Abstract
Background
The purpose of this study was to evaluate the usefulness of an accelerometer in predicting body weight (BW) change during a lifestyle intervention and to find out whether exercise or overall physical activity is associated with change in insulin sensitivity and body composition.
Methods
A total of 49 overweight (body mass index [BMI] ≥ 23 kg/m2) women with diabetes were enrolled and performed lifestyle intervention while monitoring BW, total energy expenditure (TEE) and physical activity energy expenditure (PAEE) using an accelerometer, and energy intake (EI) using a three-day dietary record at baseline and every 2 weeks for 12 weeks. We assessed body composition using bioimpedance analysis and compared the actual BW change to the predicted BW change, which was calculated from the energy deficit (ED) between EI and TEE (ED = EI-TEE).
Results
Mean age was 57.2 years, duration of diabetes was 8.0 years, and BMI was 27.8 kg/m2. There was no significant difference between EI and TEE at baseline. For 12 weeks, the ED was 474.0 kcal·day-1, which was significantly correlated with BW change (-3.1 kg) (r = 0.725, P < 0.001). However, the actual BW change was 50% lower than the predicted BW change. Both TEE and PAEE correlated with change in KITT (r = 0.334, P = 0.019; r = 0.358, P = 0.012, respectively), BMI (r = -0.395, P = 0.005; r = -0.347, P = 0.015, respectively), and fat mass (r = -0.383, P = 0.007; r = -0.395, P = 0.005, respectively), but only TEE correlated with fat free mass change (r = -0.314, P = 0.030).
Low physical activity has been shown to be associated with, cardiovascular disease [1], type 2 diabetes mellitus [2], obesity, and chronic ailments [3]. Increased physical activity plays an important role in the prevention and treatment of these diseases [4]. Physical activity is defined as body movement, produced by skeletal muscle [5]. Physical activity does not only include sports, but also includes non-sports activities such as working, leisure activities, and household chores, which can all be expressed as total energy expenditure [6]. Total energy expenditure may be measured through direct measurement methods such as doubly labeled water (DLW) [7] and human calorimeter chambers [8], and also through indirect measurement methods such as the Douglas 100 respiratory chamber, heart rate monitor, pedometer, accelerometer [8], and self-reported questionnaires and activity diaries [9].
Since accelerometers obtain objective information about physical activity patterns and are small and easy to wear, they are effective in evaluating daily total energy expenditure in free living conditions [10]. Most accelerometers showed high correlation with direct measurement methods of energy expenditure during walking, running, and other pedestrian movements [11,12]. Total energy expenditure was measured by accelerometer data has shown very high correlation with the measured value in the chamber during 24 hour period in healthy men and women [8]. In a 14-day study, a DLW method comparison showed a high correlation [13], hereby the validity of accelerometers for monitoring tool that measures total energy expenditure was proven. In several previous studies, total energy expenditure and patterns of physical activity such as duration, frequency, and intensity were used to evaluate through using an accelerometer in free living conditions [14-16]; however, most previous studies have been short-term trials (1-14 days). Long-term interventions and epidemiological studies that measure actual total energy expenditure have been used to examine accelerometer-measured activity, but such studies are limited.
Energy intake surveys most frequently use 24-hour recall methods [17]. In a previous study, comparison studies that used DLW as a direct measurement method and 24-hour recall methods on healthy adults showed a high correlation [18]. The results of their comparison between food frequency questionnaire and 24-hour recall methods showed a high level of correlation on 65-year-olds [19]. However, measured total energy expenditure and energy intake measured by each tool were simultaneously measured long term, and in terms of energy balance, actual weight loss, and predicted weight loss, comparative studies are limited. Data for total energy expenditure is especially limited. Therefore, this study set out to prove the usefulness of accelerometer-measured physical activity for Korean type 2 diabetes mellitus patients regarding the balance of energy. This was done by investigating whether total energy expenditure measured using an accelerometer and energy intake using 24-hour recall methods matched up under conditions in which there were no body weight changes in obese diabetic patients. We investigated whether the difference in energy intake and total energy expenditure could predict actual weight change and compared actual with predicted weight change during long-term intervention periods. Additionally, we investigated which compositions of accelerometer were highly correlated with changes in insulin sensitivity, and body composition which related to insulin sensitivity.
