Abstract
Purpose
Studies of genetic variation in the prostate-specific antigen (PSA) gene have improved the diagnostic accuracy of PSA for diagnosing prostate diseases in Caucasians. However, the reference ranges and pharmacokinetics of PSA differ significantly according to race. Therefore, we evaluated the association between genetic variations in the PSA promoter area and benign prostatic hyperplasia (BPH) phenotypes in Korean BPH patients.
Materials and Methods
One hundred twenty-one men were enrolled. The initial serum PSA level, prostate size, and PSA changes at 3 months after treatment with dutasteride were determined. We amplified the promoter region of the PSA gene (nucleotide positions -158 to -356 and -5217 to -5429) and sequenced the products.
Results
Three relatively well characterized single-nucleotide polymorphisms (SNPs; rs3760722, rs266867, and rs266868), six uncharacterized SNPs (rs17554958, rs266882, rs4802754, rs2739448, rs2569733, and rs17526278), and one novel SNP (nucleotide position -5402) were found. There were no statistically significant correlations between any of the SNPs of the PSA promoter area and age-adjusted prostate sizes, initial PSA levels, or PSA variations after 3 months of dutasteride treatment.
The incidence of benign prostatic hyperplasia (BPH) increases with advancing age [1]. Even though several hypotheses regarding the trigger for BPH have been suggested, BPH is mainly caused by augmented levels of androgen or dihydrotestosterone (DHT) [2,3]. 5α-Reductase inhibitors (5ARIs) are currently available for reducing the intra-prostatic DHT level, resulting in a reduction of serum prostate-specific antigen (PSA) [2-4].
Because the prostate is the major site of PSA expression in men, serum PSA levels have become the most widely used marker for prostate cancer (PC) screening and for monitoring patient responses to therapeutic interventions [5,6]. However, PSA has several limitations, including a low specificity and low sensitivity for PC detection [7]. Furthermore, PSA levels are highly variable over time. In addition, serum PSA levels after treatment with a 5ARI exhibit a considerable degree of variability [3,8]. Therefore, the inconsistent effect of 5ARIs on serum PSA levels has limited our ability to differentiate BPH from PC. To overcome the limitations of using PSA diagnostically, research on PSA kinetics in prostate diseases has progressed considerably in the last decade [9,10].
Recently, Cramer et al reported that genetic variations in the PSA promoter are associated with serum PSA levels [10]. Thus, an understanding of the PSA promoter genotype may help us to understand PSA kinetics after dutasteride treatment and to develop models of PSA cutoff values to increase the sensitivity or specificity for detecting PC. But whether or not the findings regarding the PSA promoter genotype are universal or applicable to the European population alone is not known because reference ranges of PSA differ significantly according to race [11,12]. Furthermore, because the enrolled population in that study comprised workers exposed to asbestos, the serum PSA levels or genetic phenotypes were not representative of BPH patients [10]. Because there is a significant overlap in PSA values between patients with BPH and those with PC, and because BPH and PC frequently co-exist in aging men, a genetic study involving BPH patients, not the general population, must be performed to understand PSA pharmacokinetics and to differentiate BPH from PC.
Our aim was to evaluate the variations in the PSA promoter gene in Korean patients with BPH, as well as to compare the results with BPH phenotypes and serum PSA changes after dutasteride treatment. We also focused on differences in the PSA promoter gene among different ethnicities that may reflect the different phenotypes in prostate diseases.
The study population comprised 121 Korean men with a clinical diagnosis of BPH (Table 1). Informed consent was obtained from the study participants. The research protocol was approved by the Institutional Review Board of the Dankook University Hospital.
Patients with a serum PSA level >4 ng/ml underwent transrectal biopsy to exclude PC. Blood samples were collected in tubes containing sodium ethylenediamine tetraacetic acid (EDTA) [13]. We used a QIAmp blood extraction kit (Qiagen, Valencia, CA, USA) for DNA extraction. Serum PSA levels were determined with a PSA kit (PSA-RIACT; CIS Bio International, Gif Sur Yvette, France). Prostate size was determined through transrectal ultrasound (HD7 Ultrasound System; Philips, Shenyang, China). We prescribed one tablet of dutasteride (0.5 mg/day; GSK, Beinheim, France) for 3 months and determined serum PSA changes by serial testing. PSA changes were calculated as a ratio (PSA level at 3 months/initial PSA level). Three people were lost to follow-up.
