Abstract
Purpose
In this retrospective study, we analyzed the outcomes of prostate cancer patients treated with the CyberKnife radiotherapy system (Accuray).
Materials and Methods
Between 2007 and 2010, 31 patients were treated for prostate cancer by use of the CyberKnife radiotherapy system. After excluding six patients who were lost to follow-up, data for the remaining 25 patients were analyzed. Patients were divided into the CyberKnife monotherapy group and a postexternal beam radiotherapy boost group. Clinicopathologic features and treatment outcomes were compared between the groups. The primary endpoint was biochemical recurrence-free survival period based on the Phoenix definition. Toxicities were evaluated by using the Radiation Therapy Oncology Group scoring criteria.
Results
Of 25 patients, 17 (68%) and 8 (32%) were classified in the monotherapy and boost groups, respectively. With a median follow-up of 29.3 months, most of the toxicities were grade 1 or 2 except for one patient in the boost group who experienced late grade 3 gastrointestinal toxicity. The overall biochemical recurrence rate was 20% (5/25) and the median time to biochemical recurrence was 51.9 months. None of the patients with low or intermediate risk had experienced biochemical recurrence during follow-up. Among D'Amico high-risk populations, 16.7% (1/6) in the monotherapy group and 50.0% (4/8) in the boost group experienced biochemical recurrence.
Conclusions
Our data support that prostate cancer treatment by use of the CyberKnife radiotherapy system is feasible. The procedure can be a viable option for managing prostate cancer either in a monotherapy setting or as a boost after conventional radiotherapy regardless of the patient's risk stratification.
The number of prostate cancer (PCa) patients is rising annually in Korea owing to the aging of society, adoption of a westernized lifestyle, and implementation of the prostate-specific antigen (PSA) screening test. In 2005, the incidence of PCa was reported to be 4.5%, and a more recent investigation updated this figure to 7.6%, making PCa the fifth most common cancer among Korean men [1]. As the prevalence of PCa increases, various treatment modalities are considered. Radical prostatectomy is considered as the definitive treatment option for localized PCa. However, it is limited to patients who are at minimal risk for complications of general anesthesia and pelvic operation. For high surgical risk candidates, radiotherapy (RT) is a good alternative. In the past, RT was regarded as less effective than prostatectomy; however, efforts have been made to limit the high dose volume to the prostate while increasing the dose within that volume to improve local control of RT for PCa [2]. Along these lines, newer radiation treatment techniques such as three-dimensional conformal RT and intensity-modulated radiation therapy have been developed and show potentially better oncologic and safety outcomes than conventional RT [3-6].
Based on the idea that large radiation fraction sizes are radio-biologically favorable over small fraction sizes in treating PCa, hypofractionation with brachytherapy using high dose rate (HDR) brachytherapy showed promise as both a monotherapy and to boost external beam radiation therapy (EBRT) [2,7]. More recently, the use of the CyberKnife RT system (Accuray, Sunnyvale, CA, USA) has been reported to further improve patient tolerance in comparison with HDR brachytherapy. Early results with the CyberKnife system have shown acceptable PSA responses and low toxicities; however, the data are still insufficient [8-11]. As a follow-up to a previous study from 2009, we report the follow-up outcomes of patients with PCa treated by use of CyberKnife RT as a monotherapy and as a post-EBRT boost [12].
Between July 2007 and March 2010, 31 patients had undergone CyberKnife RT for localized or locally advanced non-metastatic PCa at our institution. Six patients were lost to follow-up, and the data for the remaining 25 patients were retrospectively reviewed. Of 25 patients, 17 (5 low, 6 intermediate, 6 high D'Amico risk) were treated with CyberKnife RT alone by use of dose/fractionation of 7.5-8.5 Gy×4-5 fractions (monotherapy group). The remaining 8 patients in the high-risk group received CyberKnife boost therapy with 6.0-8.0 Gy×3 fractions after conventional EBRT with a total radiation dose of 40 Gy in 20 fractions (boost group). The interval between EBRT and CyberKnife RT in the boost group was 7 to 10 days. Data were collected for previous history, results of a physical examination including digital rectal examination, and results of laboratory tests including serum PSA. Each of the patients underwent a transrectal ultrasound-guided prostate biopsy to confirm the pathologic diagnosis. The Gleason grading system was used to classify the differentiation of the cancer. Prostate magnetic resonance imaging (MRI) and whole-body bone scan were done for the staging work up, and each case was classified according to clinical staging guidelines suggested by the American Joint Cancer Committee (AJCC). The D'Amico risk classification was used to define risk groups.