Sixty-one female type 2 diabetes mellitus patients who were overweight or obese, with a body mass index (BMI) greater than 23 kg/m2, and between the ages of 35 to 65 were selected from the Eulji University Hospital Diabetes Center. Patients who had difficulty walking [20], performed aquatic physical activity in which the accelerometers would be hard to attach, performed upper body-focused exercise such as table tennis or resistance exercises on a regular basis [21], would be difficult to monitor for other reasons, or who refused to cooperate with the study were excluded from the study. Also, patients with the following profile were excluded from the study: low socioeconomic status, unable to record a regular activity and dietary diary, poorly educated, and intellectually and developmentally unable to record. Patients who have had a myocardial infarction, stroke, type 1 diabetes mellitus, gestational diabetes, diabetic ketoacidosis, osteoporosis, degenerative arthritis, cancer, or who have uncontrolled high blood pressure (BP > 160/90 mm Hg) were also excluded due to their inability to participate in the exercise program.
Our goal was to measure how well total energy expenditure measured with an accelerometer coincides with energy intake measured using 24-hour recall method under the condition of there being no change in body weight. We also wanted to use a long-term intervention period of weight change to verify whether the difference in energy intake and total energy expenditure can be used to predict actual weight changes, and compare actual weight and predicted weight changes. In our long-term clinical trials, we wanted to evaluate the usefulness of accelerometers as tools for predicting weight change and measuring total energy expenditure in type 2 diabetes mellitus patients.
During the screening period, which took place 2 weeks prior to the beginning of the study, we requested that participants maintain their typical physical activity and dietary intake. As part of the study, they were requested to participate in an exercise and diet program for 12 weeks. Considering that an energy expenditure of 7,700 kcal facilitates a loss of 1 kg, our target weight loss for one month was a body weight reduction of 1-1.5 kg. To increase physical activity energy expenditure by 200 kcals per day, they walked 5 times per week, 30 minutes per day and moderate intensity (3-5 METs) or corresponding to activity level 4 or more recorded by an accelerometer [8]. The diet was intended to reduce dietary intake by 300 kcals. For 12 weeks, patient compliance, energy intake, and total energy expenditure was monitored every two weeks to improve the accuracy of the measurements. Additionally, to find whether total energy expenditure and physical activity energy expenditure measured with the accelerometer were associated with changes in insulin sensitivity and body composition, which reflects insulin sensitivity, we performed an insulin sensitivity test and body composition examination.
Total energy expenditure and physical activity energy expenditure were measured with an accelerometer (Lifecorder®; Susuken Co., Nagoya, Japan). Prior to the study, a trainer gave patients an accelerometer and activity diary to record daily exercise duration, frequency, and mode. Participants entered their age, sex, height, weight, start date of measurements with the accelerometer, and fastened the device to their belts. They were required to wear the accelerometer for 12 weeks, all day long, excluding when they were sleeping and showering. Since accelerometer considers one's age, sex, height, and weight, and automatically calculate one's basal metabolic rate, we re-entered the participant's weight every 2 weeks to account for weight loss caused by the intervention. Among the 11 stages of exercise intensity recorded by the accelerometer, physical activity energy expenditure means higher at intensities above stage 4.
Subjects were required to record their energy intake using a three-day dietary record (two weekdays and one weekend day). Prior to the study, the participants were given simple briefings regarding recording methods. On the form, they were told to record all food consumed on that day, categorized by meal, and noting the amount of food and their ingredients, including health supplements and snacks. When the survey forms were collected, to increase the accuracy of the results, professional nutritionists were consulted throughout the review process, and the CAN-program version 2.0 (computer-aided nutritional analysis program: Korean Nutrition Society, Seoul, Korea) was used for analysis.
