Abstract
Background
Recent evidences have revealed metabolic functions of p53 in cancer cells; adaptation or survival to metabolic stress and metabolic shift toward oxidative phosphorylation. However, further studies in clinical setting are needed. We investigated whether p53 protein expression, as a surrogate marker for loss of p53 function, is associated with metabolic features of stage I non-small cell lung cancer (NSCLC), focusing on tumor necrosis and maximal standardized uptake value (SUVmax) on 18F-fluorodeoxyglucose positron emission tomography.
Methods
Clinical information was obtained from retrospective review of medical records. p53 expression was assessed by immunohistochemical staining.
Results
p53 protein expression was detected in 112 (46%) of 241 NSCLC cases included in this study. p53 expression was independently associated with the presence of necrosis (odds ratio [OR], 2.316; 95% confidence interval [CI], 1.215~4.416; p=0.011). Non-adenocarcinoma histology (OR, 8.049; 95% CI, 4.072~15.911; p<0.001) and poorly differentiation (OR, 6.474; 95% CI, 2.998~13.979; p<0.001) were also independently associated with the presence of necrosis. However, p53 expression was not a significant factor for SUVmax.
The tumor suppressor p53 plays key roles in coordinating cellular responses to diverse stresses through transcriptional regulation of target gene expression1. Apoptosis, cell cycle arrest, DNA repair, and senescence are well-known cellular responses induced by p53 activation2. Recently, several lines of evidence have suggested that p53 acts as bioenergetic switch for cellular energy homeostasis. Activation of p53 up-regulates expression of TP53-induced glycolysis and apoptosis regulator, which lowers the fructose-2,6-bisphosphate level3. In addition, p53 suppresses expression of phosphoglycerate mutase and induces synthesis of cytochrome c oxidase subunit of complex IV of the electron transport chain in mitochondria4,5. Pharmacologic activation of AMP-activated protein kinase (AMPK) by metformin or aminoimidazole carboxamide ribonucleotide enhances oxidation of fatty acids, an alternative energy substrate for cancer cells, in a p53-dependent manner6. These p53-induced metabolic modulations may lead to a shift in glucose catabolism toward oxidative phosphorylation rather than glycolysis.
In contrast to its role in apoptosis induction p53 can exert a pro-survival effect, especially under conditions of metabolic stress. Two independent studies demonstrated that AMPK-dependent p53 activity is critical for the maintenance of cellular viability under glucose-deprived conditions7,8. In addition, metformin selectively induced apoptotic cell death in metabolically-compromised areas of p53-deficient tumors6.
p53 mutations that cause perturbation of p53 function are the most common mutations in human cancers, including non-small cell lung cancer (NSCLC)1,2,9,10. Based on the metabolic roles of p53, tumors with loss of p53 function are predicted to exhibit a glycolytic phenotype and have increased susceptibility to stress-induced cell death. These metabolic features of tumors could be reflected by increased maximal standardized uptake value (SUVmax) on 18F-fluorodeoxyglucose positron emission tomography (18F-FDG PET)/CT and by observation of cellular death in pathologic specimens11. p53 gene mutations cause accumulation of p53 protein in cancer cells, which is rarely seen for while wild-type p5312. Therefore, we investigated whether p53 protein expression, as a surrogate marker for loss of p53 function, is associated with metabolic features of stage I NSCLC, focusing on tumor necrosis and SUVmax on 18F-FDG PET/CT.
We retrospectively reviewed medical records of patients who received surgical resection of NSCLC from March 2003 and August 2006 and obtained data on patient demographics, smoking status, preoperative staging work-up, and type of surgery. Tumor size, histological type, grade of differentiation, and visceral pleural invasion were determined from post-resection pathology reports. Pathologic stage was determined on the basis of all available data. Patients meeting the following criteria were included in this study: pathologic stage I NSCLC (excluding carcinoid, mucoepidermoid carcinoma, or adenoid cystic carcinoma) treated by lung resection surgery and mediastinal lymph node dissection or sampling; immunohistochemical staining of primary tumor for p53 expression; 18F-FDG PET/CT imaging performed within 30 days of lung cancer surgery at the Samsung Medical Center only; tumor diameter more than 1 cm due to spatial resolution of the PET scanner13; and no neoadjuvant therapy. Patients with no description of necrosis in the pathologic report were excluded. This study was approved by the Institutional Review Board of the Samsung Medical Center, which waived the requirement for informed consent of individual patients, given the retrospective nature of the study.