Three or four gold fiducial pins were placed under the guidance of transrectal prostate ultrasonography before treatment planning. Planning imaging with computed tomography (CT) and MRI was performed 7 to 10 days after fiducial placement to allow the fiducial pins to be stabilized. The gold fiducial pins were 1 mm in diameter and 5 mm in length. The distance between the gold fiducial pins was 20 mm, and the angle between the gold fiducial pins was more than 15 degrees. The prostate gland with or without the seminal vesicle (SV) was carefully delineated by using CT-MRI fusion. The delineation and extent of SV involvement included in the clinical target volume (CTV) was determined on a case-by-case basis depending on the clinical T stage and risk of SV involvement. The planning target volume equaled the CTV expanded 2 mm posteriorly and 5 mm in all other dimensions. The gold fiducial pins were corrected by use of a real-time 3-LED camera fixed at the ceiling by the recognition of the movement and the target reposition during the treatment. The coordinate system allowed an average error of less than 0.7 mm and treatment accuracy of less than 0.3 mm. The CyberKnife system comprised a computerized robot arm with a 6 MV linear accelerator perpendicular to an x-ray system to track the target organ in real time and allowed effective stereotactic body radiation therapy. The median total radiation dose was 34.0 Gy (range, 32.0-37.5 Gy) divided into 4 to 5 fractions in the monotherapy group and 18.0 Gy (range, 18.0-24.0 Gy) divided into 3 to 5 fractions in the boost group, respectively. The treatment required about 90 minutes for each session.
Serum PSA was checked before the treatment, the first and second month after the CyberKnife RT, every 3 months until 24 months, and every 6 months thereafter. Biochemical recurrence (BCR) was defined as an increase of at least 2 ng/mL from the nadir PSA (Phoenix criteria). Acute toxicity was defined as events presented and resolved within 6 months after completion of CyberKnife RT by use of the Radiation Therapy Oncology Group (RTOG) scoring criteria. Complications relevant to CyberKnife RT after 6 months were considered as late toxicities [13].
Clinicopathologic features were compared between the monotherapy and boost groups. For the comparison, the Mann-Whitney U test and Kruskal-Wallis test were used for continuous variables, and the chi-square test was used for categorical variables. The Kaplan-Meier method was used to estimate the BCR-free survival, and survival curves were compared by using the log-rank test. All statistical procedures were performed by using PASW ver. 18.0 (SPSS Inc., Chicago, IL, USA). A p-value <0.05 was considered significant, and all p-values were two-sided.
The mean age of the total cohort was 71.12±7.64 years with an average follow-up duration of 29.3 months (interquartile range, 16.8-50.4 months). Baseline median PSA was 10.95 ng/mL. There were 5 (20.0%), 6 (24.0%), and 14 patients (56.0%) in the low, intermediate, and high D'Amico risk groups, respectively.
The clinicopathologic and treatment characteristics of the patient cohort are summarized in Table 1. There were no significant differences in median age or performance status between the groups. In the monotherapy cohort, 83.3% (5/6) and 100% of the patients (6/6) in the intermediate and high-risk groups, respectively, received androgen deprivation therapy (ADT) before CyberKnife RT. None of the patients in the low-risk group received ADT. In the boost cohort, 87.5% of the patients (7/8) received ADT. All the patients in this group were D'Amico high risk and 50% (4/8) had a Gleason score of 9.
