Abstract
Purpose
Carbon monoxide (CO) may mediate smooth muscle relaxation in the rat corpus cavernosum smooth muscle (CCSM). We hypothesized that CO plays a role in neurally derived, frequency-dependent relaxation of rat CCSM.
Materials and Methods
To study the effect of CO on CCSM relaxation induced by electrical field stimulation (EFS), a CCSM bundle was mounted on a force transducer and perfused with Hanks' balanced salt solution at 37℃ with 95% O2 and 5% CO2. After 1 hour equilibration with -500 mg of passive tension, contraction of the CCSM bundle was elicited by 10-5 M phenylephrine, which was continuously added with different concentrations of CO (1%, 2%, and 5%). Frequency-dependent relaxation was induced by EFS trains (0.2 ms at 0.5-32 Hz, for 10 s) repeated at 2 min intervals over 15 min in the presence of adrenergic and muscarinic receptor blocking agents (guanethidine and atropine, respectively). To study the distribution of heme oxygenase-2 (HO-2) in the rat CCSM, we performed immunohistochemical evaluation.
Results
CO produced a dose-dependent enhancement of EFS-induced relaxation. Pretreatment with NG-nitro-L-arginine (a nitric oxide synthase blocker) greatly reduced the EFS-induced relaxation in the presence of CO (-45%). Pretreatment with zinc protoporphyrin-IX (ZnPP-9, a heme oxygenase inhibitor) had no significant effect on EFS-induced relaxation in the absence or the presence of CO. We found immunoreactivity for HO-2 in CCSM and immunoreactivity for protein gene product 9.5 (PGP 9.5) in nerve fibers.
Conclusions
We conclude that CO produced a dose-dependent enhancement of EFS-induced relaxation in rat CCSM bundles, but neurally derived, frequency-dependent relaxation in the rat CCSM depended mostly on nitric oxide in response to nonadrenergic noncholinergic neurotransmission. Immunoreactivity for HO-2 was found in rat CCSM but not nerve fibers.
Relaxation of cavernosal smooth muscle and artery is important in the process of penile erection. Previous studies indicated that nitric oxide (NO) is a physiological messenger molecule/neurotransmitter that mediates the regulation of corpus cavernosum smooth muscle (CCSM) function and arterial tone by the activation of soluble guanylate cyclase (SGC) with binding to the heme moiety of that enzyme [1,2]. NO is known to be produced in the nerves, endothelium, and muscle by nitric oxide synthase (NOS) [3,4]. The enzyme NOS has close homology to cytochrome P-450 reductase, the source of electrons for heme oxygenase (HO)-mediated production of carbon monoxide (CO), activating guanylate cyclase by binding to the heme moiety [5,6].
It has been proposed that CO might act in a fashion similar to that of NO [7]. CO is a noxious gas that can bind to the iron atom of hemoproteins and that also activates SGC, leading to an increase in endogenous cyclic guanosine 3',5'-monophosphate (cGMP) levels and consequent relaxation of smooth muscle [8,9]. Like NO, exogenously applied CO has been shown to cause relaxation of various isolated blood vessels by increasing endogenous cGMP levels and has also been claimed as a novel neuroendocrine modulator for the regulation of vascular smooth muscle tone [9]. The penis is a vascular organ, and therefore, CO may have roles in the tumescence or detumescence of the corpus cavernosum in conjunction with NO. However, previous studies on rabbit CCSM and rabbit cerebral arteries indicated that endogenous CO had no significant effect on relaxation or tone [10,11].
Penile erection following relaxation of CCSM is known to be primarily mediated by NO produced in response to nonadrenergic noncholinergic (NANC) neurotransmission [11,12]. NO also mediates a significant component of NANC neurotransmission in intestinal smooth muscle [13]. CO is required for NO-mediated intestinal NANC neurotransmission [13]. In addition, the suppression of NANC-mediated relaxation by the HO inhibitor (zinc protoporphyrin-IX; ZnPP-9) was reported to imply the involvement of CO in neurally mediated sphincteric smooth muscle relaxation [14]. In the presence of adrenergic and muscarinic receptor blocking agents (guanethidine and atropine, respectively), electrical field stimulation (EFS) excites only the NANC nerves, which leads to rapid and transient relaxation in pre-contracted CCSM [12]. The purpose of this study was to examine the hypothesis that CO plays a role in neurally derived, frequency-dependent relaxation of rat CCSM in response to NANC neurotransmission.
