Abstract
Background
Mucus hypersecretion from airway epithelium is a characteristic feature of airway inflammatory diseases. Tumor necrosis factor α (TNF-α) regulates mucin synthesis. Glucocorticoids including mometasone fuorate (MF) have been used to attenuate airway inflammation. However, effects of MF on mucin production have not been reported.
Methods
Effects of MF and budesonide (BUD) on the phorbol-12-myristate-13-acetate (PMA)–induction of mucin and TNF-α in human airway epithelial cells (NCI-H292) were investigated in the present study. Confluent NCI-H292 cells were pretreated with PMA (200 nM) for 2 hours. Subsequently, the cells were stimulated with MF (1–500 ng/mL) or BUD (21.5 ng/mL) for 8 hours. Dexamethasone (1 µg/mL) was used as the positive control. Real-time polymerase chain reaction was used to determine MUC2 and MUC5AC mRNA levels. The level of total mucin, MUC2, MUC5AC, and TNF-α in culture supernatants were measured using enzyme-linked immunosorbent assay.
Inflammatory diseases of the respiratory tract including allergic rhinitis, asthma, and rhinosinusitis are among the most prevalence conditions to affect the general population12. Many steroids including budesonide (BUD), fluticasone (FP), and mometasone fuorate (MF) have been used to treat rhinosinusitis3 and asthma4. Excessive mucin secretion is a hallmark of the pathogenesis of several airway diseases, including asthma and rhinosinusitis. Overexpression of MUC5AC and MUC5B has reported in chronic rhinosinusitis56. In addition to an increase in MUC5AC and MUC5B, MUC2 overexpression was described in asthma7. Despite wide use of steroids, their role is still controversial in many pulmonary conditions, especially regarding to mucin secretion. BUD suppressed interleukin-1β up-regulated MUC2 and MUC5AC synthesis in human airway epithelial cells (NCI-H292)8. FP repressed MUC5AC mRNA expression or MUC5AC protein production in NCI-H292 stimulated with transforming growth factor α9. However, a previous study in chronic rhinosinusitis patients with nasal polyposis demonstrated that intranasal FP had no effect on MUC5AC gene and protein expression in nasal polyps10. Although many articles show that MF exhibits the high potency compared to other steroid drugs, there appear to be no reports of the effects of MF on mucin production11.
Tumor necrosis factor α (TNF-α) has been implicated in the pathophysiologic mechanisms of airway inflammatory diseases, including chronic rhinosinusitis12 and asthma13. However, the effect of steroids on TNF-α is not clear. BUD significantly reduced lipopolysaccharide (LPS)-induced TNF-α production from lymphocytes14. MF suppressed the release of TNF-α in nasal lavage of patients with allergic rhinitis15. In contrast, TNF-α expression in nasal polyps of patients with chronic rhinosinusitis was unaffected by FP10.
Therefore, the aim of our study was to investigate the effect of MF on mucin and TNF-α production in human airway epithelial cells, compared to that of BUD. NCI-H292 cells was used a model for studies of mucin synthesis. Phorbol-12-myristate-13-acetate (PMA) was used as an inflammatory stimulant. Our findings show that MF and BUD can down-regulate MUC5AC and MUC2 gene induction and mucin protein production by PMA. The inhibitory effect of MF and BUD on TNF-α production was also demonstrated in the study.
All chemicals and reagents used in the study were obtained from Sigma-Aldrich (St. Louis, MO, USA) unless otherwise specified. Mometasone fuorate monohydrate (Nasonex) was obtained from Merck Sharp & Dohme Corp. (Kenilworth, NJ, USA). The drug was dissolved in distilled water. BUD and dexamethasone (DEX) were dissolved in dimethyl sulfoxide (DMSO). The final concentration of DMSO in the medium was less than 0.1%.
