Abstract
Background
Vascular Endothelial Growth Factor (VEGF) and Advanced Glycation End products (AGEs) have been implicated in the development of diabetic retinopathy. In this study, we examined the expression of VEGF and AGEs in the retina and serum, apoptosis in the retina, and lens opacities in streptozotocin (STZ)-induced diabetic rats.
Methods
The localization of VEGF and AGEs in the retina of STZ-induced diabetic rats was determined by immunohistochemical analysis, and apoptotic cell death was assessed using the TUNEL assay. In the serum, STZ-induced diabetic rats were assayed for VEGF and AGEs by ELISA. Lenses were also isolated to detect the opacity.
Results
Expression of VEGF and accumulation of AGEs were significantly increased in the retinal ganglion cell layers (GCL) and nuclear cell layers (NCL) of STZ-induced diabetic rats compared to normal control rats. In addition to cellular expression, serum VEGF and AGEs levels were also increased significantly in STZ-diabetic rats compared to normal rats (both P < 0.001) and there was a significant correlation between the serum VEGF and AGEs levels (r = 0.504). The lens opaque density of STZ-induced diabetic rats were significantly higher than in normal rats (P < 0.001).
Conclusions
AGEs could be involved in the development of diabetic retinopathy through the induction of VEGF. One could possibly correlate this lens opaque formation with elevation of AGE induced VEGF level. Thus, this study should be considered as a basic research for studying pathology of the retina and lens in diabetic experimental models.
Vascular endothelial growth factor (VEGF) and advanced glycation end products (AGEs) play important roles in the pathogenesis of diabetic retinopathy, and advanced diabetic retinopathy is the leading cause of blindness1-5). The overexpression of VEGF in the retina causes intraretinal and subretinal neovascularization, whereas the inhibition of VEGF expression or activity inhibits retinal neovascularization6). In the extracellular space, as well as within the cytoplasmic compartment of retina cells, AGEs form directly from the reaction of glucose with amino groups7). In vivo and in vitro studies suggest that the elevated AGEs that are present in diabetes may be an important factor in the initiation and progression of retinopathy. In experimental diabetic rats, retinal pericytes accumulate AGEs, and in cultured bovine retinal pericytes, AGEs act to stimulate VEGF expression. Furthermore, AGEs induce apoptotic cell death and neuronal degeneration in retinal explants8,9). The cellular effects of AGEs are mediated by specific receptors, one of which is the receptor for AGEs (RAGE). The presence of RAGE has been demonstrated in all cells related to the micro- and macrovascular complications10,11). The present study was to examine the immunohistological localization and expression of major factors, such as VEGF, AGEs, and RAGE, in the early phase of diabetic retinopathy in streptozotocin (STZ)-induced diabetic rats, 12 weeks after the induction of diabetes. Furthermore, the relationship between serum VEGF levels and increased serum AGEs levels was investigated. Apoptotic cell death in the retina was assessed using the TUNEL assay and the lens opaque density of STZ-induced diabetic rats was also measured.
Healthy male Sprague-Dawley rats (7 weeks old, body weight 200-220 g, Orient Ltd., Seoul, Korea) were housed one animal per cage. The animals were maintained under standard environmental conditions (the temperature was 23 ± 3℃, the relative humidity was 50 ± 10%, and there was analternating 12 h light-dark cycle); standard rodent chowand water ad libitum was provided for the 12-week study period. The experiments were performed according to the United States' National Institutes of Health (NIH) Guide for the Care and Use of Laboratory Animals. The study was approved by the Committee on Animal Care of our institute.
A single dose of STZ (60 mg/kg, i.p) was used to induce type 1 diabetes in the rats. Age-matched normal rats received an equal volume of vehicle (0.01 M citrate buffer, pH 4.5). Two days after the injection, the blood glucose levels were measured using blood samples obtained from the tail vein rats with a blood glucose level over 300 mg/dL were labeled as STZ-induced diabetic rats.
Drinking water and food consumption per 24 h were measured once a month. One day before autopsy, the animals were fasted for at least 15 h, and they were then anesthetized with ethyl ether. The final body weight and eyeball weight were measured immediately before blood collection. The fasting serum glucose was determined using the hexokinase method12).