Male Sprague-Dawley rats (-300 g) were used in the present study. Animals were anesthetized with ketamine (60 mg/kg) and xylazine (2.5 mg/kg). Corporal biopsy samples were obtained from 15 rats (5 for immunohistochemistry and 10 for isometric force measurement). All procedures were approved by the Institutional Animal Care and Use Committee of the Chonbuk National University.
Custom-made gas mixtures with different concentrations of CO (1%, 2%, 5%) were obtained from Praxair, Inc. (St. Louis, MO, USA). Zamboni's fixative (2% paraformaldehyde, 0.2% picric acid in 0.1 M phosphate buffer, pH 7.4) was obtained from Newcomer (Middleton, WI, USA). Triton-X-100, phenylephrine, NG-nitro-L-arginine (L-NOARG), guanethidine, atropine, and ZnPP-9 were obtained from Sigma (St. Louis, MO, USA). Ornithine carbamoyltransferase (OCT) compound was obtained from Tissue-Tek, Sakura, Finetek Inc. (CA, USA). Normal donkey serum (NDS) was obtained from Jackson, Immuno-Research Laboratory (West Grove, PA, USA). Antibody raised in rabbit to HO-2 was obtained from StressGen Biotechnology (Victoria, BC, Canada). Antibody raised in mouse to protein gene product 9.5 (PGP 9.5) was obtained from Biogenesis (Sandown, NH, USA). CYTM3-conjugated AffiniPure donkey anti-rabbit IgG and CYTM2-conjugated AffiniPure donkey anti-mouse IgG were obtained from Jackson, ImmunoResearch Laboratory (West Grove, PA, USA). 10x Hank's balanced salt solution (HBSS) was obtained from Invitrogen Corporation (Grand Island, NY, USA).
ZnPP-9 (0.1 mM) was dissolved in a small volume of 0.1 N NaOH solution, diluted in HBSS, and titrated to pH 7.4 with 0.1 N HCl. Other substances were dissolved in HBSS solution.
All specimens were immediately immersed in Zamboni's fixative (2% paraformaldehyde, 0.2% picric acid in 0.1 M phosphate buffer, pH 7.4, Newcomer Supply, Middleton, WI, USA) for 4 hour at 4℃, followed by several washes in cold phosphate-buffered saline (PBS) solution. Prior to sectioning, all tissues were stored at 4℃ in PBS containing 30% sucrose solution and 0.01% sodium azide (Sigma Chemicals Co., St. Louis, MO, USA) for at least 24 hour. All sections were immediately processed by immersion in OCT compound (Tissue-Tek, Sakura, Finetek Inc., CA, USA) before snap-freezing in -60℃ viscous isopentane (Sigma Chemicals Co., St. Louis, MO, USA) cooled with a dry ice slurry. The frozen samples were then stored at -70℃ for subsequent immunohistochemical evaluation.