Cytotoxicity of MF and BUD was measured using the 3-(4,5-dimethylthiazol-2-yl)-2,5-diphenyltetrazolium bromide (MTT) colorimetric assay. NCI-H292 cells were maintained in RPMI 1640 medium supplemented with 10% fetal calf serum, penicillin (100 U/mL) and streptomycin (100 µg/mL) at 37℃ in a humidified atmosphere of 95% air and 5% CO2. NCI-H292 cells were grown until they reached 80% confluence, they were then plated into 96-well culture plates at a density of 5,000 cells/well in a total volume of 100 µL and allowed to attach and grow for 24 hours. The supernatant in each well was then replaced with RPMI 1640 medium containing various concentrations of MF (0, 1, 10, 50, and 100 µg/mL) and BUD (0 µg/mL, 4.3 µg/mL [10 nM], 21.5 µg/mL [50 nM], 43 µg/mL [100 nM], 86 µg/mL [200 nM], and 172 µg/mL [400 nM]). After 72 hours of incubation, 100 µL of MTT (Sigma-Aldrich) was added to each well. After 4 hours incubation, the supernatant was removed and 150 µL DMSO (Sigma-Aldrich) was added to each well. Samples were then shaken for 15 minutes to dissolve the dark blue crystals. The absorbance of each well was read at 570 nm using an enzyme-linked immunosorbent assay (ELISA) microplate reader (Sunrise; Tecan, Salzburg, Austria). All experiments were performed in triplicate. Cell cytotoxicity was calculated as in Eq. (1). , where Ca is the absorbance of untreated cells (control) and Ta is the absorbance of treated cells.
NCI-H292 cells were maintained in the complete RPMI 1640 medium as mentioned before. When cultures were confluent, the cell were incubated with RPMI 1640 medium containing 0.5% fetal calf serum for 24 hours, after which they were rinsed with phosphate buffered saline (PBS) and exposed to the indicated concentrations of BUD or MF for 2 hours before exposed to PMA (200 nM) for 8 hours. In case of controls, the cells were incubated with medium alone for the same times. DEX has been reported to inhibit mucin expression in NCI-H292 cells9. Therefore, it was used as a positive control for inhibition.
Conditioned media were collected for the determination of total mucin, MUC2, MUC5AC, and TNF-α levels. Total RNA was extracted for measuring the mRNA expression of MUC2 and MUC5AC genes by real-time reverse transcription polymerase chain reaction (RT-PCR).
Total RNA was isolated from cells using the Trizol reagents (Gibco-BRL, Rockville, MD, USA) according to the manufacturer's instruction. cDNA was synthesized from 1 µg of RNA using the Primer RT-PCR Premix (2×; Genet BIO, Daejeon, Korea). Real-time RT-PCR TagMan assays were used to determine MUC2 and MUC5AC expression. Sequences of primers and TagMan probes (Table 1) were designed and used as reported previously1617. Real-time PCR was performed using an IQ5 Multicolor Real-time PCR Detection System (Bio-Rad Laboratories, Hercules, CA, USA) with gene-specific primers and FastStart Universal Probe Master (Rox; Roche Diagnostic GmbH, Mannheim, Germany). RNA expression levels were calculated using the comparative cycle threshold (Ct) method18. Quantification of relative mRNA expression levels was determined and normalized to a constitutive expression of glyceraldehyde 3-phosphate dehydrogenase mRNA.
MUC2 levels in conditioned media were measured by sandwich ELISA 19. Briefly, a monoclonal anti-human MUC2 (sc 59956; Santa Cruz Biotechnology, Santa Cruz, CA, USA) at 1:5,000 in a blocking buffer (1% gelatin in PBS-Tween) was incubated at 4℃ overnight in a 96-well plate. After washing with PBS-Tween, the plate was blocked with the blocking buffer at 37℃ for 1 hour. Following washing with PBS-Tween, samples were added to each well and the plate was incubated at 37oC for 2 hours. After washing with PBS-Tween, the plate was incubated with horseradish peroxidase (HRP)–conjugated Helix pomatia (5 µg/mL in the blocking buffer) for 1 hour at 37℃. Color was developed with tetramethybenzidine (TMB) solution, and the reaction was stopped by adding 0.5 M hydrochloric acid. Absorbance was read at 450 nm.
