Abstract
Purpose
Understanding of prostate anatomy has evolved as techniques have been refined and improved for radical prostatectomy (RP), particularly regarding the importance of the neurovascular bundles for erectile function. The objectives of this study were to develop inexpensive and simple but anatomically accurate prostate models not involving human or animal elements to teach the terminology and practical aspects of nerve-sparing RP and simple prostatectomy (SP).
Materials and Methods
The RP model used a Foley catheter with ballistics gelatin in the balloon and mesh fabric (neurovascular bundles) and balloons (prostatic fascial layers) on either side for the practice of inter- and intrafascial techniques. The SP model required only a ripe clementine, for which the skin represented compressed normal prostate, the pulp represented benign tissue, and the pith mimicked fibrous adhesions. A modification with a balloon through the fruit center acted as a "urethra."
Results
Both models were easily created and successfully represented the principles of anatomical nerve-sparing RP and SP. Both models were tested in workshops by urologists and residents of differing levels with positive feedback.
Conclusions
Low-fidelity models for prostate anatomy demonstration and surgical practice are feasible. They are inexpensive and simple to construct. Importantly, these models can be used for education on the practical aspects of nerve-sparing RP and SP. The models will require further validation as educational and competency tools, but as we move to an era in which human donors and animal experiments become less ethical and more difficult to complete, so too will low-fidelity models become more attractive.
Prostate anatomy has become better understood in the past 20 years as techniques have been refined and improved for radical prostatectomy [1,2]. In particular, our understanding of the neurovascular bundles has become of paramount importance in striving to preserve erectile function in men undergoing surgery for prostate cancer [3-5]. With the increasingly complex surgical terminology and techniques, it is important that those learning nerve-sparing radical prostatectomy (NSRP) techniques understand the basic principles of surgical anatomy so that the new surgical terminology can be placed in context. For example, terms such as intrafascial, interfascial, and non-nerve-sparing surgery are all commonly used in the literature. Although videos and written descriptions may assist in learning such terms, ultimately, practicing surgery is the best facilitator of learning. Owing to various constraints upon training, however, this is not always possible or practical. It is therefore imperative that the urology community develop simple, low-cost models that can be used in informative and brief workshops to allow surgical trainees to study anatomical and surgical concepts before embarking on surgery.
Surgical skills laboratories have become important tools for educating and training surgeons. Although the end-points of improving patient outcome are yet to be fully defined, there are more conceptual areas of surgery that may benefit trainees provided integration between anatomy and surgical practice is maintained [6-8]. Furthermore, with newly enforced work hour restrictions, opportunities to acquire operative skills are limited, necessitating the development of alternative strategies of education [9]. This combined with the paucity of donated cadavers and the inherent ethical and cost issues in dealing with live and deceased animals in laboratories have forced educators to develop and consider creative workshops that require none of these elements [10-12].
From a urological point of view, a model for teaching prostate anatomy and placing into context the complex terminology such as that used in NSRP while also allowing the simulation of surgical maneuvers appears to be one of the most relevant models in need of development. This is also true for open simple prostatectomy, an operation that, although still required, is less common. Accordingly, an instructive model may improve conceptual understanding of such a procedure.
The objectives of this study were therefore to develop inexpensive and easily constructed but anatomically accurate models of the prostate that do not involve human or animal elements to teach the terminology and practical aspects of NSRP and simple prostatectomy.
A 22 Fr Foley urinary catheter (Teleflex Medical, Pilling Weck, Markham, Canada) with a 75 cc balloon, gelatin (Knox® Gelatine, Associated Brands, Mississauga, Canada), a piece of timber approximately 40×6 cm with two hook screws (4 cm), two standard party balloons, fabric mesh (or stockings), a heavy silk tie, hobby glue, water, and food dye (optional).
Ballistics gel has long been used in forensic medicine. We modified the standard version [13] to develop a texture that appropriately represented a prostate. Two 1.8 g sachets were added to 155 cc of room-temperature water in a plastic container and were slowly stirred so as to not allow accumulation of air bubbles, which weakens the structure of the gel. After the water was mixed for 30 s, it was cooled at 4℃ for at least 2 hours (preferably overnight). Next, hot water (50℃) was placed in a sink or repository so that the gel could be gently melted within its container, with stirring, while again being careful to not introduce air bubbles. Red food dye can be added (several drops as desired) at this stage for an even more life-like prostate. A 20 cc syringe was then used to aspirate the warm gelatin mixture and insert it via the catheter balloon channel of the Foley catheter into the balloon (50 cc for a 50 cc prostate and varied as required) in an inverted manner to avoid air bubble introduction, thus forming the prostate and its capsule (the actual catheter balloon).