Among the whole study population, the overall BCR rate was 20% (5/25). None of the patients in the low- or intermediate-risk groups had reached BCR during follow-up. Among D'Amico high-risk populations, however, 16.7% (1/6) in the monotherapy group and 50.0% (4/8) in the boost group had experienced BCR. Of the patients who had reached BCR, one patient (20.0%) in the boost group had undergone salvage radical prostatectomy. The other four (80%) required adjuvant ADT between 8 and 60 months after the initiation of CyberKnife RT.
The median time to BCR of the whole cohort was 51.9 months (Fig. 1). For D'Amico high-risk patients, the median time to BCR was 51.9 and 45.7 months in the monotherapy and boost groups, respectively. There was a significant difference in BCR between the two groups (log rank=0.018).
Five patients (29.4%) in the monotherapy group and four patients (50.0%) in the boost group experienced complications after CyberKnife RT (Table 2). In the monotherapy group, four patients showed acute genitourinary toxicities including frequency, nocturia, and urgency and one patient had late gastrointestinal toxicity. All toxicities were RTOG grade I and were managed conservatively. Patients in the boost group reported similar toxicities; however, one patient complained of hematochezia and bowel habit change, which was diagnosed as radiation proctitis. Eventually, it was surgically managed. Late toxicities (4/4) were reported only in the D'Amico high-risk group.
RT is defined as irradiation of a given target organ with high-dose ionizing radiation in mono- or hypo-fractionated treatment. When RT is performed, the radiation beam should accurately irradiate the target organ according to its size, shape, and location. At the same time, dose distribution should be highly conformal and cause minimal cellular damage to adjacent organs [14]. Ideally, the fractionation schedule of RT should match the fractionation sensitivity of the tumor relative to nearby normal tissues. The biologically effective dose (BED) is expressed as BED=D[1+d/(α/β)], where D refers to the total dose, d to the dose per fraction, and α/β to the sensitivity of the target organ to dose fraction size [15]. Radio-biologically, the α/β ratio of PCa tissue is 1.5; that of early-responding tissues (e.g., skin, mucosa, and most tumors) is 10; and that of late-responding tissues (e.g., connective tissue, bladder/rectal mucosa, and muscle) is 3 [16-18]. With the accumulation of evidence for such a low α/β ratio for PCa, hypofractionated RT is emerging as an effective and safe alternative option for treatment. The BED of conventional RT (74 Gy at 2 Gy per fraction for 37 fractions) of PCa is 172.6 Gy: 88.8 Gy in early-responding tissue and 123.3 Gy in late-responding tissue. However, the BED of hypofractionated RT (34 Gy at 8.5 Gy per fraction for 4 fractions) of PCa is 226 Gy: 62.9 Gy in early responding tissue and 130.3 Gy in late responding tissue [19]. In other words, hypofractionated RT enables high-dose radiation of the target organ with minimal radiation to normal tissue on the condition that precise radiation is possible.
Hypofractionation with brachytherapy using HDR brachytherapy has already shown excellent efficacy and safety profiles as both monotherapy and as a post-EBRT boost [20,21]. Despite such excellent results, HDR brachytherapy has not been widely adopted because of its relatively invasive nature, need for anesthesia, and technical difficulties. Adaptation of CyberKnife RT to replicate HDR brachytherapy dosimetry in treating localized PCa was applied by investigators to overcome the disadvantages of HDR brachytherapy [22].
Early results of CyberKnife RT for localized PCa have been reported by other researchers. King et al. [15] reported that CyberKnife RT was feasible in PCa, compared with other types of cancer, and Friedland et al. [10] suggested hypofractionated CyberKnife monotherapy as an emerging modality.
In our study, 80% of patients (20/25) remained free of BCR during follow-up. In the monotherapy group, none of the patients with low to intermediate risk encountered BCR, whereas 16.7% of the high-risk patients (1/6) showed BCR 51.9 months after the CyberKnife therapy. Other studies in the treatment of PCa with CyberKnife RT reported similar oncological results in low- or intermediate-risk groups. Freeman and King [9] reported BCR-free survival of 92.7% at a median of 60 months of follow-up, and among 41 low-risk PCa patients, only 3 patients had developed BCR at 33, 37, and 42 months. Katz et al. [23] reported 97.6% of BCRFS among low- and intermediate-risk PCa patients. The cohort study including high-risk PCa patients by Kang et al. [24] reported 100% of BCR-free survival at a median follow-up of 40 months.