MUC5AC protein was determined by ELISA20. Each sample was incubated at 37℃ in a 96-well plate until dry. After washing with PBS-Tween, the plate was blocked with a blocking solution (1% gelatin in PBS-Tween) at 37℃ for 1 hour. After incubation with monoclonal anti-human MUC5AC (clone 45M1; Neomarkers, Fremont, CA, USA) at 37℃ for 2 hours, the plate was washed and incubated with HRP-conjugated goat anti mouse antibody (Pierce, Rockford, IL, USA) at 37℃ for 2 hours. TMB solution was added to develop color. After the reaction is stopped, the absorbance of each well was read at 450 nm using an ELISA microplate reader (Sunrise; Tecan). The expression of MUC2 and MUC5AC proteins was presented as absorbance values (arbitrary unit) since there are no commercial standards available for human MUC2 and MUC5AC at present.
An enzyme-linked lectin assay was used to determine the mucin-like glycoprotein secretion as previously described19. Briefly, wells of a 96-well plate were coated with samples or standard (bovine submaxillary gland mucin, diluted in sodium carbonate buffer [0.05M, pH 9.6]; Sigma-Aldrich). The plate was incubated overnight at 4oC, washed with PBS containing 0.05% Tween 20 (PBS-Tween), and blocked with a blocking buffer (1% gelatin in PBS-Tween) for 2 hours at 37oC. After washing with PBS-Tween, the plate was incubated with 5 µg/mL HRP-conjugated Helix pomatia lectin in a blocking buffer for 2 hours at 37oC. Following washing with PBS-Tween, the plate was incubated with the peroxidase substrate, TMB solution, at room temperature. The reaction was stopped after 10 minutes by the addition of 0.5 M hydrochloric acid. The absorbance of each well was read at 450 nm using an ELISA microplate reader (Sunrise; Tecan). The concentration of total mucin was calculated by comparison with standards.
TNF-α levels in culture supernatant were determined by High Sensitive ELISA Kit (eBioscience, San Diego, CA, USA), according to the manufacturer's recommendations.
Statistical analyses were conducted using GraphPad Prizm 5 (GraphPad Software, San Diego, CA, USA). Data were presented as mean±standard error of the mean from three independent experiments. Statistical comparisons among groups were performed by one-way ANOVA, followed by Dunnett's post hoc test. Significance was set to p≤0.05.
To test the potential cytotoxicity of MF and BUD at different concentrations, we used MTT assays to examine the viability of NCI-H292 cells and the results are shown in Figure 1. NCI-H292 cells were exposed to MF (0–100 µg/mL) or BUD (0–172 µg/mL) for 72 hours. MF (Figure 1A) had little effect on the viability of NCI-H292 cells when the concentration was 1 µg/mL; however, when the concentration was higher than 1 µg/mL, cell viability decreased with increased MF concentrations. When the concentration was above 10 µg/mL, cell viability markedly decreased to less than 20%. There was no cytotoxic of BUD to NCI-H292 cells at concentrations up to 172 µg/mL (Figure 1B). The maximum non-toxic dose of MF and BUD provided the basis for the dose selection for further experiments in our study.
PMA (200 nM) increased the expression of MUC2 and MUC5AC gene expression in NCI-H292 cells (Figures 2, 3). This effect of PMA was significantly inhibited by pretreatment of cells with BUD (21.5 µg/mL). MF (1–500 ng/mL) exhibited inhibitory effects on the expression of MUC2 and MUC5AC genes (p<0.05).
To verify the effect of MF and BUD on mucin production, the effects of these steroids on MUC2 and MUC5AC protein expression were evaluated in NCI-H292 cells stimulated by PMA. We demonstrated that MF at 1–500 ng/mL suppressed MUC2 and MUC5AC induced by PMA in a dose-dependent manner (p<0.05) (Figures 4, 5). The stimulating effect of PMA was significantly inhibited with BUD at 21.5 µg/mL (p<0.05).
Treatment NCI-H292 cells with PMA stimulated total mucin production (Figure 6). This confirms that we can use PMA as an inflammatory stimulant in our model. BUD (21.5 µg/mL) and MF (1–500 ng/mL) significantly inhibited the upregulatory effect of PMA (p<0.05).