Subjects were given medical examinations to asses any illnesses, and their height and weight was also measured. Height and weight were measured while the subjects were wearing a thin layer of clothing; waist size was measured using a tape measure around the thinnest part between bottom of the ribcage and iliac crest, with participants standing in a comfortable position. BMI was calculated in kg/m2. A Bio Impedance Analyzer (Inbody 3.0; Biospace, Seoul, Korea) was used to measure body fat mass, fat free mass, and body fat percentage before and after the study. During body fat analysis, measurements were taken with subjects wearing the lightest possible clothing. Subjects stepped on the electrode plate bare-footed, while holding the electrode units in their hands and lightly pressing the electrodes with their thumbs. Body fat mass and fat free mass were measured in kgs, and body fat percentages were recorded in %.
Insulin sensitivity was measured by the insulin tolerance test, the KITT index (rate constant for plasma glucose disappearance, %/min) [22]. The subjects fasted for longer than 10 hours and visited the clinic on the day of test under fasting conditions. A 20-gauge catheter was inserted into the side of a vein in the hand and used for blood collection. To the contralateral antecubital vein, another 20-gauge catheter was inserted and used for the injection of insulin and the injection of glucose after the completion of tests. Under resting condition, insulin was diluted in advance (Humulin R; Eli Lilly & Co., Indianapolis, IN, USA) to 0.1 U/kg and injected to the antecubital vein and the glucose concentration was measured after 0, 3, 6, 9, 12, and 15 minutes. The measured glucose concentrations over time were input into a computer program, and the date was converted to natural logarithms. The slope of the regression line was calculated using the concentration at 3 to 15 minutes, and the half life was obtained by dividing base blood sugar in half(t1/2). The KITT was calculated as an index of insulin resistance with the following formula:
KITT = 0.619t1/2 * 100 (%/min).
The data from this study was used to calculate means and standard deviations for each category using the statistical program SPSS for Windows, version 15.0 (SPSS Inc., Chicago, IL, USA). Comparisons of before and after mean figures for physiological characteristics, biochemical tests, total energy expenditure, and energy intake over the 12-week period were done using paired t-tests. Comparisons were made between total energy expenditure and energy intake in 2-week increments using paired t-tests, and the correlation of the results were tested using a Bland-Altman plot. Pearson correlation coefficients were used to test correlation between the difference in energy intake and total energy expenditure, change in body weight, BMI and body fat percentage over the 12 week period. To test the correlations of predicted and actual body weight change, total energy expenditure, physical activity energy expenditure, insulin sensitivity, and body composition, Pearson correlation coefficients were calculated. Statistical significance was established as a P value less than 0.05.
Out of a total of 61 patients, 12 (17.4%) were failed during the 12-week study period and a total of 49 female type 2 diabetes mellitus patients were included in the final results. The average age of the participants was 57.2 ± 7.2 years, and the mean duration of diabetes was 8.0 ± 6.1 years. The mean BMI was 27.8 ± 3.0 kg/m2, mean body weight was 67.8 ± 7.7 kg, and the mean glycosylated hemoglobin (HbA1c) level was 7.6 ± 1.2% (Table 1). Prior to the beginning of this study, 34.7% of participants were treated with exercise and diet therapy, 46.9% were treated with hypoglycemic agents (12.2% Sulfonylurea factors, 10.2% metformin, 24.5% sulfonylurea factor/metformin combination therapy), and 18.4% were treated with insulin. Over the 12-week study period, patients taking drugs for the prevention of hypoglycemia had their dosages adjusted by 6.0% (2.0% Sulfonylurea factors, 2.0% metformin, and 2.0% sulfonylurea factor/metformin combination therapy).
In the 14-day screening period, during which there was no change in body weight, the total energy expenditure measured with the accelerometers was 1,908.2 ± 206.3 kcal·day-1 and energy intake measured with three-day dietary record was 1,842.5 ± 236.7 kcal·day-1, resulting in a difference of 65.8 ± 3.3 kcal·day-1 (-1.0 ± 3.3 kcal·day-1·kg-1). This was not considered to be statistically significant. Energy intake and total energy expenditure coincided by 96.5%. Additionally, the confidence interval of the Bland-Altman plot was ± 1.96 SD, with a range of -543.8 to 412.3 kcal·day-1 (-7.5 to 5.5 kcal·day-1·kg-1) (Fig. 1).