The eyes were collected for histopathologic examination 12 weeks after the induction of diabetes. In brief, the eyes were preserved in 4% paraformaldehyde at room temperature for 24 h, embedded in paraffin, and sectioned (3 µm). Paraffin sections were deparaffinized, hydrated with water, and then stained with hematoxylin and eosin as a counterstain. For double-immunofluorescence AGEs (6D12, TransGenic Inc., Kumamoto, Japan) and RAGE (Santa Cruz Biotechnology, Santa Cruz, CA) staining, formalin-fixed paraffin embedded section cuts were mounted on slides, baked for 3 h at 58℃, deparaffinized, rinsed with 3% hydrogen peroxide, and then incubated with proteinase K (0.5 mg/mL) for 5 min at room temperature. These sections were washed with rinse buffer and blocked with PBS containing 2% normal horse serum for 5 min; they were subsequently incubated with 6D12 anti-AGEs mouse monoclonal antibody (1:250). Then, the sections were washed three times with PBS containing 1% normal horse serum and rabbit anti-RAGE antibody (1:200) for 30 min at room temperature. After washing with PBS, the sections were incubated with FITC-labeled anti-mouse IgG (1:200) and Texas red-labeled anti-rabbit IgG (1:200) for 30 min at room temperature. Finally, immunoreactivity was examined under an Olympus fluorescence microscope (Japan).
Total RNA was isolated using TRIzol (MCRC, Cincinnati, OH) reagent according to the manufacturer's instructions. cDNA was synthesized with g of RNA using RT-primix (Bioneer, Korea). The upstream and downstream primers for rat VEGFmRNA were 5'-AAC CAT GAA CTT TCT GCT CTC TTG-3' and 5'-GCC TGG CTC ACC GCC TTG GCT TGT C-3', yielding a 398-bp product. On the other hand, those for β-actin, used as an internal control, were 5'-CGT AAA GAC CTC TAT GCC AA-3' and 5'-AGC CAT GCC AAA TGT GTC AT-3', yielding a 350-bp product. RNA was similarly reverse transcribed by Takara PCR Thermal Cycler (Japan). The RT-PCR products were separated by electrophoresis and DNA band intensities in agarose gels and quantitated with densitometry (Las-3000, Fujiphoto, Tokyo, Japan).
The terminal deoxynucleotidyl transferase-mediated biotinylated UTP nick end labeling (TUNEL) reaction was done along with DAPI staining. The protocol was adapted from Gavrieli et al.11) The prepared tissue sections were deparaffinized in xylene for 10 min and hydrated through a graded ethanol series. The TUNEL assay was then carried out according to the manufacturer's (Roche Diagnostics, Meylan, France) instructions; the samples were stained with DAPI solution after the TUNEL reaction.
The serum AGEs concentrations were measured using the indirect ELISA protocol. The serum VEGF levels were also measured using the commercially available VEGF Quantikine ELISA Kit from R&D systems (Minneapolis, MN). Briefly, 96-well microplates were coated with serum in 0.1 mol/L sodium carbonate buffer (pH 9.5) for 2 h at room temperature. The wells were blocked for 1 h with PBS containing 1% FBS and 0.05% Tween 20. Rabbit anti-AGEs antibodies were diluted at a titer of 1:3,000 in PBS and incubated for 1.5 h at 37℃; this was followed by washing. An alkaline phosphatase-conjugated antibody to rabbit IgG was then added as the secondary antibody at a titer of 1:3,000 in PBS and incubated for 1 h at 37℃. After the washing steps were repeated three times, the wells were developed with p-nitrophenyl phosphate substrate solution (pH 10.4). The reaction was terminated by adding 1 M sulfuric acid (50 µg/well) and the absorbance at 450 nm was read on a microplate reader (Synergy HT, BIO-TEK).