Figure 7 shows that MF at 1–500 ng/mL inhibited PMA-induced TNF-α production from NCI-H292 cells in a dose-response fashion (p<0.05). BUD at 21.5 µg/mL significantly suppressed the stimulating effect of PMA on TNF-α synthesis in NCI-H292 cells.
Previous studies have revealed that normal human bronchial epithelial (NHBE) and NCI-H292 cells share key components of the signaling pathways downstream of epidermal growth factor receptor, which are responsible for mucin production21. Furthermore, it has been demonstrated that PMA could enhance MUC5AC mRNA and protein expression in both NHBE and NCI-H292 cells22. Therefore, we assumed that NCI-H292 cells would provide a valid model of mucin production in normal cells, and thus, focused on investigating the effect of steroid drugs on mucin production using NCI-H292 cells.
Glucocorticoids act via the glucocorticoid receptor, which resides in the cytoplasm. MF exhibits a very high affinity for the glucocorticoid receptor, which is one explanation for the high potency compared to other steroid drugs11. However, the effect of this drug on mucin production is still unknown. To investigate this effect, we used PMA, a widely used stimulant known to increase mucin production in airway epithelial cells. We show that PMA increase the expression of MUC2 and MUC5AC gene and protein expression in NCI-H292 cells. Our findings are in line with previous studies1923.
Here, we found that MF inhibited the stimulating effects of PMA on MUC2 and MUC5AC mRNA and protein expression including total mucin synthesis in NCI-H292 cells. To the best of our knowledge, there are no reports about the effect of MF on mucin gene and protein expression from airway epithelial cells. It was reported that mitogen-activated protein kinases and nuclear factor κB pathways play important roles in PMA-induced mucin activation1923. Although it is not known exactly how MF suppresses mucin synthesis in airway epithelial cells, it is probably that the suppression occurs somewhere in the pathways.
Previous reports demonstrated that following the dry-powder inhaler administration of MF (400 µg); the plasma concentrations of MF were below the limit of quantification (50 pg/mL) in most of subjects. The system bioavailability of this drug by this route was estimated to be less than 1%2425. However, MF is rapidly and extensively metabolized. Therefore, plasma concentration of MF may not correlate with its clinical effects because the large numbers of active metabolites were formed in body. In addition, in vitro binding studies for MF were reported to 98%–99% in the concentration ranges of 5–500 ng/mL2627. MF concentrations used in our study are comparable to the report by Papi et al.28 that rhinovirus-induced intercellular adhesion molecule-1 (ICAM-1) release from primary bronchial cells (A549) was suppressed with MF at concentrations of 0.43 pg/mL to 430.53 ng/mL. The inhibition of ICAM-1 was also observed in human lung fibroblasts treated with MF at the concentrations up to 10 nM (4.3 ng/mL)29. Thus, it is likely that concentrations of MF used in the present study are found in vivo in the lung following administration by inhalation.
The present study reveals that BUD inhibits PMA mediated MUC2 and MUC5AC genes in NCI-H292 cells. Inhibitory effects of BUD on the levels of MUC2 and MUC5AC proteins including total mucin produced by PMA-stimulated NCIH292 cells were also demonstrated in the study. Our findings were similar to the result that BUD suppressed MUC2 and MUC5AC up-regulation in NCI-H292 cells stimulated with interleukin (IL)-1β30. Although the BUD concentration used in our study is relatively high but this concentration is in the range of doses used to study in primary airway epithelial cells31 and NCI-H292 cells30. Our findings are also consistent with those of previous studies where demonstrated inhibitory effects of DEX on MUC5AC mRNA abundance in NCI-H292 and NHBE cells932. This confirmed that we could use DEX as a positive control.
MF has been shown to have a high potency in reducing mediators involved in the early and late phase of the inflammatory response. To explore the role of steroid drugs in TNF-α synthesis, we pretreated NCI-H292 cells with steroid drugs before PMA stimulation. We demonstrated that MF, BUD and DEX inhibited TNF-α production from PMA-stimulated NCIH292 cells. Our findings were in line with a previous study using LPS-stimulated murine myelomonocytic leukemia cells33. Our results are also in partial agreement with some previous studies of BUD and DEX. Inhibitory effects of BUD were observed in LPS-induced TNF-α in human lung epithelial cells and alveolar macrophages34. Previous studies demonstrated inhibitory effects of DEX on human monocytes35 and rat macrophages 36.