The average physical activity energy expenditure before the start of the program using an accelerometer was found to be 310.1 ± 135.4 kcal·day-1. The average daily physical activity energy expenditure during 12 weeks was 393.9 ± 154.8 kcal·day-1, an increase of 83.8 ± 123.4 kcal·day-1. The average total energy expenditure prior to the start of the program was 1,908.2 ± 206.3 kcal·day-1; it was 2,007.3 ± 216.5 kcal·day-1 during 12 weeks, an increase of 83.8 ± 123.5 kcal·day-1.
The average energy intake using with the 3-day dietary record showed 1,842.5 ± 236.7 kcal·day-1 before the start of the program and 1,545.2 ± 247.2 kcal·day-1 during the 12 weeks: a decrease of 297.3 ± 227.3 kcal·day-1 (Table 2). Over 12 weeks, the daily average of the difference between energy intake and total energy expenditure was 474.0 ± 291.9 kcal·day-1 (6.2 ± 4.1 kcal·day-1·kg-1). Weight loss over those 12 weeks was 3.1 ± 2.4 kg, while the difference in the average energy intake and total energy expenditure resulted in a significant correlation with body weight change (r = 0.725, P < 0.001). Additionally, changes in body fat mass and percentage also showed significant correlation (r = 0.605, P < 0.001; r = 0.443, P = 0.002, respectively) (Fig. 2).
The difference in daily average energy intake and total energy expenditure was 474.0 ± 291.9 kcal·day-1 and the actual and predicted reduction in body weight showed significant correlation (r = 0.628, P < 0.001). However, it is known that an energy expenditure of 7,700 kcals results in a 1 kg reduction in body weight. By this measure, the actual weight loss was only 50% of what would be expected (Fig. 3).
After the 12-week experimental period, KITT, BMI, body fat percentage, fat mass, and fat free mass were all significantly decreased (P < 0.001, P < 0.001, P < 0.001, P < 0.001, and P = 0.047, respectively). The variation of KITT appears to have a highly significant correlation with changes in fat free mass and fat mass (r = -0.310, P = 0.032; r = -0.363, P = 0.011). Additionally, both total energy expenditure and physical activity energy expenditure measured with an accelerometer appears to be correlated with changes in KITT (r = 0.334, P = 0.019; r = 0.358, P = 0.012), changes in BMI (r = -0.395, P = 0.005; r = -0.347, P = 0.015, respectively) and changes in body fat mass (r = -0.383, P = 0.007; r = -0.395, P = 0.005, respectively), but there was no correlation with the changes in body fat percentage. However, there was only significant correlation with change in total energy expenditure and fat free mass (r = -0.314, P < 0.030) (Table 3).
Currently, the standard methods to measure total energy expenditure are DLW method and direct calorimetry. The accuracy of their validity is 1-2% [7,23]. Although the DLW method is technique available to accurately measure TEE in daily life, frequency, duration and intensity of physical activity cannot be measured. Also, due to the high cost of oxygen-18, the stable isotope, and analytical devices, it is not economically practical [6]. The most commonly used method for measuring physical activity in clinical trials and research are surveys and self-recorded questionnaires and activity diaries [9]. Although these are used in large-scale epidemiological studies, they take a long time to create, and because they depend on memory, they are less accurate. A previous study developed a questionnaire assay with a total of 32 items and used recollection dates varying from one day to one year. The reliability value when retesting the questionnaire was between 0.24 and 0.93 [9]. When considering that most diabetes patients are elderly, producing an accurate personal history or questionnaire can be very difficult. Additionally, tools used to assess exercise education and patient compliance are considered inappropriate. However, the size of the accelerometers used to measure energy expenditure is small and they make long-term measurements possible. An objective analysis of physical activity patterns such as intensity, time, and frequency of physical activity of the participants is also possible [24]. In previous studies, total energy expenditure measured using accelerometers have shown a very high correlation between comparative results for direct calorimetry (r = 0.928, P < 0.001) [8]. Results compared with the DLW method over 14 days showed a high correlation (r = 0.83, P < 0.001) [13].