Not many studies have reported treatment outcomes of CyberKnife RT as a post-EBRT boost. Preliminary data on the efficacy and safety of post-EBRT CyberKnife boost compared with HDR brachytherapy boost were introduced by Oermann et al. [2]. Recently, Jabbari et al. [25] reported that PSA response defined by nadir PSA was acceptable compared with the HDR brachytherapy boost group (median nadir PSA, 0.10 ng/mL vs. 0.09 ng/mL). However, these studies had relatively short follow-up periods from which to draw conclusions about the PSA response. Half of the patients in our study encountered BCR with a median time to BCR of 45.7 months, but it obvious to expect poorer PSA responses in such a high-risk group. In our study population, it would not be reasonable to compare the PSA response between the monotherapy and boost groups in the high-risk patients because the clinicopathologic features of the patients were more unfavorable in the boost group.
Because of the anatomical position of the prostate, adjacent to the rectum, bladder, and urethra, genitourinary and gastrointestinal toxicities are commonly reported for RT. In our study, 36% of patients (9/25) experienced RT-induced toxicity. Of the 9 patients, 8 complained of RTOG I-II genitourinary or gastrointestinal toxicity. Gastrointestinal toxicities were shown only in high-risk patients, and patients in the boost group tend to have higher grade toxicity than did patients in the monotherapy group. Including SV in the CTV may have affected the higher incidence of gastrointestinal toxicity. Most of the patients were managed conservatively, except for the patients in the boost group with radiation proctitis who underwent subsequent surgical treatment. The toxicity rate of our study was similar to that of previous studies. In other studies, grade I-II and grade III acute rectal toxicity rates were 9% to 80% and 10%, respectively [26]. Late grade I-II rectal toxicity was 0% to 48%, and grade III toxicity occurred in only 1% to 5% [10,27]. Several studies have reported late grade III proctitis, which required argon plasma laser ablation in certain cases. Concerning genitourinary toxicity, 13.5% to 78% of acute grade I-II toxicity was reported in phase I or II clinical trials; 5% of grade III toxicity in only one study [16]. Townsend et al. [28] reported an incidence of acute grade I-II toxicity of 64%, which was mostly frequency/nocturia or dysuria owing to epididymitis after fiducial placement. Only 8% of patients experienced acute grade III urinary toxicity. No grade IV acute or late urinary toxicities were reported [27].
Our study has shown the feasibility of CyberKnife RT as a treatment of PCa regardless of the risk stratification. In most studies, only low- to intermediate-risk patients had been treated with CyberKnife RT. In our series however, 52% of the cohort was classified as having high-risk PCa, and the oncologic results and complication rate of this group were comparable with those of other treatment modalities. Of the five patients who experienced BCR, four patients were managed nonoperatively with ADT, and their PSA remained low throughout the review period (under 0.5 ng/mL). The one remaining patient with BCR underwent salvage radical prostatectomy.
Radical prostatectomy would be the standard treatment option in patients with good performance status and long life expectancy; however, choosing the optimal treatment plan for patients with high surgical risk, owing to age or other comorbidities, is always a concern. For the latter group of patients, CyberKnife RT is a viable alternative on the basis of recent findings regarding its oncologic and toxicity results. Compared with conventional RT, the shorter treatment session is one of the advantages of CyberKnife RT, because it provides incentives for patients to become more compliant. Despite such advantages, the high cost remains the main obstacle to the widespread use of CyberKnife RT in Korea for now.
There were some limitations to our study. First, the statistical significance of the study could not be ascertained because of the small sample size. Second, owing to the retrospective design of the study, there was no strict protocol for patient enrollment. The study outcomes cannot be solely attributed to CyberKnife therapy because patients were treated with other treatment modalities such as ADT before and after the CyberKnife RT.