It was reported that MF was at least 10 times more potent than beclomethasone, DEX, and hydrocortisone in inhibiting the production and release of proinflammatory mediators including TNF-α, IL-1, IL-5, and IL-6 in murine cell culture33. However, the previous report is not confirmed by our study. Although we found that MF at lower concentrations inhibited TNF-α and mucin production from PMA-induced NCI-H292 but it could not compare to that of the single dose of DEX and BUD.
TNF-α has been reported to be a stimulant for secretion and gene expression of MUC237 and MUC5AC genes3839 in NCI-H292 cells. Although in this study we did not determine direct effects of MF, BUD or DEX on mucin production in NCI-H292 cells stimulated with TNF-α but parallel inhibition of mucin and TNF-α production was observed in treated cells. Therefore, in addition to direct inhibition of MUC2 and MUC5AC genes as shown in previous reports and our study, we suggests that the suppression of mucin may be also cause by indirectly inhibitory effect of MF, BUD, and DEX on TNF-α release from the air way epithelial cells.
It should be noted that the inhibitory effects of MF and BUD on mucin and TNF-α production in the present study was not due to cytotoxicity of the tested drugs. We found no cytotoxic effects of MF and BUD on NCI-H292 cells within the tested concentrations for 72 hours.
In summary, the present study demonstrated that the stimulation of NCI-H292 cells with PMA results in the up-regulation of MUC2 and MUC5AC gene and protein expression, total mucin production, and TNF-α release. Pretreatment of NCI-H292 cells with MF, BUD, or DEX attenuated the stimulating effects of PMA. Information gained from the present study is important in clarifying the benefit of MF in attenuating pulmonary inflammation regarding to mucin inhibition. However, at present no data are available regarding the magnitude of the reduction in mucin content that is clinically significant. For this reason, appropriate clinical studies are needed to evaluate effects of MF on mucin.
Figures and Tables
Figure 1
Effects of MF and BUD on cell viability. NCI-H292 cells were treated with MF (A) and BUD (B) at indicated concentrations for 72 hours. Cell viability was determined by the MTT assay and results are presented as percent of the control (untreated group). Values are expressed as mean±SEM of three independent experiments. MF: mometasone fuorate; BUD: budesonide; MTT: 3-(4,5-dimethylthiazol-2-yl)-2,5-diphenyltetrazolium bromide; SEM: standard error of the mean.
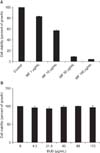
Figure 2
Glucocorticoids inhibit PMA-induced MUC2 gene expression. NCI-H292 cells were pretreated with or without MF, BUD, or DEX at indicated concentrations for 2 hours. Subsequently, the cells were stimulated with PMA for 8 hours. Total RNA was isolated and MUC2 mRNA levels were analyzed by real-time polymerase chain reaction. Values are expressed as mean±SEM of three independent experiments. *p<0.05, compared to PMA-stimulated cells in the absence of glucocorticoids. PMA: phorbol-12-myristate-13-acetate; MF: mometasone fuorate; BUD: budesonide; DEX: dexamethasone; SEM: standard error of the mean; GAPDH: glyceraldehyde 3-phosphate dehydrogenase.
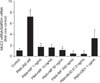
Figure 3
MF and BUD inhibit PMA-induced MUC5AC gene expression. NCI-H292 cell were pretreated with or without MF, BUD, or DEX at indicated concentrations for 2 hours before stimulation with PMA for 8 hours. Total RNA was isolated and MUC5AC mRNA levels were analyzed by real-time polymerase chain reaction. Values are expressed as mean±SEM of three independent experiments. *p<0.05, compared to PMA-stimulated cells in the absence of glucocorticoids. MF: mometasone fuorate; BUD: budesonide; PMA: phorbol-12-myristate-13-acetate; DEX: dexamethasone; SEM: standard error of the mean; GAPDH: glyceraldehyde 3-phosphate dehydrogenase.