In this study, the total energy expenditure measured using an accelerometer was 1,908.2 ± 206.3 kcal·day-1 during the 14-day period where there was no change in body weight. The energy intake measured using a three-day dietary record was 1,842.5 ± 236.7 kcal·day-1, a difference of -65.8 ± 3.3 kcal·day-1, which is not statistically significant. Energy intake was found to be 96.5% of total energy expenditure. Using a questionnaire for total energy expenditure and dietary diary for energy intake, Kim and Cha [25] reported that the surveyed total energy expenditure levels for overweight middle-aged women was 3,256.8 kcal·day-1, while energy intake was 2,080.2 kcal·day-1, which showed a large comparative difference (-1,176.6). However, Tesfaye et al. [26] used a survey to measure total energy expenditure, which was 1,925 kcal·day-1, and a dietary diary to measure energy intake, which was 1,720 kcal·day-1. These values differ by -205 kcal·day-1, which is similar to our results. When there is no change in body weight as a result of energy balance, energy intake and expenditure values are approximately equal. However, in the absence of changes in body weight, Seale [27] found that subjects underestimated the changes in energy intake recorded in a dietary record versus total energy expenditure collected through the DLW method by 25%. Singh et al. [28] also said that their study participants underestimated those values by 23%. The underestimation of energy intake in that investigation was lower than the actual intake because the recorded values were lower than the actual values, and they varied based on weight, obesity, gender, income, and education level [27]. In this study, in the absence of body weight changes, energy intake and total energy expenditure showed a tendency to be underestimated. This is because our subjects were overweight female who want to control their body weight. However, a 5% underestimation is a very low value. Between energy intake and total energy expenditure, there was no significant difference, and the two tools used to investigate and measure energy intake and total energy expenditure in diabetic patients are considered to be appropriate.
Change in body weight, energy intake, and total energy expenditure in the energy balance can be considered related [29]. Energy intake and total energy expenditure are important indicators of blood glucose and body weight regulation because they are necessary in measuring and monitoring accurately. In this study, patients who participated in a long-term weight loss program were later contacted to confirm whether there is an association between change in body weight and difference in energy intake, and total energy expenditure. The results of our 12-week study showed that the difference between average energy intake and total energy expenditure was -474.0 ± 291.9 kcal·day-1, average weight loss was 3.1 ± 2.4 kg, and 13,761 kcals had to be expended in order to lose 1 kg.
A study was performed by Matsuo et al. [30] for 14 weeks to observe the effects of an exercise and diet program on obese adults. The difference between average energy intake and total energy expenditure was -666 kcal·day-1, average weight loss was 6.2 kg, and the energy required to lose 1 kg was 11,279 kcal. Ahn et al. [31] performed a 12-week study to observe the effects of an exercise and diet program on obese adults. In that study, the average energy intake and total energy expenditure difference was -594 kcal·day-1, average weight loss was 5.1 kg, and the energy required to lose 1 kg was 11,658 kcals. In most intervention studies, weight loss based on daily energy deficit had similar result. In this study, the difference in daily average energy intake and total energy expenditure from aspects of energy balance was significantly correlated with the actual change of body weight during 12-week (r = 0.729, P < 0.001). Therefore we infer that accelerometers may be useful tools for diabetic patients in measuring long-term total energy expenditure.