Considering the oncological and toxicity outcomes, CyberKnife RT is a feasible treatment modality in the management of PCa. On the basis of our experience, CyberKnife RT can be a viable option for managing PCa either in a monotherapy setting or as a boost after EBRT regardless of the risk stratifications. Larger, prospectively designed randomized trials are needed before the outcomes of this study can be generalized in the management of PCa.
Figures and Tables
FIG. 1
Kaplan-Meier curves for biochemical recurrence (BCR) free survival based on Phoenix definition. (A) All cohort, (B) comparison of monotherapy and postexternal beam radiation therapy boost group. CI, confidence interval; EBRT, external beam radiation therapy; NA, not available.
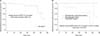
TABLE 1
Patient and treatment characteristics in the CyberKnife monotherapy and post-EBRT boost groups (based on D'Amico risk classification)
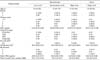
Values are presented as median (range) or number (%) unless otherwise indicated.
EBRT, external beam radiation therapy; ECOG, Eastern Cooperative Oncology Group; PSA, prostate-specific antigen; CK, CyberKnife; ADT, androgen deprivation therapy; Rx, radiation; IQR, interquartile range.
a:p<0.05 using chi-square test. b:p<0.05 using Kruskal-Wallis test.
References
1. Jung KW, Won YJ, Kong HJ, Oh CM, Seo HG, Lee JS. Cancer statistics in Korea: incidence, mortality, survival and prevalence in 2010. Cancer Res Treat. 2013; 45:1–14.
2. Oermann EK, Slack RS, Hanscom HN, Lei S, Suy S, Park HU, et al. A pilot study of intensity modulated radiation therapy with hypofractionated stereotactic body radiation therapy (SBRT) boost in the treatment of intermediate- to high-risk prostate cancer. Technol Cancer Res Treat. 2010; 9:453–462.
3. Smart RF. Radical radiotherapy for carcinoma of the prostate. N Z Med J. 1979; 90:285–287.
4. Kim BK, Park SW, Ha SW. Pattern of decrease of prostate specific antigen after radical radiotherapy for the prostate cancer. J Korean Soc Ther Radiol Oncol. 1999; 17:136–140.
5. Chin OH, Kim SI, Hong SJ. Early experience of localized prostate cancer treated with neoadjuvant androgen ablation therapy and radiotherapy. Korean J Urol. 2001; 42:702–706.
6. Kuban DA, Levy LB, Cheung MR, Lee AK, Choi S, Frank S, et al. Long-term failure patterns and survival in a randomized dose-escalation trial for prostate cancer. Who dies of disease? Int J Radiat Oncol Biol Phys. 2011; 79:1310–1317.
7. Fowler JF. The radiobiology of prostate cancer including new aspects of fractionated radiotherapy. Acta Oncol. 2005; 44:265–276.
8. Kim GH, Park K, Jo MK, Lee CW, Yang KM, Cho CG. CyberKnife for the treatment of nonmetastatic prostate cancer: preliminary results. Korean J Urol. 2006; 47:1172–1177.
9. Freeman DE, King CR. Stereotactic body radiotherapy for low-risk prostate cancer: five-year outcomes. Radiat Oncol. 2011; 6:3.
10. Friedland JL, Freeman DE, Masterson-McGary ME, Spellberg DM. Stereotactic body radiotherapy: an emerging treatment approach for localized prostate cancer. Technol Cancer Res Treat. 2009; 8:387–392.
11. Ju AW, Wang H, Oermann EK, Sherer BA, Uhm S, Chen VJ, et al. Hypofractionated stereotactic body radiation therapy as monotherapy for intermediate-risk prostate cancer. Radiat Oncol. 2013; 8:30.
12. Hyun JH, Park BH, Koo DY, Kim KS, Song KH, Jeong WK, et al. Early experience of prostate cancer treated with cyberknife radiotherapy. Korean J Urol. 2009; 50:1066–1072.