DNA samples from the 121 subjects were amplified by polymerase chain reaction (PCR). All PCR products yielded specific amplifications, but approximately 6% of the samples failed to yield specific genetic information or yielded partially ambiguous DNA sequencing data. The ambiguous sequencing data at specific single-nucleotide polymorphism (SNP) sites were excluded from the data analysis.
We performed PCR to amplify 2 regions in the PSA promoter gene (-158 to -356 and -5217 to -5429 from the transcription start site; Fig. 1). The primer sequences of the -158 to -356 region were 5'-GGGATCAGGGAGTCTCA CAA-3' and 5'-AAACCTTCATTCCCCAGGAC-3', respectively. The primer sequences of the -5217 to -5429 region were 5'-TGTTAGCCAGGATGGTCTCA-3' and 5'-CCTCA GAGCCTGAGAGGTCA-3', respectively. The PCR was performed in a 2720 Thermal Cycler (Applied Biosystems, Foster City, CA, USA). We used 25 to 70 ng of genomic DNA as a template in a total PCR volume of 50 µl. Each tube contained 25 µl of 2x GoTaq Green Master Mix (400 µM dATP, 400 µM dGTP, 400 µM dCTP, 400 µM dTTP, and 3 mM MgCl2; Promega, Madison, WI, USA), 300 nM of each oligonucleotide primer, and 2 µl of extracted DNA template. All reaction samples were heated to 94℃ for 5 minutes and were then subjected to 30 cycles at 95℃ for 1 minute, 55℃ for 1 minute, and 72℃ for 1 minute. A final extension was performed at 72℃ for 5 minutes. After purification (Bioneer, Daejeon, Korea), we identified the sequences with a BigDye Terminator Sequencing Kit (Applied Biosystems) using the above four primers.
Hardy-Weinberg equilibrium tests for all genotyped SNPs and pairwise linkage disequilibrium tests for all pairs of genotyped SNPs were performed by using the Haploview program [14]. Lewontin's D' was used to estimate the strength of pairwise linkage disequilibrium. An association between the genetic variations and BPH phenotypes was estimated by using SPSS ver. 11.0 (SPSS Inc., Chicago, IL, USA). p-values<0.05 were considered statistically significant.
We identified 10 SNPs in this region (Fig. 1, 2). Three relatively well-characterized SNPs (rs3760722, rs266867, and rs266868), six uncharacterized SNPs (rs17554958, rs266882, rs4802754, rs2739448, rs2569733, and rs17526278), and one novel SNP (nucleotide position -5402) were found. One polymorphism at -206 (rs17554958) was a T insertion or deletion variation (Fig. 2), and the other nine polymorphisms showed a typical SNP phenotype: two homogenotypes and one heterogenotype. Because the rs3760722 significantly deviated from the Hardy-Weinberg equilibrium (HWE; p=0.002), it was removed from further statistical analysis.
The 121 study subjects had a Mean±SD age of 70.33±6.92 years and a mean serum PSA concentration of 6.56±8.48 ng/ml. There were no significant differences in the age intervals among genetic variations in each SNP. No SNPs were associated with the initial serum PSA level, prostate volume, or PSA changes after 3 months of dutasteride treatment (p>0.05, chi-square test) (Table 2). We used haplotype analysis in eight SNPs by using the Haploview program. The results showed one typical haplotype at -5217, -5307, and -5412. Haplotype 1 (TGC), haplotype 2 (TAT), haplotype 3 (AGT), and haplotype 4 (TGT) had an estimated frequency of 41.7%, 31.5%, 15.2%, and 10.6%, respectively.
We tested whether specific combinations of these sequence variants were associated with BPH phenotypes. As shown in Table 3, our study population had two major haplotypes. Men who had one copy of the -5217 TT/-5307AA or AG/-5412CC haplotype had significantly higher PSA levels than did men who had copies of the -5217AA or AT/-5307 GG/-5412 TT or CT haplotypes (p=0.012, adjusted for age). However, other phenotypes, such as prostate size and PSA changes after dutasteride, were not associated with the above haplotype. Pairwise linkage disequilibrium tests demonstrated that some of the SNPs examined in the PSA promoter gene were in strong linkage disequilibrium, but others were not (Table 4).