Cryostat sections, 20 µm thick, were mounted on slides coated with gelatin and chrome alum. Slides were rinsed 3 times with 0.1 M PBS solution for 30 min. All slides were subsequently incubated in PBS solution containing 0.3% (v/v) Triton-X 100 (Sigma Chemical Co., St. Louis, MO, USA) and 10% (v/v) NDS (Jackson, ImmunoResearch Laboratories, Inc., West Grove, PA, USA) for 120 min at room temperature in a humid chamber. Slides were incubated overnight with antiserum raised in rabbits to HO-2 (1:500 diluted in 0.1 M PBS solution containing 5% NDS, 0.3% Triton-X 100; StressGen Biotechnologies, Corp., Victoria, British Columbia, Canada) and antiserum raised in mouse to PGP 9.5 (1:1,600 diluted in 0.1 M PBS containing 5% NDS, 0.3% Triton-X 100; Biogenesis, Sandown, NH, USA) in a humid chamber at room temperature. Unbound primary antibodies were washed from the sections with 0.1 M PBS solution 3 times for 10 min. After this washout, the sections were exposed for 2 hour at room temperature to CYTM3-conjugated AffiniPure donkey anti-rabbit IgG (1:100 diluted in 0.1M PBS containing 5% NDS, 0.3% Triton-X 100; Jackson, ImmunoResearch Laboratories, Inc., West Grove, PA, USA) for HO-2 and CYTM2-conjugated AffiniPure donkey anti-mouse IgG (1:200 diluted in 0.1 M PBS solution containing 5% NDS, 0.3% Triton-X 100; Jackson, ImmunoResearch Laboratories, Inc., West Grove, PA, USA) for PGP 9.5. Following this secondary antiserum incubation, all sections were rinsed 3 times for 10 min with 0.1 M PBS solution. The specimens were air-dried and cover-slipped by using xylene and were viewed with a Zeiss Axiophoto fluorescence microscope equipped with epi-illumination. To obtain CYTM3-fluorescence and CYTM2-fluorescence, excitation wavelengths of 568 nm and 488 nm were used, respectively. Photographs of these fluorescent signals were taken at a magnification of ×400 with Kodak (400 ASA) film.
A thin bundle of rat CCSM (2-3 mm long and -300 µm wide) was dissected under a dissecting microscope and mounted on a force transducer. The bundle, surrounded by the quartz cuvette (90 µl), was constantly perfused with HBSS buffered with 10 mM N-[2-Hydroxyethyl]piperazine-N'[2-ethanesulfonic] acid (pH 7.4) aerated with 95% O2 and 5% CO2 at 37℃. After 1 hour equilibration with -500 mg of passive tension, contraction of the CCSM bundle was elicited by 10 µM phenylephrine (Phe; Sigma Chemical Co., St. Louis, MO, USA). Force output signal was digitized at 10 samples per s and displayed on a computer monitor by use of LabVIEW software (LabVIEW 5.0, Graphical Programming for Instrumentation).
Frequency-dependent relaxation in Phe-precontracted bundles was induced by EFS trains (0.2 ms pulse duration at 0.5-32 Hz, for 10 s; Grass Stimulator, S88) repeated at 2-min intervals over 15 min. The experiments were performed in the presence of adrenergic and muscarinic receptor blocking agents (10 µM guanethidine and 1 µM atropine, respectively). Following the control measurement in the absence of CO, the Phe-precontracted bundle was washed out with HBSS until it relaxed to the resting state. The resting fiber bundle inside the quartz cuvette was again precontracted by perfusing Phe solution, to which different concentrations of CO mixtures (1%, 2%, and 5%; Filling Method: Gravimetric; Praxair, Inc.) were continuously added during the plateau phase, respectively.
Effects of inhibitors on EFS-induced relaxation from the Phe-induced contraction was measured in the presence of CO. Before the Phe-induced contraction, the fiber bundle was incubated for 1 hour with ZnPP-9 (0.1 mM) and L-NOARG (100 µM; NOS inhibitor), respectively.
The in vitro isometric force response of CCSM to Phe varied across preparations. Therefore, relaxation effects were reported as % of initial Phe-induced force response for each preparation. The normalized data were expressed as the mean±standard error (SE). Statistical comparisons were performed by using Student's t-test for paired comparisons. Multiple comparisons were made with an analysis of variance (ANOVA). Differences were considered significant when p<0.05.
The preparations in which non-immune normal rabbit serum at a dilution of 1:500 was used instead of the primary antibody served as negative control (Fig. 1A). Immunoreactivity for HO-2 was observed throughout the CCSM (Fig. 1B), and immunoreactivity for PGP 9.5 was detected in nerve fibers (Fig. 1C) but not specifically in relation to the CCSM detected by HO-2 immunoreactivity (Fig. 1D).