The lenses were excised from the eyeball under an optical microscope, then transferred onto 24-well plates, each containing 2 mL of saline solution. They were then photographed under an optical microscope with a CCD camera. The opaque area of the lens was analyzed using an imaging system program (Image analysis 42D 3D measuring software, TDI Scope Eyemedia 3.0, Olysia, Japan). The data are expressed as the percentage of opaque area compared to the entire lens area.
Twelve weeks after the induction of diabetes by STZ, there was a significant difference between the normal rats and the diabetic rats in body weight, eyeball weight, and serum glucose levels. The intakes of water and food were markedly increased in the diabetic rats (Table 1).
Twelve weeks after the induction of diabetes, the changes in the thickness of the retinal layers were not marked in the STZ-induced diabetic rats (Fig. 1A). Figure 1B shows VEGF-positive cells detected by immunohistochemistry in the retinas of the diabetic and the normal control rats. Very few VEGF-positive spots were present in the ganglion cell layers (GCL) and inner nuclear layers (INL) in the normal control rat retina. However, the VEGF-positive area was increased in the GCL and INL of the diabetic rats (Fig. 1B, b' and b''). The VEGF mRNA: β-actin mRNA ratio in STZ-diabetic rats was increased 2.57 fold (P < 0.01) compared with control rats (Fig. 1D). Serum VEGF levels were also significantly increased in the diabetic rats compared to the normal control rats (Fig. 1E).
The localization of AGEs and RAGE in the retinas was examined using double-immunofluorescence staining (Fig. 2A). The immunoreactivity of AGEs in retinal GCL (indicated by arrows) and INL (indicated by arrowheads) was increased in the retina of the diabetic rats (Fig. 2A, b) compared to the normal control rats (Fig. 2A, a). However, there was no significant difference in RAGE expression between the two groups (Fig. 2A, a' and b'). Serum AGEs levels were significantly increased in diabetic rats compared to normal control rats (Fig. 2B).
To examine the correlation between serum VEGF and AGEs levels, serum was collected from normal control rats and diabetic rats, and the levels were measured using ELISA. Figure 3 shows that there was a highly significant correlation between serum VEGF and AGEs levels (p=0.012) in both normal control and STZ-induced diabetic rats. The present study is the first to report that there is significant correlation between serum VEGF and AGEs levels in normal control and STZ-induced diabetic rats (r = 0.504).
To identify apoptotic cell death in the retina affected by diabetes, whole retinas were reacted with TUNEL. Twelve weeks after the induction of diabetes, TUNEL -positive cells were observed in the GCL, and a large number of apoptotic ganglion cells were present in the retinas of STZ-induced diabetic rats (Fig. 4b).
The onset of cataract was observed macroscopically after 7 weeks. Seven to eight weeks after the induction of diabetes by STZ injection, all lenses showed cataract formation. These results suggest that STZ-induced diabetic rats at 9~10 weeks after STZ-injection are suitable for screening drugs for the treatment of experimental diabetic cataract (Fig. 5).
Our results demonstrated that expression of VEGF and accumulation of AGEs were significantly increased in the GCL and NCL of STZ-induced diabetic rats compared to normal control rats and a large number of apoptotic ganglion cells were present in the retinas of STZ-induced diabetic rats. In addition, serum VEGF and AGEs levels were also increased significantly in STZ-diabetic rats and there was a significant correlation between the serum VEGF and AGEs levels. The lens opaque density of STZ-induced diabetic rats was significantly higher than in normal rats.
Among the growth factors involved in diabetic retinopathy, VEGF is the major mediator of retinal angiogenesis and is a 46 kDa homodimer glycoprotein with vasopermeability5). VEGF binds to cell surface tyrosine kinase receptors, such as VEGFR-1 (Flt-1) and VEGFR-2 (kinase domain region, KDR or Flk-1). A significant number of studies have already reported the upregulation of VEGF in the retinas of experimental diabetic rats and in the vitreous fluid of patients with proliferative diabetic retinopathy4,5,18,19). Because of the potential role of VEGF in the development and progression of diabetic retinopathy, elevated serum VEGF levels can affect pathological angiogenesis and increase vascular permeability, resulting in eye disease, such as diabetic retinopathy and cataracts.