Testosterone is converted to the potent DHT by a steroid 5α-reductase enzyme that binds to and activates the androgen receptor (AR) [2]. The activated AR mediates and up-regulates the expression of PSA through androgen response elements (AREs) in the promoter gene. Interventions that inhibit 5α-reductase enzyme inactivate the AR and down-regulate serum PSA levels [2,15]. Thus, the median change in PSA in dutasteride-treated patients is -59.5% at 2 years, which increases to -66.1% at 4 years [3]. Our study evaluated the early changes in serum PSA after dutasteride treatment; the serum PSA level was reduced 25% from initial PSA levels.
The serum PSA levels after 5ARI treatment are generally reduced; however, there are interindividual variations in the reduced PSA levels. It is difficult to differentiate patients with BPH from those with PC because of the variation in PSA levels; thus, an understanding of PSA pharmacokinetics and the prediction of individual PSA changes after treatment with 5ARIs is of enormous practical significance [3,10,16].
Xue et al reported that serum PSA is associated with PSA genotype at -158, with higher PSA levels among men with the PSA AA genotype than among men with the AG or GG genotypes [17]. This polymorphism has also been associated with an increased risk for the development of PC [18,19]. However, another group reported that this polymorphism was not associated with the serum PSA level in two separate groups of men without PC [20,21].
In a recent study, SNPs in the PSA promoter gene near -6 kb in the 5' region (the -4643G/A SNP [G allele], the -5412C/T SNP [C allele], and the -5429T/G SNP [G allele]) were associated with increases in serum PSA levels. Those authors concluded that genetic variations in the PSA promoter gene are associated with serum PSA levels in men without prostatic disease [10]. Thus, the PSA promoter genotype may help to develop models of PSA cutoff values [10,18,19].
Each individual has a change in DNA sequence of approximately 1 DNA base in every 200 to 300 bases compared with other individuals [22]. The ARE areas in our study involved 10 SNPs in approximately 800 bp. A high incidence of SNPs in an ARE area may be responsible for different phenotypes or inherited individual differences in BPH or PC phenotypes.
Three polymorphism sites (rs3760722, rs266867, and rs266868) are well characterized in the HapMap information. The genotypes of the rs266868 polymorphism in Japanese and Chinese populations from HapMap data were as follows: GG type, 36% and 47%; AG type, 45% and 46%; and AA type, 18% and 6%, respectively. Our data for the rs266868 polymorphism, which were very similar to the results from HapMap data, were as follows: GG type, 47.3%; AG type, 40.3%; and AA type, 12.2%. The genotype of the rs266867 polymorphism in Japanese and Chinese populations was as follows: AA type, 1.2% and 3.6%; AT type, 29.4% and 38.1%; and TT type, 69.4% and 58.3%, respectively. Our data for the rs266867 polymorphism were as follows: AA type, 2.6%; AT type, 27.1%; and TT type, 70.1%.
Six uncharacterized SNPs (rs17554958, rs266882, rs4802754, rs2739448, rs2569733, and rs17526278) were found. The polymorphism at -206 (rs17554958) was a T insertion or deletion variation, whereas the other five polymorphisms showed two homogenotypes and one heterogenotype. One novel SNP (nucleotide position -5402) was also found. Because the incidence of the CT and TT genotypes is rare (1.6% and 0.8% in the novel SNP), large numbers of samples are needed for a complete characterization.
The 121 study subjects from whom the samples were obtained had a mean±standard deviation age of 70.33±6.92 years and a mean serum PSA concentration of 6.56±8.48 ng/ml. Because the serum PSA from the enrolled population was higher than that reported in other studies, some patients in our study may had coexisting PC. To reduce contamination with PC, we routinely obtain prostate biopsies in patients with a serum PSA >4 ng/ml. Sixteen patients with a serum PSA ≥10 ng/ml underwent prostate biopsies and follow-up at least 2 years after dutasteride treatment. They all showed continuously decreased serum PSA levels for 2 years, thus suggesting BPH. It is well known that the median PSA level consistently decreases from baseline in both patients with and those without PC within the early period after dutasteride treatment. However, from month 12 on, the median PSA increases in patients eventually diagnosed with PC, whereas it continues to decrease in those without a subsequent diagnosis of PC [3,16].