Immunohistochemical staining was performed using the avidin-biotinylated horseradish peroxidase complex method (DAKO LSAB kit). Briefly, 4-µm thick formalin-fixed paraffin-embedded lung tissue sections were prepared for immunohistochemistry. The tissue sections were deparaffinized in xylene and rehydrated through a series of alcohols. The sections were treated in 10 mmol/L citrate buffer (pH 6.0) for 10 minutes at 120℃ in an autoclave for antigen retrieval, then incubated overnight at 4℃ with mouse anti-p53 monoclonal antibody (1:20 dilution; BP 53.12; Invitrogen & Zymed Technologies, South San Francisco, CA, USA) followed by incubation with biotinylated anti-mouse IgG for 30 minutes. The tissue sections were treated with streptavidin-biotin complex peroxidase, followed by 3.3'diaminobenzidine tetrahydrochloride as the chromogen, and counterstained with hematoxylin. Nuclear staining of normal stromal cells was used as an internal control. The score of p53 immunoreactivity was expressed as the percentage of the total number of tumor cell nuclei showing p53 staining. Tumor p53-positivity was defined as a score ≥10%14.
All patients fasted for at least six hours before the positron emission tomography (PET)/computed tomography (CT) examination, although oral hydration with glucose-free water was allowed. After normal peripheral blood glucose level (≤150 mg/dL) was confirmed, patients received an intravenous injection of 370 MBq (10 mCi) 18F-FDG and rested for approximately 45 minutes before undergoing scanning. A PET/CT device (Discovery LS; GE Healthcare, Milwaukee, WI, USA) consisting of a PET scanner (Advance NXi; GE Medical Systems, Waukesha, WI, USA) and an 8-slice CT scanner (LightSpeed Plus; GE Medical Systems) was used for image acquisition. The 18F-FDG PET data were evaluated using SUVmax because this is a semiquantitative and widely accepted index that characterizes the glycolytic activity of the tumor11,15. We used the region of interest analysis tools included with the scanner to calculate the SUVmax over the primary tumor after correction for the injected dose of 18F-FDG and the patient's body weight.
We used the Students t-test for analysis of variables with normal distribution and the Mann-Whitney U test for variables without normal distribution. Categorical variables were analyzed by Pearson's chi-square test or Fisher's exact test. The linear-by-linear association method was used to test for trends across an ordinal categorical variable. For multivariate logistic regression analysis, independent variables were selected on the basis of clinical and biological relevance with necrosis and tested for associations between necrosis and independent variables using the enter method. Multiple linear regression with enter method was used for the analysis of associations between SUVmax and independent variables. Analyses were performed using an SPSS package, network version 17.0 (SPSS Inc., Chicago, IL, USA). All p-values reported are two-sided, and a p-value <0.05 was considered significant.
Between March 2003 and August 2006, 1,063 patients with NSCLC underwent surgical resection at the Samsung Medical Center. Of these, 508 patients had pathologic stage I NSCLC, and 241 met the eligibility criteria for this study. Baseline characteristics of the patients are summarized in Table 1. Most of tumors were adenocarcinoma or squamous cell carcinoma (223/241, 93%). p53 protein expression was detected in 112 (46%) tumors. Necrosis was observed in 104 (43%) tumors. The median SUVmax of primary tumors of NSCLC was 10.8. p53 expression was significantly associated with male gender, smoking, stage IB, presence of necrosis, and non-adenocarcinoma histology (Table 2). p53 expression increased significantly as the histological grade of differentiation progressed (p=0.008). SUVmax of p53-positive tumors was significantly higher than that of p53-negative tumors (12.6 vs. 8.1; p<0.001).
The presence of necrosis was significantly associated with male gender, smoking, stage IB, non-adencarcinoma histology, progression of histological grade of differentiation, and p53 expression (Table 3). Multivariate logistic regression analysis adjusted for stage, histology (adenocarcinoma vs non-adenocarcinoma), grade of differentiation (well and moderately vs. poorly), and p53 expression revealed that p53 expression was independently associated with the presence of necrosis (odds ratio [OR], 2.316; 95% confidence interval [CI], 1.215~4.416; p=0.011) (Table 4, Figure 1). Non-adenocarcinoma histology (OR, 8.049; 95% CI, 4.072~15.911; p<0.001) and poorly differentiation (OR, 6.474; 95% CI, 2.998~13.979; p<0.001) were also independently associated with the presence of necrosis (Table 4).