We used balloons (fascia) and mesh fabric (the neurovascular bundles) to simulate the neurovascular bundles and prostatic fascial layers. First, a small hole (2 mm) was cut into the dome of one balloon and a squirt of glue (5 cc) was placed inside the neck of this first balloon. The balloon was then pulled over the catheter balloon after passing the distal or eye end of the Foley catheter through the hole in the balloon and was secured snugly over the catheter balloon as the first layer of prostatic fascia. The mesh fabric was then placed in a similar fashion over the first balloon after passing the distal or eye end of the Foley catheter through the mesh. Finally, glue was inserted into the neck of the second balloon (preferably a different color for later identification) and it was stretched over the fabric to form the outer fascial layer. The fabric mesh (neurovascular bundle) was thus between the two balloons (fascia). The balloons and mesh were stretched and secured with silk ligatures proximally and distally over the catheter to ensure that the shape was maintained as the glue dried. The glue formed gentle but dissectible adhesions as in real life. Excess balloon at the distal or eye end of the Foley catheter was trimmed if needed. The catheter with inflated balloon and fascial layers were placed in the refrigerator (4℃) overnight.
The catheter was removed from the refrigerator with the gel now set within the Foley catheter balloon with the texture of a prostate (Fig. 1). The screw hooks were placed one on the top of the timber and one on the bottom edge. The eye of the catheter was anchored on top of the wood and the "Y" of the injection port was hooked under the bottom side. The catheter was placed so that it could be "operated" on in the workshop or skills laboratory.
Standard instruments can be used to dissect the fascial layers in an inter- or intrafascial manner by use of surgical clips if desired. The neurovascular bundle is easily identified (the mesh) and the principles of high (veil) and low release can be explored. Incisions into the prostate are noted by perforations in the Foley catheter balloon.
One ripe clementine (mandarin), a foam (or thick card or cork) board 10×20 cm, heavy silk or Vicryl suture on a needle, and a party balloon (optional). The clementines should be relatively large to approximate a prostate with significant benign prostatic hyperplasia (BPH) (other citrus fruit that can be peeled may be substituted depending on the season). One party balloon and needle driver are also required.
The skin of the clementine acts as the compressed normal prostate tissue, as happens with significant benign prostatic hyperplasia adenoma formation (Fig. 2). The pulp (segments within) acts as the prostate tissue, and the white fibrous adhesions of the pith from fruit to skin mimic adhesions in humans. To make the model more anatomical, we pulled a balloon through the center of the fruit by its neck after scissors grasped by needle holders were passed through (Fig. 3). Once pulled through, the balloon was tied to the other side to fix it in place. This formed the "prostatic urethra" that must be deliberately incised at the time of prostatectomy.
The clementine (prostate) need only be fixed to the foam board by three to four sutures at the base, being careful to take the full thickness of the fruit skin. This is important because once the fruit pulp is liberated from the skin, these sutures will be the "bleeding vessels" at the base of the prostate that will need to be oversewn in the model (Fig. 3).
Standard instruments were used to dissect the skin (compressed normal prostate) away from the pulp (adenoma) after suturing the vessels in the fruit skin (thickened prostate). The pulp was carefully dissected by use of sharp and blunt techniques to separate the pith (adhesions), with sharp dissection formally cutting the balloon (urethra). At the base of the capsule, the fixing sutures of the model can be likened to bleeding vessels and can be oversewn. Vessels at the bladder neck can also be imitated if desired by using sutures as for the fixing ones. The skin (capsule) was then closed and a catheter (only one is needed that can be used by more than one group) can be passed through to demonstrate the end result. The pulp can be inspected for completeness of excision of the adenoma.
Both models were tested by urological trainees, fellows, and staff for their ability to outline the principles of NSRP and simple prostatectomy as part of their standard teaching. This testing was done as part of the official University of Toronto teaching sessions for urology residents, and all data collected were anonymous as per the teaching protocol.
At the end of each session, the participants were asked to fill out a typical five-level Likert scale-based questionnaire (e.g., strongly disagree, disagree, neither agree nor disagree, agree, and strongly agree when asked a question) detailing their experience with the models. The questionnaire was a standardized form used for all University of Toronto resident training sessions. Participants were then also debriefed one-on-one to ascertain the nature of the models and their usefulness in skills workshops. Any technical points could also be noted on the back of the survey.