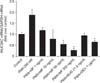
Figure 4
MF and BUD inhibit PMA-induced MUC2 synthesis. NCI-H292 cells were pretreated with indicated glucocorticoids for 2 hours. Subsequently, the cells were stimulated with PMA for 8 hours. The level of MUC2 protein in culture supernatants was determined using ELISA. Data shown are mean±SEM of three independent experiments. *p<0.05, compared to PMA-stimulated cells in the absence of glucocorticoids. MF: mometasone fuorate; BUD: budesonide; PMA: phorbol-12-myristate-13-acetate; ELISA: enzyme-linked immunosorbent assay; SEM: standard error of the mean; DEX: dexamethasone.
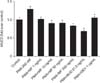
Figure 5
MF and BUD inhibit PMA-induced MUC5AC production. NCI-H292 cells were pretreated with indicated glucocorticoids for 2 hours. Subsequently, the cells were stimulated with PMA for 8 hours. The level of MUC5AC protein in culture supernatants was determined using ELISA. Data shown are mean±SEM of three independent experiments. *p<0.05, compared to PMA-stimulated cells in the absence of glucocorticoids. MF: mometasone fuorate; BUD: budesonide; PMA: phorbol-12-myristate-13-acetate; ELISA: enzyme-linked immunosorbent assay; SEM: standard error of the mean; DEX: dexamethasone.
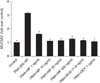
Figure 6
Inhibition of total mucin production by MF, BUD, and DEX. NCI-H292 cells were pretreated with the indicated glucocorticoids for 2 hours. The cells were stimulated with PMA and total mucin levels were quantitated by an enzyme-linked lectin assay. Data shown are mean±SEM of three independent experiments. *p<0.05, compared to PMA-stimulated cells in the absence of glucocorticoids. MF: mometasone fuorate; BUD: budesonide; DEX: dexamethasone; PMA: phorbol-12-myristate-13-acetate; SEM: standard error of the mean.
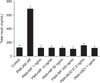
Figure 7
Glucocorticoids suppress the PMA-induced release of TNF-α from NCI-H292 cells. NCI-H292 cells were pretreated with MF, BUD, or DEX for 2 hours before the cells were stimulated with PMA for 8 hours. TNF-α in the culture supernatant was quantitated by ELISA. Data shown are mean±SEM of three independent experiments. *p<0.05, compared to PMA-stimulated cells in the absence of glucocorticoids. PMA: phorbol-12-myristate-13-acetate; TNF-α: tumor necrosis factor α; MF: mometasone fuorate; BUD: budesonide; DEX: dexamethasone; ELISA: enzyme-linked immunosorbent assay; SEM: standard error of the mean.
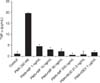
Table 1
Primer and probe sequences used in real-time RT-PCR
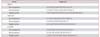
Acknowledgments
This study was supported by 2013 Research Grant from Thammasat University, Thailand.
References
1. Zhang X, Morrison-Carpenter T, Holt JB, Callahan DB. Trends in adult current asthma prevalence and contributing risk factors in the United States by state: 2000-2009. BMC Public Health. 2013; 13:1156.
2. Sedaghat AR, Phipatanakul W, Cunningham MJ. Prevalence of and associations with allergic rhinitis in children with chronic rhinosinusitis. Int J Pediatr Otorhinolaryngol. 2014; 78:343–347.
3. Mello JF Jr, Mion Ode G, Andrade NA, Anselmo-Lima WT, Stamm AE, Almeida WL, et al. Brazilian Academy of Rhinology position paper on topical intranasal therapy. Braz J Otorhinolaryngol. 2013; 79:391–400.
4. Yang D, Wang J, Bunjhoo H, Xiong W, Xu Y, Zhao J. Comparison of the efficacy and safety of mometasone furoate to other inhaled steroids for asthma: a meta-analysis. Asian Pac J Allergy Immunol. 2013; 31:26–35.
5. Martinez-Anton A, Debolos C, Garrido M, Roca-Ferrer J, Barranco C, Alobid I, et al. Mucin genes have different expression patterns in healthy and diseased upper airway mucosa. Clin Exp Allergy. 2006; 36:448–457.