13. Lawton CA, Won M, Pilepich MV, Asbell SO, Shipley WU, Hanks GE, et al. Long-term treatment sequelae following external beam irradiation for adenocarcinoma of the prostate: analysis of RTOG studies 7506 and 7706. Int J Radiat Oncol Biol Phys. 1991; 21:935–939.
14. Coste-Maniere E, Olender D, Kilby W, Schulz RA. Robotic whole body stereotactic radiosurgery: clinical advantages of the Cyberknife integrated system. Int J Med Robot. 2005; 1:28–39.
15. King CR, Lehmann J, Adler JR, Hai J. CyberKnife radiotherapy for localized prostate cancer: rationale and technical feasibility. Technol Cancer Res Treat. 2003; 2:25–30.
16. King CR, Brooks JD, Gill H, Pawlicki T, Cotrutz C, Presti JC Jr. Stereotactic body radiotherapy for localized prostate cancer: interim results of a prospective phase II clinical trial. Int J Radiat Oncol Biol Phys. 2009; 73:1043–1048.
17. Kal HB, Van Gellekom MP. How low is the alpha/beta ratio for prostate cancer? Int J Radiat Oncol Biol Phys. 2003; 57:1116–1121.
18. Wang JZ, Li XA, Yu CX, DiBiase SJ. The low alpha/beta ratio for prostate cancer: what does the clinical outcome of HDR brachytherapy tell us? Int J Radiat Oncol Biol Phys. 2003; 57:1101–1108.
19. Duchesne GM, Peters LJ. What is the alpha/beta ratio for prostate cancer? Rationale for hypofractionated high-dose-rate brachytherapy. Int J Radiat Oncol Biol Phys. 1999; 44:747–748.
20. Hoskin PJ, Motohashi K, Bownes P, Bryant L, Ostler P. High dose rate brachytherapy in combination with external beam radiotherapy in the radical treatment of prostate cancer: initial results of a randomised phase three trial. Radiother Oncol. 2007; 84:114–120.
21. Pieters BR, de Back DZ, Koning CC, Zwinderman AH. Comparison of three radiotherapy modalities on biochemical control and overall survival for the treatment of prostate cancer: a systematic review. Radiother Oncol. 2009; 93:168–173.
22. Fuller DB, Naitoh J, Lee C, Hardy S, Jin H. Virtual HDR CyberKnife treatment for localized prostatic carcinoma: dosimetry comparison with HDR brachytherapy and preliminary clinical observations. Int J Radiat Oncol Biol Phys. 2008; 70:1588–1597.
23. Katz AJ, Santoro M, Ashley R, Diblasio F. Stereotactic body radiation therapy for low- and low-intermediate-risk prostate cancer: Is there a dose effect? Front Oncol. 2011; 1:49.
24. Kang JK, Cho CK, Choi CW, Yoo S, Kim MS, Yang K, et al. Image-guided stereotactic body radiation therapy for localized prostate cancer. Tumori. 2011; 97:43–48.
25. Jabbari S, Weinberg VK, Kaprealian T, Hsu IC, Ma L, Chuang C, et al. Stereotactic body radiotherapy as monotherapy or post-external beam radiotherapy boost for prostate cancer: technique, early toxicity, and PSA response. Int J Radiat Oncol Biol Phys. 2012; 82:228–234.
26. Aluwini S, van Rooij P, Hoogeman M, Bangma C, Kirkels WJ, Incrocci L, et al. CyberKnife stereotactic radiotherapy as monotherapy for low- to intermediate-stage prostate cancer: early experience, feasibility, and tolerance. J Endourol. 2010; 24:865–869.
27. McBride SM, Wong DS, Dombrowski JJ, Harkins B, Tapella P, Hanscom HN, et al. Hypofractionated stereotactic body radiotherapy in low-risk prostate adenocarcinoma: preliminary results of a multi-institutional phase 1 feasibility trial. Cancer. 2012; 118:3681–3690.
28. Townsend NC, Huth BJ, Ding W, Garber B, Mooreville M, Arrigo S, et al. Acute toxicity after cyberknife-delivered hypofractionated radiotherapy for treatment of prostate cancer. Am J Clin Oncol. 2011; 34:6–10.