This model was easily created and was successfully utilized to study the principles of NSRP in the open setting (Fig. 1). The model was also used in laparoscopic simulations. The key features of the model are that it provides the correct anatomy for a radical prostatectomy section and highlights the relationship between the neurovascular bundle, fascial layers, and prostatic capsule. In particular, the layers can be dissected and color-coded and the different facets of prostatectomy such as intra- versus interfascial nerve-sparing techniques can be demonstrated. Incisions into the capsule of the prostate are noted by appearance of the ballistics gel.
The average score was 4.7/5 for the NSRP and 4.5/5 for the simple prostatectomy models as judged for overall learning experience. Anatomy appeared to be reproduced in the case of both models to a high degree despite the low-fidelity nature of the tools. Importantly, maneuvers can be practiced with instruments used in real surgery. Thus, experience with handling of instruments and the principles of handling such instruments are an important byproduct of such a workshop. Some specific unexpected technical points were also able to be noted in this low-fidelity model. First, the ability to perform nerve-sparing correctly by not incising the capsule was evidenced by the gel being visible when the "capsule" (Foley catheter balloon) was inadvertently incised. Also, the concept of a high release ("veil") versus a low release of the neurovascular bundle appeared to be mastered. In the simple prostatectomy model, the challenge was to remove the pulp intact with as little juice spilled as possible, indicating minimal handling of the "adenoma" with the juice likened to blood loss. Preservation of the "urethra" in this model was also identified as an excellent teaching point.
Simulation of surgical procedures is considered to be a huge paradigm shift in surgical education and skills acquisition. Certainly, simulation is now accepted as a new concept not only for surgery but also for broader health education [14]. The driving force behind such a shift is a complex mix of shorter work hours with reduced training time, a greater number of trainees with a reduced patient workload for educational opportunities, a demand to acquire "proven" surgical skill before practicing on patients in a bid to reduce medical errors, and a shift to outcome-based education with its requirements for assessment and demonstration of competence [9,15]. These reasons have spurred the development of alternative strategies of education with surgical skills laboratories being a central focus. Certainly, skills laboratories can be used to demonstrate specific skills transferable to the operating room at their highest level (e.g., nephrectomy in a live porcine model), but they may also be used further "downstream": that is, to reaffirm basic principles of anatomy and steps of surgical procedures as well as to provide experience with the use of instruments that, although not designed to, may approach levels expected in competency testing. In other words, not every exercise in a skills laboratory need be done on human cadavers or live animal models to benefit training surgeons. We were able to create low-fidelity yet highly anatomically correct, inexpensive, and simple models that are useful for teaching urological surgery, with a focus more on the anatomy and procedural levels rather than the competency level. The models may be used for open, laparoscopic, or robotic-assisted radical and simple prostatectomy and may in the future be modified or tested so that they can become competency tools.
Evidence-based medicine is now entrenched in all specialties, including urology [16]. As such, overriding all of these new educational devices is the need for validation of the tools in a preliminary sense as not only educational devices but also for transference into real-life practice [17]. Both remain a challenge. In this manner, some models will be suitable for teaching anatomy and procedural steps, as our model appears to be, whereas others will be better for technical competency assessment and transferal; some will fall between. In a graduated way, low-fidelity models may assist with the initial experience of teaching procedures (e.g., robot-assisted radical prostatectomy) to surgeons-in-training by providing a forum for standardized learning, training, and evaluation as we move away from the master-apprentice educational system [18].
Surgical models were created in the early 1960s to establish the surgical skills of training surgeons for transurethral procedures [19] and are not likely to disappear soon [12,20]. Clearly, there will always be a place for cadaveric or animal models, but the current constraints on resources as well as ethical issues mean that low-fidelity models that are inexpensive and simple to construct are necessary [12,20,21]. It may be argued that surgical simulation may also fill the void in some instances, and even virtual reality is being proposed and investigated for use in a variety of urological procedures [22-24]. Although increasingly studied in urology, such educational tools do not allow the surgeon to hold real instruments and to practice on a cheap and easily made model that is tangible and anatomically correct. Many clinicians are questioning the validity of surgical skills laboratories that use and develop simulators and their true place in surgical education. For example, some have debated whether simulators are really just pseudo "video-games" or are really helpful [25]. The lines between the two may truly be blurred in laparoscopy. Some evidence suggests that indeed video games are helpful for surgical skill acquisition [26]. Even innovative ideas such as gaze training for laparoscopy rather than focusing on the instruments themselves has been suggested [27].