In the current study, the law of Wishnofsky, which states that 7,700 kcals of total energy expenditure results in a 1 kg weight loss, was used to predict weight loss. When estimated weight change and actual weight change were compared, the actual weight loss was 50% lower than the predicted weight loss. In an intervention-study performed by Heymsfield et al. [32] and Goele et al. [33], actual weight loss was 50% lower than the predicted weight loss using the law of Wishnofsky, which is similar to the results of this study. Because fractional energy absorption is higher in obese patients whose weight loss is abrupt than obese patients whose weight is stable even in the same BMI, predicted weight loss was measured to be lower than actual weight loss [34]. Additionally, a Wishnofsky-predicted loss of 1 kg consists of 79% fat mass and 21% fat free mass [35]. In a study performed by Goele et al. [33], 1 kg of weight loss was 87% fat mass and 13% fat free mass, which by the law of Wishnofsky is 50% lower than predicted. Between individuals, the range of fat mass and fat free mass varies greatly (51-98% fat mass and 2-49% fat free mass). Using the laws of Wishnofsky, calculated predicted weight changes and actual weight changes may be different. Because diabetes patients had lower lipid oxidation than healthy subjects who used the same weight management program during exercise [36] and insulin increases body weight [37], an actual body weight decrease is more common than a lower expected weight. Therefore, we infer that further studies are required to determine the energy deficit suitable for producing a 1 kg weight loss in Korean patients. In this study, although actual weight loss was 50% less than predicted, the correlation between actual weight loss and predicted weight loss was good. Using the following formula, actual weight changes can be predicted:
Y (actual weight change, kg) = -0.63 + 0.005 * X (EI - TEE)
In the interest of diabetes prevention and treatment, 30 minutes or more of moderate exercise 3 times per week is recommended [4]. However, recent studies have demonstrated that improvements to insulin resistance can occur as a result of increased low intensity physical activities of daily life [38]; this has been reported to be important for the management of type 2 diabetes mellitus. In this study, both total energy expenditure and physical activity energy expenditure showed significant correlation with change in insulin sensitivity, BMI, and body fat, but total energy expenditure was only significantly correlated with changes in fat free mass.
Starling et al. [39] said that total energy expenditure was related to weight, body fat, and fat free mass. In Seale's study, total energy expenditure and fat free mass had a very high correlation [27], which was also a result found in our study. However, Jacobs et al. [9] showed a correlation between moderate or high intensity activity and BMI. In addition, the duration of moderate or high intensity was also correlated with BMI [40]. The clinical application of the intensity of physical activity is not yet clear. In this study, not only moderate intensity, but also overall physical activity, was found to be associated with insulin sensitivity, and has a high correlation with body fat and fat free mass. For the management of type 2 diabetes mellitus, even an increase in the physical activities of daily life is thought to be important. Limitation of this study was that accelerometer-measured total energy expenditure could not be compared with the DLW as a standard-method, and dual energy X-ray absorptiometry did not use to evaluate the proportion of fat and fat free mass necessary for 1 kg of weight loss. However, in daily life, using an accelerometer as a measuring tool for short-term and long-term total energy expenditure appears to be useful.
Figures and Tables
Fig. 1
Difference between total energy expenditure (TEE) measured by an accelerometer and energy intake (EI) plotted against the mean of the 2 measurements, according to Bland and Altman.
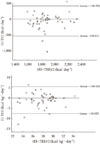
Fig. 2
Correlation between the difference in energy intake (EI) and total energy expenditure (TEE) and actual change of body weight (A), change of fat mass (B), or change of %fat for 12 weeks; %fat (percentage body fat).
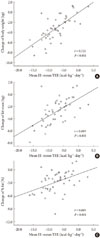
Fig. 3
Correlation between change in actual body weight and predicted body weight change with law of Wishnofsky.
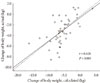
References
1. Fang J, Wylie-Rosett J, Cohen HW, Kaplan RC, Alderman MH. Exercise, body mass index, caloric intake, and cardiovascular mortality. Am J Prev Med. 2003. 25:283–289.
2. Hu FB, Li TY, Colditz GA, Willett WC, Manson JE. Television watching and other sedentary behaviors in relation to risk of obesity and type 2 diabetes mellitus in women. JAMA. 2003. 289:1785–1791.
3. Jebb SA, Moore MS. Contribution of a sedentary lifestyle and inactivity to the etiology of overweight and obesity: current evidence and research issues. Med Sci Sports Exerc. 1999. 31:S534–S541.
4. Pate RR, Pratt M, Blair SN, Haskell WL, Macera CA, Bouchard C, Buchner D, Ettinger W, Heath GW, King AC, Kriska A, Leon AS, Marcus BH, Moris J, Paffenbarger RS Jr, Patrick K, Pollock ML, Rippe JM, Sallis J, Wilmore JH. Physical activity and public health. A recommendation from the Centers for Disease Control and Prevention and the American College of Sports Medicine. JAMA. 1995. 273:402–407.
5. Caspersen CJ, Powell KE, Christenson GM. Physical activity, exercise, and physical fitness: definitions and distinctions for health-related research. Public Health Rep. 1985. 100:126–131.