Significantly higher SUVmaxs were observed in male gender (12.1 vs. 5.9; p<0.001), smoker (12.5 vs. 5.9; p<0.001), stage IB (12.5 vs. 6.8; p<0.001), non-adenocarinoma (14.5 vs. 6.0; p<0.001), and poorly differentiation (13.7 vs. 8.8; p<0.001) compared the counterparts. After adjustment for stage, histology, grade of differentiation, and p53 expression for multiple linear regression analysis, stage IB (coefficient, 2.805; standard error [SE], 0.798; p=0.001), poorly differentiation (coefficient, 2.006; SE, 0.835; p=0.017), non-adenocarcinoma (coefficient,7.219; SE, 0.776; p<0.001) were found to be independent variables for SUVmax. However, p53 expression was not a significant variable for SUVmax (p=0.694).
Metabolic adaptations are critically important for cancer cells to sustain viability under conditions of bioenergetic stress. Recent studies demonstrated that p53 activity is critical for cancer cells to overcome metabolic stress7,8. In contrast to genotoxic stress, which induces cellular apoptosis via p53 activation, metabolic stress activates a pro-survival function of p537,8. In the present study, we demonstrated that p53 expression was a significant and independent factor associated with tumor necrosis in patient with stage I NSCLC. Of note, all cases in this study had no history of chemotherapy or radiotherapy before surgical resection; therefore the presence of necrosis reflects naturally occurring cell death caused by failure to overcome various metabolic stresses during the early course of NSCLC progression. The association between necrosis and p53 expression thus provides additional indirect support for a pro-survival function of the tumor suppressor p53 in cancer.
Enhanced glucose uptake is a fundamental characteristic of cancer cell metabolism that could be assessed by measuring SUVmax of primary tumor via 18F-FDG PET11,15. Glucose transporter, hexokinase, phosphofructokinase, hypoxia-inducible factor-1alpha (HIF-1α), c-Myc, ras, Akt, and p53 are important molecules that contribute to glycolytic activity of cancer cells11. In clinical settings, several studies have reported that expression of glucose transporter 1, HIF-1α, p53, and Ki-67 are significantly correlated with high SUVmax in lung cancer16-19. In agreement with previous reports16,20, this study found a significantly higher SUVmax in p53-positive NSCLC than in p53-negative NSCLC upon univariate analysis. However, p53 expression was not an independent factor for SUVmax in this study. Actually, non-adenocarcinoma histology, poorly differentiation, and stage IB were found to be independent factors for SUVmax. SUVmax on 18F-FDG PET is a semiquantitative index which might be affected by fasting duration, plasma glucose level, region of interest parameters, and cellular density of tumors21. To investigate the association between molecular markers and SUVmax, subgrouping according to histology, grade of differentiation, and stage might be necessary in the future study.
p53 expression assessed by immunohistochemistry were used as a surrogate marker of p53 mutations in this study. Indeed, p53 protein expression typically represents missense mutations and not all mutations of the p53 gene22. However, most of the mutations identified in lung cancers are missense mutations22, and several studies have demonstrated clinical implications of p53 status assessed by immunohistochemistry as a surrogate marker of p53 mutations in lung cancer23-25. Furthermore, immunohistochemistry is a simple method to perform using clinical samples. Because tumor p53-positivity was defined as a score ≥10% in a previous research from our institution using NSCLC tissues14, we applied the same criteria in this study. However, further study to validate the criteria is needed. To reduce bias in the interpretation of p53 expression, immunohistochemical evaluation incorporated both stain intensity and percentage such as HSCORE may be helpful in the future study26.
In summary, p53 protein expression is independently associated with the presence of necrosis, but not SUVmax in patients with stage I NSCLC.
Figures and Tables
Figure 1
Surgical specimens of non-small cell lung cancer without necrosis (A) or with necrosis (B, arrow) (H&E stain, ×100). Immunostainings for p53 (C~F). No p53 expression was observed in the nuclei of non-small cell lung cancer without necrosis (C, p53 immunostaining, ×100; E, p53 immunostaining, ×400). p53 is strongly expressed in the nuclei of non-small cell lung cancer with necrosis (D, p53 immunostaining, ×100; F, p53 immunostaining, ×400).
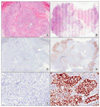
Table 2
Relationship between p53 expression and clinical characteristics in patients with stage I non-small cell lung cancer
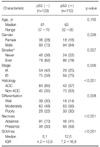
References
1. Levine AJ. p53, the cellular gatekeeper for growth and division. Cell. 1997. 88:323–331.
2. Vogelstein B, Lane D, Levine AJ. Surfing the p53 network. Nature. 2000. 408:307–310.
3. Bensaad K, Tsuruta A, Selak MA, Vidal MN, Nakano K, Bartrons R, et al. TIGAR, a p53-inducible regulator of glycolysis and apoptosis. Cell. 2006. 126:107–120.