The final question to answer is whether models may also help to compensate for the lack of anatomy now taught at medical schools. Much has been written on the potential penalty of insufficient anatomy education at the undergraduate level, which may lead to incompetent anatomists and health care professionals, leaving patients to face dire repercussions [28]. Evidence suggests that textbooks and even in some cases contemporary multimedia tools have failed to keep pace with advances in surgical anatomy, which also delays educational flow to clinicians [1,2]. In our model, principles of anatomy are reinforced. When combined with appropriate diagrams and interactive lectures, the model certainly acts as an educational model. Models also need to be adaptable to have a place in open and laparoscopic (and robotic) surgery, because these techniques are progressing, and training the next generation of surgeons is essential [29,30].
The limitation of any low-fidelity model is that it does not exactly mimic the tissues encountered in surgery. Furthermore, there is no active physiology, so the tissues do not bleed. However, in the radical prostatectomy model, capsular incisions can be noted and discussed. Also, in the simple prostatectomy model, the ability to remove the pulp with minimal disruption (as evidenced by squeezed pulp) and evidence of citrus juice around the model provide some life-like responses. Furthermore, tissues may be torn, so there is some degree of passive tissue physiology. It may be possible to add pulsating bleeding vessels at low cost and with minimal equipment to such models, and we are working on this for future models.
Low-fidelity models are achievable in urology for the demonstration of prostate anatomy and for surgical practice and need not be expensive, cumbersome, or complex to construct. Importantly, these models can be utilized to study both the terminology and the practical aspects of NSRP and simple prostatectomy. The models will require further validation as educational and competency tools, but as we move to an era where human donors and animal experiments become less ethical and more difficult to complete, so too will low-fidelity models become more attractive.
Figures and Tables
FIG. 1
Ballistics gel radical prostatectomy neurovascular bundle dissection model. The model is complete and fixed to the wood (A). The diagram (B) demonstrates the layers of the prostate, its capsule, the fascias, and the neurovascular bundle. The results of the two techniques are demonstrated (C) with the interfascial dissection still having fascia (blue balloon indicated by yellow arrow) over the capsule yet the neurovascular bundle being pushed away. The intrafascial dissection has no fascia visible with the prostate capsule exposed (clear Foley balloon indicated by white arrow) and the neurovascular bundle completely pushed away between both fascial layers. The artwork in part B is published with the permission of Shelley L.W. Chen, University of Toronto, Biomedical Communications Department.
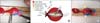
FIG. 2
The simple prostatectomy model using a clementine. The model in this iteration was not modified to be fixed on foam or cardboard and has no urethra, thus highlighting the simplest form it may take yet still be instructional. For a retropubic approach, hemostatic sutures are first applied to the capsule (A) before incision (B) of the capsule (skin). The pulp (adenoma) is then dissected off the capsule (C) and finally carefully removed from the capsule, preferably intact, with the capsule closed (skin) with sutures (D).
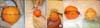
FIG. 3
The simple prostatectomy model using a clementine as modified to be fixed on foam or cardboard and to have a urethra. A party balloon is inserted into the centre of the fruit (A) as the urethra and is later tied to the foam board. The urethra can be stretched as in real cases (B) before being formally incised. The blue suture fixing the base of the prostate model to the foam board (A and C) acts as a bleeding vessel to oversew when the pulp (adenoma) has been removed (in this case the skin [capsule] is split to demonstrate fixation to the foam board).
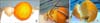
ACKNOWLEDGEMENT
We acknowledge Shelley L.W. Chen, University of Toronto, Biomedical Communications Department, for the permission to publish the artwork in Fig. 1.
References
1. Lawrentschuk N, Fleshner N. An interactive multimedia approach to urological anatomy. BJU Int. 2010. 105:904–906.
2. Lawrentschuk N, Lindner U, Fleshner N. Current textbooks and anatomy of the prostate--a case for an update. BJU Int. 2009. 103:1319–1322.
3. Walsh PC. The discovery of the cavernous nerves and development of nerve sparing radical retropubic prostatectomy. J Urol. 2007. 177:1632–1635.
4. Kourambas J, Angus DG, Hosking P, Chou ST. A histological study of Denonvilliers' fascia and its relationship to the neurovascular bundle. Br J Urol. 1998. 82:408–410.
5. Costello AJ, Brooks M, Cole OJ. Anatomical studies of the neurovascular bundle and cavernosal nerves. BJU Int. 2004. 94:1071–1076.
6. Grober ED, Roberts M, Shin EJ, Mahdi M, Bacal V. Intraoperative assessment of technical skills on live patients using economy of hand motion: establishing learning curves of surgical competence. Am J Surg. 2010. 199:81–85.
7. Chipman JG, Schmitz CC. Using objective structured assessment of technical skills to evaluate a basic skills simulation curriculum for first-year surgical residents. J Am Coll Surg. 2009. 209:364–370.