6. Kim DH, Chu HS, Lee JY, Hwang SJ, Lee SH, Lee HM. Up-regulation of MUC5AC and MUC5B mucin genes in chronic rhinosinusitis. Arch Otolaryngol Head Neck Surg. 2004; 130:747–752.
7. Morcillo EJ, Cortijo J. Mucus and MUC in asthma. Curr Opin Pulm Med. 2006; 12:1–6.
8. Kim YD, Kwon EJ, Park DW, Song SY, Yoon SK, Baek SH. Interleukin-1beta induces MUC2 and MUC5AC synthesis through cyclooxygenase-2 in NCI-H292 cells. Mol Pharmacol. 2002; 62:1112–1118.
9. Takami S, Mizuno T, Oyanagi T, Tadaki H, Suzuki T, Muramatsu K, et al. Glucocorticoids inhibit MUC5AC production induced by transforming growth factor-alpha in human respiratory cells. Allergol Int. 2012; 61:451–459.
10. Burgel PR, Cardell LO, Ueki IF, Nadel JA. Intranasal steroids decrease eosinophils but not mucin expression in nasal polyps. Eur Respir J. 2004; 24:594–600.
11. Westergaard CG, Porsbjerg C, Backer V. A review of mometasone furoate/formoterol in the treatment of asthma. Expert Opin Pharmacother. 2013; 14:339–346.
12. Park SJ, Kim TH, Jun YJ, Lee SH, Ryu HY, Jung KJ, et al. Chronic rhinosinusitis with polyps and without polyps is associated with increased expression of suppressors of cytokine signaling 1 and 3. J Allergy Clin Immunol. 2013; 131:772–780.
13. Brightling C, Berry M, Amrani Y. Targeting TNF-alpha: a novel therapeutic approach for asthma. J Allergy Clin Immunol. 2008; 121:5–10.
14. Rudiger JJ, Gencay M, Yang JQ, Bihl M, Tamm M, Roth M. Fast beneficial systemic anti-inflammatory effects of inhaled budesonide and formoterol on circulating lymphocytes in asthma. Respirology. 2013; 18:840–847.
15. Ciprandi G, Tosca MA, Passalacqua G, Canonica GW. Intranasal mometasone furoate reduces late-phase inflammation after allergen challenge. Ann Allergy Asthma Immunol. 2001; 86:433–438.
16. Inoue D, Yamaya M, Kubo H, Sasaki T, Hosoda M, Numasaki M, et al. Mechanisms of mucin production by rhinovirus infection in cultured human airway epithelial cells. Respir Physiol Neurobiol. 2006; 154:484–499.
17. Li R, Meng Z. Effects of SO2 derivatives on expressions of MUC5AC and IL-13 in human bronchial epithelial cells. Arch Toxicol. 2007; 81:867–874.
18. Livak KJ, Schmittgen TD. Analysis of relative gene expression data using real-time quantitative PCR and the 2(-Delta Delta C(T)) Method. Methods. 2001; 25:402–408.
19. Bamrungphon W, Prempracha N, Bunchu N, Rangdaeng S, Sandhu T, Srisukho S, et al. A new mucin antibody/enzyme-linked lectin-sandwich assay of serum MUC5AC mucin for the diagnosis of cholangiocarcinoma. Cancer Lett. 2007; 247:301–308.
20. Hewson CA, Edbrooke MR, Johnston SL. PMA induces the MUC5AC respiratory mucin in human bronchial epithelial cells, via PKC, EGF/TGF-alpha, Ras/Raf, MEK, ERK and Sp1-dependent mechanisms. J Mol Biol. 2004; 344:683–695.
21. Davies DE, Polosa R, Puddicombe SM, Richter A, Holgate ST. The epidermal growth factor receptor and its ligand family: their potential role in repair and remodelling in asthma. Allergy. 1999; 54:771–783.
22. Shao MX, Nadel JA. Dual oxidase 1-dependent MUC5AC mucin expression in cultured human airway epithelial cells. Proc Natl Acad Sci U S A. 2005; 102:767–772.
23. Ishinaga H, Takeuchi K, Kishioka C, Suzuki S, Basbaum C, Majima Y. Pranlukast inhibits NF-kappaB activation and MUC2 gene expression in cultured human epithelial cells. Pharmacology. 2005; 73:89–96.