There were no statistically significant differences in age intervals among genetic variations in each SNP. Interestingly, no SNPs were significantly associated with the serum PSA level, prostate volume, or PSA changes after 3 months of dutasteride treatment (Table 2). Our results were different from the report of Cramer et al [10]. There are several reasons for these differences. For the most part, the characteristics of the enrolled patients differed. Cramer et al included asbestos workers who were recruited for a study of the interaction of asbestos exposure with genetic and environmental factors [10]. Moreover, their patients were younger than our patients, and the median serum PSA concentration was lower than the median serum PSA level in our patients. Our study population comprised BPH patients who complained of voiding symptoms. Furthermore, all patients who had an ultrasound were shown to have a large prostate volume (>30 cc). It is well known that there is a clinically significant relationship between serum PSA and prostate volume in men with BPH [6,8]. Our population usually had a large prostate volume, which was the indication for dutasteride treatment.
We must also consider the ethnicity difference between Caucasians and Asians. Oesterling et al reported that the age-specific reference ranges were lower for serum PSA levels and higher for PSA density in Japanese men relative to Caucasian men [12]. Because PSA levels depend markedly on age and might be significantly influenced by race, a given PSA value may have different clinical meaning for patients of different races [11,12].
We used haplotype analysis in nine SNPs by use of the Haploview program. Pairwise linkage disequilibrium tests demonstrated that most of the SNPs examined in the upper area of the PSA gene promoter (-5307 to -5429) were in strong linkage disequilibrium (Table 4). However, there was weak linkage disequilibrium between the -158 SNP and the upper area of the PSA gene. Our results differed from those of Cramer et al, who reported linkage disequilibrium between the -158 SNP and further upstream SNPs [10]. This suggests that there are ethnicity differences in the PSA gene between Caucasians and Asians. Further study will be needed to determine whether the differences are attributed to ethnicity or different disease phenotypes.
Our results showed 1 haplotype at -5217, -5307, and -5412. Haplotype 1 (TGC), haplotype 2 (TAT), haplotype 3 (AGT), and haplotype 4 (TGT) had an estimated frequency of 41.7%, 31.5%, 15.2%, and 10.6%, respectively. Interestingly, men who had copies of the -5217TT/-5307AA or AG/-5412CC haplotype had a significantly higher PSA level than did men who had copies of the -5217AA or AT/-5307 GG/-5412 TT or CT phenotype (p=0.012, adjusted for age). We could not conclude whether these results were clinically meaningful or represent an accidental error.
The present findings suggest that genetic variations in the PSA promoter gene are not associated with initial PSA levels, prostate volume, or PSA changes after dutasteride treatment in Korean patients with BPH. Again, we could not predict the serum PSA changes after dutasteride treatment according to PSA promoter genotype alone in Korean patients with BPH. However, the finding that 1 haplotype in -5217, -5307, and -5412 was associated with the initial serum PSA level suggests possible genetic involvement. Additional research or a larger enrolled population will be needed to determine the mechanisms of different patterns of the same PSA promoter gene among different racial groups.
Figures and Tables
FIG. 1
Schematic structure and single-nucleotide sequence polymorphisms in two prostate-specific antigen promoter areas (-158 to -356 and from -5217 to -5429) with respect to the start of transcriptiona and contig positions of NT011109.15b. a: respect to the start of transcription, b: respect to the contig positions of NT11109.15.

FIG. 2
Representative single-nucleotide sequence polymorphisms (arrows) in this study. One polymorphism at -206 (rs17554958) was a T insertion or deletion variation, and the other nine polymorphisms showed a typical SNP phenotype, two homotypes, and one heterotype.
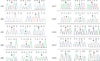
ACKNOWLEDGEMENTS
We thank Lee Ji-Young (Division of Bio-Medical and Informatics, Korea Centers for Disease Control and Prevention) for her constructive comments for use of the Haploview program.
References
1. Berry SJ, Coffey DS, Walsh PC, Ewing LL. The development of human benign prostatic hyperplasia with age. J Urol. 1984. 132:474–479.
2. Carson C 3rd, Rittmaster R. The role of dihydrotestosterone in benign prostatic hyperplasia. Urology. 2003. 61:4 Suppl 1. 2–7.
3. Marks LS, Andriole GL, Fitzpatrick JM, Schulman CC, Roehrborn CG. The interpretation of serum prostate specific antigen in men receiving 5alpha-reductase inhibitors: a review and clinical recommendations. J Urol. 2006. 176:868–874.