AGEs are a heterogeneous group of products obtained from the non-enzymatic glycation and oxidation of proteins and lipids; they accumulate under various biological conditions, such as diabetes, inflammation, renal failure, and aging3,4). The interaction of AGEs with their cellular receptors (RAGE) plays an important role in the pathogenesis of diabetic complications. In particular, molecular mechanisms of diabetic retinopathy are mediated by the AGE-RAGE interaction15). A slightly increased RAGE expression has been observed in the retinal vessels of hyperglycemic and hyperlipidemic mice16). Hyperlipidemia is related to the severity of diabetic retinopathy17). AGEs induce VEGF expression in cell culture and animal models, being considered to be involved in development of diabetic retinopathy8,18,19). Our previous study showed that high glucose or AGEs induce VEGF protein and mRNA expression in human retinal pigment epithelial cells14). The vitreous levels of AGEs and VEGF are significantly higher in patients with diabetic retinopathy than in control subjects and there is a significant correlation between the vitreous AGEs and VEGF levels18,19). Recent study provides evidence that VEGF not but inflammatory factors such as IL-6, the cell adhesion molecules intercellular adhesion molecule (ICAM) and vascular cell adhesion molecule (VCAM) play a role in the pathogenesis of diabetic retinopathy20). AGEs, toxicity is directly responsible for retinal neurodegeneration, and therefore, contributes to the early pathological mechanisms of diabetic retinopathy8,9).
A recent report showed that AGEs levels in diabetic cataractous lenses increased compared with senile cataractous lenses and clear lenses21). The relationship between the lenses' AGEs content and opacity indicates that advanced glycation plays a pivotal role in cataract formation22). AGEs inhibitor such as pyridoxamine inhibit AGEs formation in diabetic lens23). This result suggest that the visual loss associated with diabetic retinopathy and cataract could be attributed to increased serum VEGF and AGEs levels in STZ-induced diabetic rats. Furthermore, STZ-induced diabetic rats could be used as an animal model for studying pathology of retina and lens, and for testing candidate drugs for the treatment of diabetes-related ocular disease, especially lens opacity.
Expressions of VEGF and AGEs were increased in the GCL and INL of the retina in STZ-induced diabetic rats. Serum VEGF and AGEs levels were also increased in STZ-induced diabetic rats, and the serum VEGF and AGEs levels showed a strong correlation. Twelve weeks after the induction of diabetes by STZ injection, retinal GCL apoptosis and lens cataracts were observed.
Figures and Tables
Fig. 1
Expression of VEGF in the retina and the expression of VEGF in the serum of STZ-induced diabetic rats. A. Hematoxylin and eosin staining (a, normal rats; b, diabetic rats). B. VEGF staining: The brown product of the peroxidase reaction is present in the GCL (a' and b') and in the INL (a'' and b'') after VEGF staining of the retinal sections. (n = 4 retinas per group). Scale bar = 50 µm. C. Distribution of VEGF immunostaining score in the retina: Each sample was scored from 0 to 5 based on the intensity of the positive area with VEGF immunostaining. Results are given as mean ± S.E.M. D. Expression of VEGF mRNA in the retina was quantified by a RT-PCR method. Results are given as mean ± S.E.M. (n = 6). E. Serum VEGF levels: Each dot represents the serum VEGF levels determined by ELISA for each animal. N, normal (n = 12); STZ-DM, STZ-induced diabetic rats (n = 12).
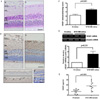
Fig. 2
Localization of AGEs and RAGE in the retina and expression of AGEs in the serum of STZ-induced diabetic rats. A. AGEs and RAGE double-immunofluorescence staining of STZ-induced diabetic rat retina. The sections were immunolabeled with anti-AGE antibody (a and b) and then RAGE antibody (a' and b'). Fluorescein (a and b) and Texas red (a' and b') show identical fields, respectively. Cell bodies showing AGEs immunoreactivity are present in the GCL and INL; The retinas of normal rats (a, a', and a'') and of STZ-induced diabetic rats (b, b', and b''). Scale bar = 50 µm. B. Serum AGEs levels. Each dot represents the serum AGEs levels determined by ELISA for each animal. The serum AGEs levels of STZ-induced diabetic rats are significantly higher than those of normal control rats. N, normal (n = 12); STZ-DM, STZ-induced diabetic rats (n = 12).