6. Plasqui G, Westerterp KR. Physical activity assessment with accelerometers: an evaluation against doubly labeled water. Obesity (Silver Spring). 2007. 15:2371–2379.
7. Ravussin E, Harper IT, Rising R, Bogardus C. Energy expenditure by doubly labeled water: validation in lean and obese subjects. Am J Physiol. 1991. 261:E402–E409.
8. Kumahara H, Schutz Y, Ayabe M, Yoshioka M, Yoshitake Y, Shindo M, Ishii K, Tanaka H. The use of uniaxial accelerome try for the assessment of physical-activity-related energy expenditure: a validation study against whole-body indirect calorimetry. Br J Nutr. 2004. 91:235–243.
9. Jacobs DR Jr, Ainsworth BE, Hartman TJ, Leon AS. A simultaneous evaluation of 10 commonly used physical activity questionnaires. Med Sci Sports Exerc. 1993. 25:81–91.
10. Schutz Y, Weinsier RL, Hunter GR. Assessment of free-living physical activity in humans: an overview of currently available and proposed new measures. Obes Res. 2001. 9:368–379.
11. Bouten CV, Westerterp KR, Verduin M, Janssen JD. Assessment of energy expenditure for physical activity using a triaxial accelerometer. Med Sci Sports Exerc. 1994. 26:1516–1523.
12. Levine JA, Baukol PA, Westerterp KR. Validation of the Tracmor triaxial accelerometer system for walking. Med Sci Sports Exerc. 2001. 33:1593–1597.
13. Rafamantanantsoa HH, Ebine N, Yoshioka M, Higuchi H, Yoshitake Y, Tanaka H, Saitoh S, Jones PJ. Validation of three alternative methods to measure total energy expenditure against the doubly labeled water method for older Japanese men. J Nutr Sci Vitaminol (Tokyo). 2002. 48:517–523.
14. Saito N, Yamamoto T, Sugiura Y, Shimizu S, Shimizu M. Lifecorder: a new device for the long-term monitoring of motor activities for Parkinson's disease. Intern Med. 2004. 43:685–692.
15. McClain JJ, Sisson SB, Washington TL, Craig CL, Tudor-Locke C. Comparison of Kenz Lifecorder EX and ActiGraph accelerometers in 10-yr-old children. Med Sci Sports Exerc. 2007. 39:630–638.
16. Crouter SE, Schneider PL, Karabulut M, Bassett DR Jr. Validity of 10 electronic pedometers for measuring steps, distance, and energy cost. Med Sci Sports Exerc. 2003. 35:1455–1460.
17. Lee MS, Woo MK, Kim SA, Oh SI, Kwak CS. Reproducibility of nutrient intake estimated by three-day 24-hour dietary recall of middle-aged subjects for 6 month intervals. Korean J Community Nutr. 2003. 8:603–609.
18. Riumallo JA, Schoeller D, Barrera G, Gattas V, Uauy R. Energy expenditure in underweight free-living adults: impact of energy supplementation as determined by doubly labeled water and indirect calorimetry. Am J Clin Nutr. 1989. 49:239–246.
19. Lee HJ, Park SJ, Kim JH, KIM CI, Chang KJ, Yim KS, Kim KW, Choi HM. Development and validation of a computerized semi-quantitative food frequency questionnaire program for evaluating the nutritional status of the Korean elderly. Korean J Community Nutr. 2002. 7:277–285.
20. Macko RF, Haeuber E, Shaughnessy M, Coleman KL, Boone DA, Smith GV, Silver KH. Microprocessor-based ambulatory activity monitoring in stroke patients. Med Sci Sports Exerc. 2002. 34:394–399.
21. Chan CB, Spangler E, Valcour J, Tudor-Locke C. Cross-sectional relationship of pedometer-determined ambulatory activity to indicators of health. Obes Res. 2003. 11:1563–1570.
22. Bonora E, Moghetti P, Zancanaro C, Cigolini M, Querena M, Cacciatori V, Corgnati A, Muggeo M. Estimates of in vivo insulin action in man: comparison of insulin tolerance tests with euglycemic and hyperglycemic glucose clamp studies. J Clin Endocrinol Metab. 1989. 68:374–378.