4. Lilja H. A kallikrein-like serine protease in prostatic fluid cleaves the predominant seminal vesicle protein. J Clin Invest. 1985. 76:1899–1903.
5. Ulmert D, O'Brien MF, Bjartell AS, Lilja H. Prostate kallikrein markers in diagnosis, risk stratification and prognosis. Nat Rev Urol. 2009. 6:384–391.
6. Roehrborn CG. The utility of serum prostatic-specific antigen in the management of men with benign prostatic hyperplasia. Int J Impot Res. 2008. 20:Suppl 3. S19–S26.
7. Pienta KJ. Critical appraisal of prostate-specific antigen in prostate cancer screening: 20 years later. Urology. 2009. 73:5 Suppl. S11–S20.
8. Lee GH, Hong JH, Kim HJ. The effect of dutasteride on serum prostate-specific antigen (PSA) in patients with benign prostate hypertrophy. J Korean Continence Soc. 2008. 12:42–47.
9. Fitzpatrick JM, Banu E, Oudard S. Prostate-specific antigen kinetics in localized and advanced prostate cancer. BJU Int. 2009. 103:578–587.
10. Cramer SD, Chang BL, Rao A, Hawkins GA, Zheng SL, Wade WN, et al. Association between genetic polymorphisms in the prostate-specific antigen gene promoter and serum prostate-specific antigen levels. J Natl Cancer Inst. 2003. 95:1044–1053.
11. Ku JH, Ahn JO, Lee CH, Lee NK, Park YH, Byun SS, et al. Distribution of serum prostate-specific antigen in healthy Korean men: influence of ethnicity. Urology. 2002. 60:475–479.
12. Oesterling JE, Kumamoto Y, Tsukamoto T, Girman CJ, Guess HA, Masumori N, et al. Serum prostate-specific antigen in a community-based population of healthy Japanese men: lower values than for similarly aged white men. Br J Urol. 1995. 75:347–353.
13. Song J, Kim DY, Kim CS, Kim HJ, Lee DH, Lee HM, et al. The association between Toll-like receptor 4 (TLR4) polymorphisms and the risk of prostate cancer in Korean men. Cancer Genet Cytogenet. 2009. 190:88–92.
14. Gabriel SB, Schaffner SF, Nguyen H, Moore JM, Roy J, Blumenstiel B, et al. The structure of haplotype blocks in the human genome. Science. 2002. 296:2225–2229.
15. Kim J, Coetzee GA. Prostate specific antigen gene regulation by androgen receptor. J Cell Biochem. 2004. 93:233–241.
16. Andriole G, Bostwick D, Civantos F, Epstein J, Lucia MS, McConnell J, et al. The effects of 5alpha-reductase inhibitors on the natural history, detection and grading of prostate cancer: current state of knowledge. J Urol. 2005. 174:2098–2104.
17. Xue WM, Coetzee GA, Ross RK, Irvine R, Kolonel L, Henderson BE, et al. Genetic determinants of serum prostate-specific antigen levels in healthy men from a multiethnic cohort. Cancer Epidemiol Biomarkers Prev. 2001. 10:575–579.
18. Xue W, Irvine RA, Yu MC, Ross RK, Coetzee GA, Ingles SA. Susceptibility to prostate cancer: interaction between genotypes at the androgen receptor and prostate-specific antigen loci. Cancer Res. 2000. 60:839–841.
19. Medeiros R, Morais A, Vasconcelos A, Costa S, Pinto D, Oliveira J, et al. Linkage between polymorphisms in the prostate specific antigen ARE1 gene region, prostate cancer risk, and circulating tumor cells. Prostate. 2002. 53:88–94.
20. Xu J, Meyers DA, Sterling DA, Zheng SL, Catalona WJ, Cramer SD, et al. Association studies of serum prostate-specific antigen levels and the genetic polymorphisms at the androgen receptor and prostate-specific antigen genes. Cancer Epidemiol Biomarkers Prev. 2002. 11:664–669.
21. Rao A, Chang BL, Hawkins G, Hu JJ, Rosser CJ, Hall MC, et al. Analysis of G/A polymorphism in the androgen response element I of the PSA gene and its interactions with the androgen receptor polymorphisms. Urology. 2003. 61:864–869.
22. Tebbutt SJ, James A, Paré PD. Single-nucleotide polymorphisms and lung disease: clinical implications. Chest. 2007. 131:1216–1223.