Before application of EFS to Phe-precontracted CCSM, only the highest concentration of CO (5%) caused -12% relaxation in Phe-precontracted CCSM. However, this relaxant effect was reversed in the absence of CO (Fig. 2). Fig. 3 shows the effect of 5% CO on the CCSM relaxation induced by EFS. CO produced a dose-dependent enhancement of EFS-induced relaxation. While the frequency of EFS increased from 0.5 Hz to 2.0 Hz, the effect of different CO concentrations (1%, 2%, and 5%) on CCSM relaxation was dose-dependently enhanced (12%, 15%, and 34% compared to control, respectively). During these low ranges of frequencies, there were statistically significant differences in the relaxation of CCSM among the three groups of different CO concentrations (p<0.05). During high ranges of frequencies (above 2.0 Hz), however, CCSM relaxation induced by EFS was maintained at a similar degree to relaxation demonstrated by 2.0 Hz (10%, 16%, and 32% compared to control, respectively), and there were also significant differences in the relaxation of CCSM among the three groups (p<0.05) (Fig. 4).
Fig. 5 shows the effect of L-NOARG (an NOS inhibitor) on rat CCSM relaxation induced by EFS in the presence of CO (5%). Pretreatment with L-NOARG greatly reduced the EFS-induced relaxation in the presence of CO (-45% when compared to control) (p<0.05), but ZnPP-9 had no significant effect on EFS-induced relaxation (p>0.05) (Fig. 6).
CO is a low molecular weight oxide that is endogenously produced from fatty acids and heme. CO is mainly produced by microsomal HO and activates SGC, leading to an increase in cGMP levels and consequent relaxation of the smooth muscle [8,12]. There are two main isoforms of HO, HO-1 (inducible) and HO-2 (constitutive) [1,5], leading to the formation of biliverdin and CO and the release of iron. In addition, a third isoform of HO, HO-3, has been reported to have strong homology to HO-2, but little is known about its function [15]. HO-1 is inducible and is concentrated in organs in which erythrocyte hemoglobin degradation by mononuclear phagocytic cells normally takes place [16]. HO-2 is constitutively expressed in various rat tissues [16]. The levels of HO prevail from low to moderate in thymus, adrenal gland, kidney, lung, and heart [5].
The present study demonstrated HO-2 immunoreactivity in rat CCSM (Fig. 1B). However, HO-2 immunoreactivity was not specifically localized to nerve fibers in CCSM. Although exposure to exogenous CO (1%, 2%, and 5%) produced a dose-dependent enhancement of EFS-induced transient relaxation in Phe-precontracted CCSM (Fig. 5), the effect of endogenously released CO via nerve stimulation on EFS-induced relaxation was not observed in this study (Fig. 6). CO released by nerve terminals on EFS does not seem to have an important role in relaxation of CCSM. This result was supported by the fact that exposure to a solution containing ZnPP-9 did not show significant inhibition of EFS-induced relaxation (Fig. 6). However, CO has been reported to potentiate EFS-induced NO release by modulating NO production [17,18]. In addition, exogenous CO-induced relaxation in Phe pre-contracted CCSM before exposure to EFS was observed (Fig. 2). This relaxation may be mediated by CO-dependent activation of SGC and subsequent elevation of cGMP or CO-dependent induction of NO release [18,19].
Furchgott and Jothianandan reported that CO was less potent than NO as a relaxant in rabbit aorta (1:1,000) [9]. It was also reported that CO does not seem to have significant effects on tone in cerebral arteries compared to NO [10]. Although CO may function like NO in terms of its ability to relax rat CCSM by stimulating SGC, the effect of CO as an endogenous neurotransmitter for relaxation could not be demonstrated in the rat CCSM. CO may function as an endogenous smooth muscle dilator when its concentration is sufficiently high or when endogenous production of NO is relatively low [20].