23. Schoeller DA, Kushner RF, Jones PJ. Validation of doubly labeled water for measuring energy expenditure during parenteral nutrition. Am J Clin Nutr. 1986. 44:291–298.
24. Hendelman D, Miller K, Baggett C, Debold E, Freedson P. Validity of accelerometry for the assessment of moderate intensity physical activity in the field. Med Sci Sports Exerc. 2000. 32:S442–S449.
25. Kim WY, Cha JY. Energy expenditure in normal-weight and overweight Korean middle-aged women. Nutr Sci. 2001. 4:34–38.
26. Tesfaye F, Byass P, Wall S. Concurrent comparison of energy intake and expenditure among adults in Butajira District, Ethiopia. Public Health Nutr. 2008. 11:675–683.
27. Seale JL. Predicting total energy expenditure from self-reported dietary records and physical characteristics in adult and elderly men and women. Am J Clin Nutr. 2002. 76:529–534.
28. Singh J, Prentice AM, Diaz E, Coward WA, Ashford J, Sawyer M, Whitehead RG. Energy expenditure of Gambian women during peak agricultural activity measured by the doubly-labelled water method. Br J Nutr. 1989. 62:315–329.
29. Brown Myrtle L. Chapter 1, Energy requirements. Nutrition reviews' present knowledge in nutrition. 1990. 6th ed. Washington, DC: The Nutrition Foundation;1–6.
30. Matsuo T, Kim MK, Murotake Y, Numao S, Kim MJ, Ohkubo H, Tanaka K. Indirect lifestyle intervention through wives improves metabolic syndrome components in men. Int J Obes (Lond). 2010. 34:136–145.
31. Ahn HJ, Cho YO, Kwon HR, Ku YH, Koo BK, Han KA, Min KW. The effects of low-calorie diets on abdominal visceral fat, muscle mass, and dietary quality in obese type 2 diabetic subjects. Korean Diabetes J. 2009. 33:526–536.
32. Heymsfield SB, Harp JB, Reitman ML, Beetsch JW, Schoeller DA, Erondu N, Pietrobelli A. Why do obese patients not lose more weight when treated with low-calorie diets? A mechanistic perspective. Am J Clin Nutr. 2007. 85:346–354.
33. Goele K, Bosy-Westphal A, Rumcker B, Lagerpusch M, Muller MJ. Influence of changes in body composition and adaptive thermogenesis on the difference between measured and predicted weight loss in obese women. Obes Facts. 2009. 2:105–109.
34. Heymsfield SB, Smith J, Kasriel S, Barlow J, Lynn MJ, Nixon D, Lawson DH. Energy malabsorption: measurement and nutritional consequences. Am J Clin Nutr. 1981. 34:1954–1960.
35. Wishnofsky M. Caloric equivalents of gained or lost weight. Am J Clin Nutr. 1958. 6:542–546.
36. Ghanassia E, Brun JF, Fedou C, Raynaud E, Mercier J. Substrate oxidation during exercise: type 2 diabetes is associated with a decrease in lipid oxidation and an earlier shift towards carbohydrate utilization. Diabetes Metab. 2006. 32:604–610.
37. Heller S. Weight gain during insulin therapy in patients with type 2 diabetes mellitus. Diabetes Res Clin Pract. 2004. 65:Suppl 1. S23–S27.
38. Healy GN, Wijndaele K, Dunstan DW, Shaw JE, Salmon J, Zimmet PZ, Owen N. Objectively measured sedentary time, physical activity, and metabolic risk: the Australian Diabetes, Obesity and Lifestyle Study (AusDiab). Diabetes Care. 2008. 31:369–371.
39. Starling RD, Matthews DE, Ades PA, Poehlman ET. Assessment of physical activity in older individuals: a doubly labeled water study. J Appl Physiol. 1999. 86:2090–2096.
40. Yoshioka M, Ayabe M, Yahiro T, Higuchi H, Higaki Y, St-Amand J, Miyazaki H, Yoshitake Y, Shindo M, Tanaka H. Long-period accelerometer monitoring shows the role of physical activity in overweight and obesity. Int J Obes (Lond). 2005. 29:502–508.