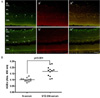
Fig. 3
Correlation between serum VEGF and AGEs levels. A strong correlation (r = 0.504) between serum VEGF levels measured by ELISA and serum AGEs levels was found in both normal rats (n = 12) and STZ-induced diabetic rats (n = 12).
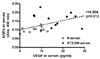
Fig. 4
Retinal cell apoptosis detected by TUNEL and DAPI staining. (A) The retinas of normal control rats (a, a', and a'') and STZ-induced diabetic rats (b, b', and b''): apoptotic retinal ganglion cell death was detected by TUNEL (green), and the nuclei (blue) were stained with DAPI. Merged images (a'' and b'') of TUNEL and DAPI. Scale bar = 50 µm. The number of TUNEL-positive cells was increased compared to that of normal control retinas (B) as indicated in the graph.
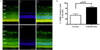
Fig. 5
Cataractogenesis in the lenses of STZ-induced diabetic rats. A. Images of rat lenses in normal and STZ-induced diabetic rats. B. Lens opacities. All lens opacities were analyzed. N, normal rats; DM, STZ-induced diabetic rats. Since the opacity changes associated with diabetes are usually asymmetrical, the eyes from each animal were always analyzed independently. All data are expressed as means ± S.E.M. (n = 14).
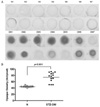
Acknowledgements
This research was supported by a grant [L07010] from the Korea Institute of Oriental Medicine.
References
1. Gardiner TA, Archer DB, Curtis TM, Stitt AW. Arteriolar involvement in the microvascular lesions of diabetic retinopathy: implications for pathogenesis. Microcirculation. 2007. 14:25–38.
2. Stitt AW. The role of advanced glycation in the pathogenesis of diabetic retinopathy. Exp Mol Path. 2003. 75:95–108.
3. Yamagishi S-I, Nakamura K, Matsui T. Advanced glycation end products (AGEs) and their receptor (RAGE) system in diabetic retinopathy. Curr Drug Discov Technol. 2006. 3:83–88.
4. Masuzawa K, Jesmin S, Maeda S, Zaedi S, Shimojo N, Miyauchi T, Goto K. Effect of endothelin dual receptor antagonist on VEGF levels in streptozotocin-induced diabetic rat retina. Exp Biol Med (Maywood). 2006. 231:1090–1094.
5. Witmer AN, Vrensen GFJM, Van Noorden CJF, Schlingemann RO. Vascular endothelial growth factors and angiogenesis in eye disease. Prog Retin Eye Res. 2003. 22:1–29.
6. Aiello LP, Pierce EA, Foley ED, Takagi H, Chen H, Riddle L, Ferrara N, King GL, Smith LE. Suppression of retinal neovascularization in vivo by inhibition of vascular endothelial growth factor (VEGF) using soluble VEGF-receptor chimeric proteins. Proc Natl Acad Sci USA. 1995. 92:10457–10461.
7. Stitt AW, Curtis TM. Advanced glycation and retinal pathology during diabetes. Pharmacol Rep. 2005. 57:156–168.
8. Yamagishi S, Amano S, Inagaki Y, Okamoto T, Koga K, Sasaki N, Yamamoto H, Takeuchi M, Makita Z. Advanced glycation end products-induced apoptosis and overexpression of vascular endothelial growth factor in bovine retinal pericytes. Biochem Biophys Res Commun. 2002. 290:973–978.
9. Lecleire-Collet A, Tessier LH, Massin P, Forster V, Brasseur G, Sahel JA, Picaud S. Advanced glycation end products can induce glial reaction and neuronal degeneration in retinal explants. Br J Ophthalmol. 2005. 89:1631–1633.