As with NO, CO is produced endogenously from NADPH-dependent enzymatic peroxidation of microsomal lipids and from NADPH-dependent oxidative heme destruction catalyzed by HO [14]. CO is also predominantly derived from the breakdown of heme and functions as another gaseous signaling molecule to stimulate SGC [1]. In other smooth muscles, CO has been demonstrated to be a neural messenger, important in regulating smooth muscle tone via alterations in endogenous cGMP levels [14,21]. However, unlike NO, CO was reported to have a cGMP-independent pathway. By activating the mitogen-activated protein kinase (MAPK) pathway, CO has cytoprotective effects such as anti-inflammation and anti-apoptosis. The MAPK pathway is involved in proliferation, apoptosis, and cytokine release [19]. Both CO and NO directly modify the function of other proteins, but when acting on the same protein, they may have different sites of action: calcium-activated large conductance potassium channels. NO increases the activity of calcium-dependent potassium channels via modifications to sulfhydryl groups, whereas CO modulates the activity of the same channels by modification of histidine residues. The effects of NO are mediated on the β subunit of the potassium channel, whereas those of CO are on the α subunit, again indicating the divergent mechanisms of action of NO and CO [22].
It is generally accepted that NO is an essential messenger for penile erection [23]. NO is normally produced by organs and serves as an important chemical messenger not only in the regulation of vascular tone, but also in neural transmission. NO-independent relaxation of certain blood vessels has been attributed to an endothelium-derived relaxing factor that hyperpolarizes vascular smooth muscle, which is referred to as endothelium-derived hyperpolarizing factor (EDHF) [24]. CO may be considered to work like EDHF. Ewing et al reported that HO-2 exists in endothelial cells of the descending aorta, and Zakhary et al discovered that HO-2 immunoreactivity was present in cultured bovine aortic endothelial cells [20,25].
NOS has been shown to be a cytochrome p-450 like hemoprotein, containing a bound protoporphyrin IX. This may provide a mechanism for feedback regulation of the enzyme by NO or CO. The NO receptor, SGC, is also a hemoprotein [26]. It is highly likely that neurons containing guanylyl cyclase or NOS might bring about high levels of HO to allow modulation of these enzymes with CO or to regulate intracellular heme levels [27]. The presence of HO-2 immunoreactivity in CCSM suggests that CO may play a role as a biological messenger molecule analogous to NO, albeit not through a neurally mediated release. In the normal vasculature, the basal production of CO may be lower than that of NO.
As we previously stated, CO is a less potent vasodilator than NO and may not be the predominant regulator of vessel integrity under basal conditions. However, during conditions of vascular injury such as those caused by hypoxia, NO induction of HO stimulates the release of CO, thereby increasing levels of guanylate cyclase, which may offer a means of maintaining blood flow to damaged areas of vasculature [28]. The differential distribution of NOS and HO also suggests that NO may compete with CO in spinal cord neurons [29]. Endogenously produced CO may shut down electron flow through the enzyme by binding to the heme group of NOS, leading to further suppression of NOS and NO formation such as negative feedback control [30]. Because we used normal rats in the present study, it was not possible to study the pathophysiological roles of CO in rat CCSM.
Figures and Tables
FIG. 1
Immunohistochemical staining with antiserum raised in rabbit to heme oxygenase-2 in rat penile smooth muscle. (A) Negative staining with rabbit serum (primary antibody) and secondary antibody (CYTM3-conjugated AffiniPure donkey anti-rabbit IgG) (Control). (B) Positive staining with primary antibody (antiserum raised in rabbits to heme oxygenase-2) and secondary antibody (CYTM3-conjugated AffiniPure donkey anti-rabbit IgG). (C) Nerve fibers stained with primary antibody (antiserum raised in mouse to protein gene product 9.5) and secondary antibody (CYTM2-conjugated AffiniPure donkey anti-mouse IgG). (D) A composite of B and C.
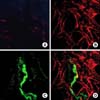
FIG. 2
Effect of different carbon monoxide (CO) concentrations (1%, 2%, and 5%) on relaxation in Phe-precontracted corpus cavernosum smooth muscle before exposure to electrical field stimulation. HBSS: Hank's balanced salt solution.