24. Bernstein DI, Berkowitz RB, Chervinsky P, Dvorin DJ, Finn AF, Gross GN, et al. Dose-ranging study of a new steroid for asthma: mometasone furoate dry powder inhaler. Respir Med. 1999; 93:603–612.
25. Affrime MB, Cuss F, Padhi D, Wirth M, Pai S, Clement RP, et al. Bioavailability and metabolism of mometasone furoate following administration by metered-dose and dry-powder inhalers in healthy human volunteers. J Clin Pharmacol. 2000; 40:1227–1236.
26. Smith CL, Kreutner W. In vitro glucocorticoid receptor binding and transcriptional activation by topically active glucocorticoids. Arzneimittelforschung. 1998; 48:956–960.
27. Valotis A, Hogger P. Significant receptor affinities of metabolites and a degradation product of mometasone furoate. Respir Res. 2004; 5:7.
28. Papi A, Papadopoulos NG, Degitz K, Holgate ST, Johnston SL. Corticosteroids inhibit rhinovirus-induced intercellular adhesion molecule-1 up-regulation and promoter activation on respiratory epithelial cells. J Allergy Clin Immunol. 2000; 105(2 Pt 1):318–326.
29. Sabatini F, Silvestri M, Sale R, Serpero L, Giuliani M, Scarso L, et al. Concentration-dependent effects of mometasone furoate and dexamethasone on foetal lung fibroblast functions involved in airway inflammation and remodeling. Pulm Pharmacol Ther. 2003; 16:287–297.
30. Kim YD, Kwon EJ, Kwon TK, Baek SH, Song SY, Suh JS. Regulation of IL-1beta-mediated MUC2 gene in NCI-H292 human airway epithelial cells. Biochem Biophys Res Commun. 2000; 274:112–116.
31. Feinstein MB, Schleimer RP. Regulation of the action of hydrocortisone in airway epithelial cells by 11beta-hydroxysteroid dehydrogenase. Am J Respir Cell Mol Biol. 1999; 21:403–408.
32. Chen Y, Nickola TJ, DiFronzo NL, Colberg-Poley AM, Rose MC. Dexamethasone-mediated repression of MUC5AC gene expression in human lung epithelial cells. Am J Respir Cell Mol Biol. 2006; 34:338–347.
33. Barton BE, Jakway JP, Smith SR, Siegel MI. Cytokine inhibition by a novel steroid, mometasone furoate. Immunopharmacol Immunotoxicol. 1991; 13:251–261.
34. Ek A, Larsson K, Siljerud S, Palmberg L. Fluticasone and budesonide inhibit cytokine release in human lung epithelial cells and alveolar macrophages. Allergy. 1999; 54:691–699.
35. Joyce DA, Kloda A, Steer JH. Dexamethasone suppresses release of soluble TNF receptors by human monocytes concurrently with TNF-alpha suppression. Immunol Cell Biol. 1997; 75:345–350.
36. Nakamura Y, Murai T, Ogawa Y. Effect of in vitro and in vivo administration of dexamethasone on rat macrophage functions: comparison between alveolar and peritoneal macrophages. Eur Respir J. 1996; 9:301–306.
37. Levine SJ, Larivee P, Logun C, Angus CW, Ognibene FP, Shelhamer JH. Tumor necrosis factor-alpha induces mucin hypersecretion and MUC-2 gene expression by human airway epithelial cells. Am J Respir Cell Mol Biol. 1995; 12:196–204.
38. Ryu J, Lee HJ, Park SH, Sikder MA, Kim JO, Hong JH, et al. Effect of Prunetin on TNF-alpha-induced MUC5AC mucin gene expression, production, degradation of IkappaB and translocation of NF-kappaB p65 in human airway epithelial cells. Tuberc Respir Dis. 2013; 75:205–209.
39. Shimizu T, Shimizu S, Hattori R, Gabazza EC, Majima Y. In vivo and in vitro effects of macrolide antibiotics on mucus secretion in airway epithelial cells. Am J Respir Crit Care Med. 2003; 168:581–587.