nerve growth factor (NGF) are altered in experimental diabetes mellitus: a possible role for NGF in the pathogenesis of diabetic neuropathy. J Neurosci Res. 1990; 26:258–267. PMID: 2142224.


96. Morris BD Jr, Drazan KE, Csete ME, Werthman PE, Van Bree MP, Rosenthal JT, et al. Adenoviral-mediated gene transfer to bladder in vivo. J Urol. 1994; 152(2 Pt 1):506–509. PMID: 8015103.


97. Goins WF, Lee KA, Cavalcoli JD, O'Malley ME, DeKosky ST, Fink DJ, et al. Herpes simplex virus type 1 vector-mediated expression of nerve growth factor protects dorsal root ganglion neurons from peroxide toxicity. J Virol. 1999; 73:519–532. PMID: 9847358.


98. Raivich G, Makwana M. The making of successful axonal regeneration: genes, molecules and signal transduction pathways. Brain Res Rev. 2007; 53:287–311. PMID: 17079020.


99. Tremp M, Salemi S, Largo R, Andersson KE, APlock J, Aboushwareb T, et al. Adipose-derived stem cells (ADSCs) and muscle precursor cells (MPCs) for the treatment of bladder voiding dysfunction. World J Urol. 2013; 11. 12. [Epub]. http://dx.doi.org/10.1007/s00345-013-1200-6.


100. Dayanc M, Kibar Y, Ural AU, Onguru O, Yildiz O, Irkilata HC, et al. The histopathologic, pharmacologic and urodynamic results of mesenchymal stem cell's injection into the decompensated rabbit's bladder. Stem Cell Rev. 2012; 8:1245–1253. PMID: 22736388.


TABLE 1
Studies with stem cell therapy in BOO models
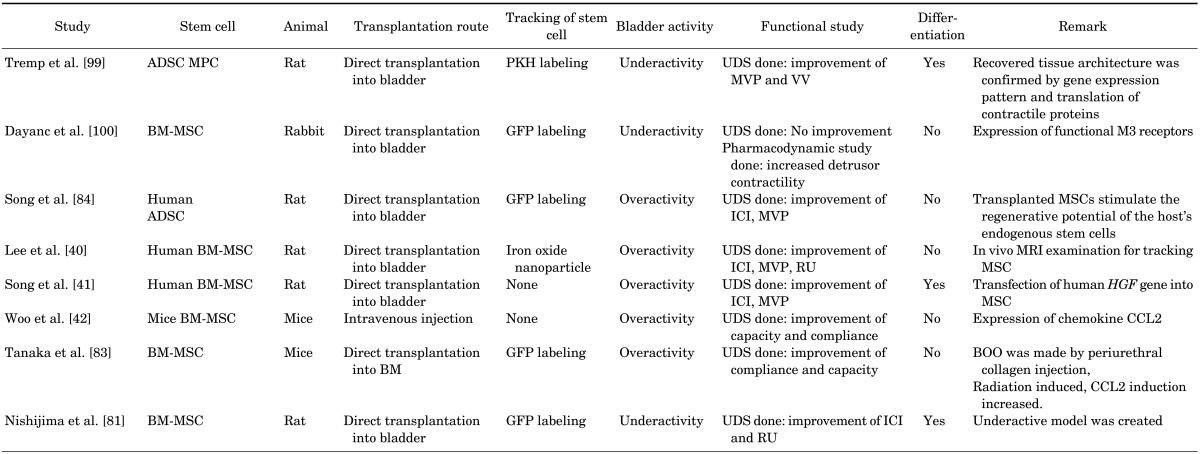
BOO, bladder outlet obstruction; ADSC, adipose derived stem cell; MPC, muscle derived stem cell; PKH, Paul Karl Horan; UDS, urodynamic study; MVP; maximal voiding pressure; VV, voided volume; BM-MSC, bone marrow-derived stem cell; GFP, green fluorescent protein; ICI inter-contraction interval; MSC, mesenchymal stem cell; RU, residual urine.