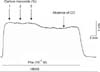
FIG. 3
Effect of carbon monoxide (5%) on corpus cavernosum smooth muscle (CCSM) relaxation induced by electrical field stimulation (EFS). Rat CCSM was phenylephrine-precontracted and responded to EFS (0.5 to 32 Hz, 0.2 ms duration). HBSS: Hank's balanced salt solution.
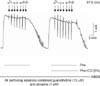
FIG. 4
Frequency-dependent relaxation of phenylephrine-precontracted rat corpus cavernosum smooth muscle on different carbon monoxide concentrations (1%, 2%, and 5%). CTL: control, CO: carbon monoxide.
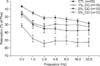
FIG. 5
Effect of nitric oxide synthase inhibitor, NG-nitro-L-arginine, on rat corpus cavernosum smooth muscle relaxation induced by electrical field stimulation in the presence of carbon monoxide (5%). CO: carbon monoxide, HBSS: Hank's balanced salt solution, L-NOARG: NG-nitro-L-arginine, EFS: electrical field stimulation.
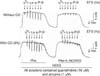
FIG. 6
Inhibition of electrical field stimulation-induced relaxation of rat corpus cavernosum smooth muscle by antagonists (NG-nitro-L-arginine, zinc protoporphyrin-IX) in the presence of carbon monoxide (5%). CTL: control, CO: carbon monoxide, L-NOARG: NG-nitro-L-arginine, ZnPP: zinc protoporphyrin.
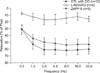
References
1. Gross SS, Wolin MS. Nitric oxide: pathophysiological mechanisms. Annu Rev Physiol. 1995. 57:737–769.
2. Andersson KE, Stief CG. Neurotransmission and the contraction and relaxation of penile erectile tissue. World J Urol. 1997. 15:14–20.
3. Griffith OW, Stuehr DJ. Nitric oxide synthases: properties and catalytic mechanism. Annu Rev Physiol. 1995. 57:707–736.
4. Umans JG, Levi R. Nitric oxide in the regulation of blood flow and arterial pressure. Annu Rev Physiol. 1995. 57:771–790.
5. Maines MD. Heme oxygenase: function, multiplicity, regulatory mechanisms, and clinical applications. FASEB J. 1988. 2:2557–2568.
6. Bredt DS, Hwang PM, Glatt CE, Lowenstein C, Reed RR, Snyder SH. Cloned and expressed nitric oxide synthase structurally resembles cytochrome P-450 reductase. Nature. 1991. 351:714–718.
7. Marks GS, Brien JF, Nakatsu K, McLaughlin BE. Does carbon monoxide have a physiologic function? Trends Pharmacol Sci. 1991. 12:185–188.
8. Gräser T, Vedernikov YP, Li DS. Study on the mechanism of carbon monoxide induced endothelium-independent relaxation in porcine coronary artery and vein. Biomed Biochim Acta. 1990. 49:293–296.
9. Furchgott RF, Jothianandan D. Endothelium-dependent and -independent vasodilation involving cyclic GMP: relaxation induced by nitric oxide, carbon monoxide and light. Blood Vessels. 1991. 28:52–61.
10. Brian JE Jr, Heistad DD, Faraci FM. Effect of carbon monoxide on rabbit cerebral arteries. Stroke. 1994. 25:639–643.
11. Kim YC, Davies MG, Marson L, Hagen PO, Carson CC 3rd. Lack of effect of carbon monoxide inhibitor on relaxation induced by electrical field stimulation in corpus cavernosum. Urol Res. 1994. 22:291–293.
12. Ignarro LJ, Bush PA, Buga GM, Wood KS, Fukuto JM, Rajfer J. Nitric oxide and cyclic GMP formation upon electrical field stimulation cause relaxation of corpus cavernosum smooth muscle. Biochem Biophys Res Commun. 1990. 170:843–850.