10. Stitt AW, Li YM, Gardiner TA, Bucala R, Archer DB, Vlassara H. Advanced glycation end products (AGEs) co-localize with AGE receptors in the retinal vasculature of diabetic and of AGE-infused rats. Am J Path. 1997. 150:523–531.
11. Gavrieli Y, Gavrieli Y, Ben-Sasson SA. Identification of programmed cell death in situ via specific labeling of nuclear DNA fragmentation. J Cell Biol. 1992. 119:493–501.
12. Westwood A, Bullock DG, Whitehead TP. An examination of the hexokinase method for serum glucose assay using external quality assessment data. Ann Clin Biochem. 1986. 23:92–96.
13. Obrosova IG, Drel VR, Kumagai AK, Szabo C, Pacher P, Stevens MJ. Early diabetes-induced biochemical changes in the retina: comparison of rat and mouse models. Diabetologia. 2006. 49:2525–2533.
14. Kim YS, Jung DH, Kim NH, Lee YM, Jang DS, Song G-Y, Kim JS. KIOM-79 inhibits high glucose or AGEs-induced VEGF expression in human retinal pigment epithelial cells. J Ethnopharmacol. 2007. 112:166–172.
15. Yamagishi S, Nakamura K, Matsui T. Advanced glycation end products (AGEs) and their receptor (RAGE) system in diabetic retinopathy. Curr Drug Discov Technol. 2006. 3:83–88.
16. Barile GR, Pachydaki SI, Tari SR, Lee SE, Donmoyer CM, Ma W, Rong LL, Buciarelli LG, Wendt T, Horig H, Hudson BI, Qu W, Weinberg AD, Yan SF, Schmidt AM. The RAGE axis in early diabetic retinopathy. Invest Ophthalmol Vis Sci. 2005. 46:2916–2924.
17. Chew EY, Klein ML, Ferris FL III, Remaley NA, Murphy RP, Chantry K, Hoogwerf BJD, Miller D. Association of elevated serum lipid levels with retinal hard exudate in diabetic retinopathy. Early Treatment Diabetic Retinopathy Study (ETDRS) Report 22. Arch Ophthalmol. 1996. 114:1079–1084.
18. Kaji Y, Usui T, Ishida S, Yamashiro K, Moore TC, Moore J, Yamamoto Y, Yamamoto H, Adamis AP. Inhibition of diabetic leukostasis and blood-retinal barrier breakdown with a soluble form of a receptor for advanced glycation end products. Invest Ophthalmol Vis Sci. 2007. 48:858–865.
19. Yokoi M, Yamagishi SI, Takeuchi M, Ohgami K, Okamoto T, Saito W, Muramatsu M, Imaizumi T, Ohno S. Elevations of AGE and vascular endothelial growth factor with decreased total antioxidant status in the vitreous fluid of diabetic patients with retinopathy. Br J Ophthalmol. 2005. 89:673–685.
20. Yokoi M, Yamagishi S, Takeuchi M, Matsui T, Yoshida Y, Ohgami K, Amano-Okamoto T, Ohno S. Positive correlation between vitreous levels of advanced glycation end products and vascular endothelial growth factor in patients with diabetic retinopathy sufficiently treated with photocoagulation. Br J Ophthalmol. 2007. 91:397–398.
21. Meleth AD, Agrn E, Chan CC, Reed GF, Arora K, Byrnes G, Csaky KG, Ferris FL 3rd, Chew EY. Serum inflammatory markers in diabetic retinopathy. Invest Ophthalmol Vis Sci. 2005. 46:4295–4301.
22. Zarina S, Zhao HR, Abraham EC. Advanced glycation end products in human senile and diabetic cataractous lenses. Mol Cell Biochem. 2000. 210:29–34.
23. Franke S, Dawczynski J, Strobel J, Niwa T, Stahl P, Stein G. Increased levels of advanced glycation end products in human cataractous lenses. J Cataract Refract Surg. 2003. 29:998–1004.
24. Padival S, Nagaraj RH. Pyridoxamine Inhibits Maillard Reactions in Diabetic Rat Lenses. Ophthalmic Res. 2006. 38:294–302.