8. Xylinas E, Ploussard G, Salomon L, Paul A, Gillion N, Laet KD, et al. Intrafascial nerve-sparing radical prostatectomy with a laparoscopic robot-assisted extraperitoneal approach: early oncological and functional results. J Endourol. 2010. 24:577–582.
9. Walker JB, Perkins E, Harkey HL. A novel simulation model for minimally invasive spine surgery. Neurosurgery. 2009. 65:6 Suppl. 188–195.
10. Schöffl H, Froschauer SM, Dunst KM, Hager D, Kwasny O, Huemer GM. Strategies for the reduction of live animal use in microsurgical training and education. Altern Lab Anim. 2008. 36:153–160.
11. Reed AB, Crafton C, Giglia JS, Hutto JD. Back to basics: use of fresh cadavers in vascular surgery training. Surgery. 2009. 146:757–762.
12. Bach T, Geavlete B, Herrmann TR, Gross AJ. "Homemade" TURsimulator for less than $40 U.S.? The "Tupper" experience. J Endourol. 2009. 23:509–513.
13. Jussila J. Preparing ballistic gelatine--review and proposal for a standard method. Forensic Sci Int. 2004. 141:91–98.
14. Qayumi K. Surgical skills lab: a hub for competency training. J Invest Surg. 2010. 23:48–56.
15. Scalese RJ, Obeso VT, Issenberg SB. Simulation technology for skills training and competency assessment in medical education. J Gen Intern Med. 2008. 23:Suppl 1. 46–49.
16. Dahm P, Preminger GM, Scales CD Jr, Fesperman SF, Yeung LL, Cohen MS. Evidence-based medicine training in residency: a survey of urology programme directors. BJU Int. 2009. 103:290–293.
17. Piechaud PT, Pansadoro A. Transfer of skills from the experimental model to the patients. Curr Urol Rep. 2006. 7:96–99.
18. Davis JW, Kamat A, Munsell M, Pettaway C, Pisters L, Matin S. Initial experience of teaching robot-assisted radical prostatectomy to surgeons-in-training: can training be evaluated and standardized? BJU Int. 2009. Epub ahead of print.
19. Rowan RL. A test to determine transurethral resection ability. Br J Urol. 1961. 33:323–325.
20. Ooi J, Lawrentschuk N, Murphy DL. Training model for open or laparoscopic pyeloplasty. J Endourol. 2006. 20:149–152.
21. Bartoletti R, Cai T, Tosoratti N, Amabile C, Crisci A, Tinacci G, et al. In vivo microwave-induced porcine kidney thermoablation: results and perspectives from a pilot study of a new probe. BJU Int. 2010. 106:1817–1821.
22. McDougall EM, Kolla SB, Santos RT, Gan JM, Box GN, Louie MK, et al. Preliminary study of virtual reality and model simulation for learning laparoscopic suturing skills. J Urol. 2009. 182:1018–1025.
23. Seixas-Mikelus SA, Kesavadas T, Srimathveeravalli G, Chandrasekhar R, Wilding GE, Guru KA. Face validation of a novel robotic surgical simulator. Urology. 2010. 76:357–360.
24. Schout BM, Muijtjens AM, Hendrikx AJ, Ananias HJ, Dolmans VE, Scherpbier AJ, et al. Acquisition of flexible cystoscopy skills on a virtual reality simulator by experts and novices. BJU Int. 2010. 105:234–239.
25. Sharma D, Shaban A, Riddell A, Kalsi V, Arya M, Grange P. Video-games station or minimally invasive skills training station? BJU Int. 2009. 104:159–160.
26. Shane MD, Pettitt BJ, Morgenthal CB, Smith CD. Should surgical novices trade their retractors for joysticks? Videogame experience decreases the time needed to acquire surgical skills. Surg Endosc. 2008. 22:1294–1297.
27. Wilson M, Coleman M, McGrath J. Developing basic hand-eye coordination skills for laparoscopic surgery using gaze training. BJU Int. 2010. 105:1356–1358.
28. Sugand K, Abrahams P, Khurana A. The anatomy of anatomy: a review for its modernization. Anat Sci Educ. 2010. 3:83–93.
29. Kim DK, Lee JW, Park SY, Kim YT, Park HY, Lee TY. Initial experience with robotic-assisted laparoscopic partial cystectomy in urachal diseases. Korean J Urol. 2010. 51:318–322.
30. Yun HK, Kwon JB, Cho SR, Kim JS. Early experience with laparoscopic retropubic simple prostatectomy in patients with voluminous benign prostatic hyperplasia (BPH). Korean J Urol. 2010. 51:323–329.