13. Xue L, Farrugia G, Miller SM, Ferris CD, Snyder SH, Szurszewski JH. Carbon monoxide and nitric oxide as coneurotransmitters in the enteric nervous system: evidence from genomic deletion of biosynthetic enzymes. Proc Natl Acad Sci USA. 2000. 97:1851–1855.
14. Rattan S, Chakder S. Inhibitory effect of CO on internal anal sphincter: heme oxygenase inhibitor inhibits NANC relaxation. Am J Physiol. 1993. 265:G799–G804.
15. McCoubrey WK Jr, Huang TJ, Maines MD. Isolation and characterization of a cDNA from the rat brain that encodes hemoprotein heme oxygenase-3. Eur J Biochem. 1997. 247:725–732.
16. Grozdanovic Z, Gossrau R. Expression of heme oxygenase-2 (HO-2)-like immunoreactivity in rat tissues. Acta Histochem. 1996. 98:203–214.
17. Ingi T, Cheng J, Ronnett GV. Carbon monoxide: an endogenous modulator of the nitric oxide-cyclic GMP signaling system. Neuron. 1996. 16:835–842.
18. Thorup C, Jones CL, Gross SS, Moore LC, Goligorsky MS. Carbon monoxide induces vasodilation and nitric oxide release but suppresses endothelial NOS. Am J Physiol. 1999. 277:F882–F889.
19. Morse D, Sethi J, Choi AM. Carbon monoxide-dependent signaling. Crit Care Med. 2002. 30:1 Suppl. S12–S17.
20. Zakhary R, Gaine SP, Dinerman JL, Ruat M, Flavahan NA, Snyder SH. Heme oxygenase 2: endothelial and neuronal localization and role in endothelium-dependent relaxation. Proc Natl Acad Sci USA. 1996. 93:795–798.
21. Vedernikov YP, Gräser T, Vanin AF. Similar endothelium-independent arterial relaxation by carbon monoxide and nitric oxide. Biomed Biochim Acta. 1989. 48:601–603.
22. Wu L, Cao K, Lu Y, Wang R. Different mechanisms underlying the stimulation of KCa channels by nitric oxide and carbon monoxide. J Clin Invest. 2002. 110:691–700.
23. Tamura M, Kagawa S, Kimura K, Kawanishi Y, Tsuruo Y, Ishimura K. Coexistence of nitric oxide synthase, tyrosine hydroxylase and vasoactive intestinal polypeptide in human penile tissue--a triple histochemical and immunohistochemical study. J Urol. 1995. 153:530–534.
24. White R, Hiley CR. A comparison of EDHF-mediated and anandamide-induced relaxations in the rat isolated mesenteric artery. Br J Pharmacol. 1997. 122:1573–1584.
25. Ewing JF, Raju VS, Maines MD. Induction of heart heme oxygenase-1 (HSP32) by hyperthermia: possible role in stress-mediated elevation of cyclic 3':5'-guanosine monophosphate. J Pharmacol Exp Ther. 1994. 271:408–414.
26. McMillan K, Bredt DS, Hirsch DJ, Snyder SH, Clark JE, Masters BS. Cloned, expressed rat cerebellar nitric oxide synthase contains stoichiometric amounts of heme, which binds carbon monoxide. Proc Natl Acad Sci USA. 1992. 89:11141–11145.
27. Vincent SR, Das S, Maines MD. Brain heme oxygenase isoenzymes and nitric oxide synthase are co-localized in select neurons. Neuroscience. 1994. 63:223–231.
28. Durante W, Kroll MH, Christodoulides N, Peyton KJ, Schafer AI. Nitric oxide induces heme oxygenase-1 gene expression and carbon monoxide production in vascular smooth muscle cells. Circ Res. 1997. 80:557–564.
29. Dwyer BE, Nishimura RN, Lu SY. Differential localization of heme oxygenase and NADPH-diaphorase in spinal cord neurons. Neuroreport. 1995. 6:973–976.
30. Morita T, Kourembanas S. Endothelial cell expression of vasoconstrictors and growth factors is regulated bt smooth muscle cell-derived carbon monoxide. J Clin Invest. 1995. 96:2676–2682.