4. Kondoh H, Lleonart ME, Gil J, Wang J, Degan P, Peters G, et al. Glycolytic enzymes can modulate cellular life span. Cancer Res. 2005. 65:177–185.
5. Matoba S, Kang JG, Patino WD, Wragg A, Boehm M, Gavrilova O, et al. p53 regulates mitochondrial respiration. Science. 2006. 312:1650–1653.
6. Buzzai M, Jones RG, Amaravadi RK, Lum JJ, DeBerardinis RJ, Zhao F, et al. Systemic treatment with the antidiabetic drug metformin selectively impairs p53-deficient tumor cell growth. Cancer Res. 2007. 67:6745–6752.
7. Jones RG, Plas DR, Kubek S, Buzzai M, Mu J, Xu Y, et al. AMP-activated protein kinase induces a p53-dependent metabolic checkpoint. Mol Cell. 2005. 18:283–293.
8. Okoshi R, Ozaki T, Yamamoto H, Ando K, Koida N, Ono S, et al. Activation of AMP-activated protein kinase induces p53-dependent apoptotic cell death in response to energetic stress. J Biol Chem. 2008. 283:3979–3987.
9. Takahashi T, Nau MM, Chiba I, Birrer MJ, Rosenberg RK, Vinocour M, et al. p53: a frequent target for genetic abnormalities in lung cancer. Science. 1989. 246:491–494.
10. Ahrendt SA, Chow JT, Yang SC, Wu L, Zhang MJ, Jen J, et al. Alcohol consumption and cigarette smoking increase the frequency of p53 mutations in non-small cell lung cancer. Cancer Res. 2000. 60:3155–3159.
11. Buerkle A, Weber WA. Imaging of tumor glucose utilization with positron emission tomography. Cancer Metastasis Rev. 2008. 27:545–554.
12. Hall PA, Lane DP. p53 in tumour pathology: can we trust immunohistochemistry?--Revisited! J Pathol. 1994. 172:1–4.
13. Nomori H, Watanabe K, Ohtsuka T, Naruke T, Suemasu K, Uno K. Evaluation of F-18 fluorodeoxyglucose (FDG) PET scanning for pulmonary nodules less than 3 cm in diameter, with special reference to the CT images. Lung Cancer. 2004. 45:19–27.
14. Kwon YM, Park JH, Kim H, Shim YM, Kim J, Han J, et al. Different susceptibility of increased DNMT1 expression by exposure to tobacco smoke according to histology in primary non-small cell lung cancer. J Cancer Res Clin Oncol. 2007. 133:219–226.
15. Berghmans T, Dusart M, Paesmans M, Hossein-Foucher C, Buvat I, Castaigne C, et al. Primary tumor standardized uptake value measured on fluorodeoxyglucose positron emission tomography is of prognostic value for survival in non-small cell lung cancer: update of a systematic review and meta-analysis by the European Lung Cancer Working Party for the International Association for the Study of Lung Cancer Staging Project. J Thorac Oncol. 2008. 3:6–12.
16. Taylor MD, Smith PW, Brix WK, Wick MR, Theodosakis N, Swenson BR, et al. Fluorodeoxyglucose positron emission tomography and tumor marker expression in non-small cell lung cancer. J Thorac Cardiovasc Surg. 2009. 137:43–48.
17. Yamamoto Y, Nishiyama Y, Ishikawa S, Nakano J, Chang SS, Bandoh S, et al. Correlation of 18F-FLT and 18F-FDG uptake on PET with Ki-67 immunohistochemistry in non-small cell lung cancer. Eur J Nucl Med Mol Imaging. 2007. 34:1610–1616.
18. van Baardwijk A, Dooms C, van Suylen RJ, Verbeken E, Hochstenbag M, Dehing-Oberije C, et al. The maximum uptake of (18)F-deoxyglucose on positron emission tomography scan correlates with survival, hypoxia inducible factor-1alpha and GLUT-1 in non-small cell lung cancer. Eur J Cancer. 2007. 43:1392–1398.
19. Chung JK, Lee YJ, Kim SK, Jeong JM, Lee DS, Lee MC. Comparison of [18F]fluorodeoxyglucose uptake with glucose transporter-1 expression and proliferation rate in human glioma and non-small-cell lung cancer. Nucl Med Commun. 2004. 25:11–17.
20. Zhang ZJ, Chen JH, Meng L, Du JJ, Zhang L, Liu Y, et al. 18F-FDG uptake as a biologic factor predicting outcome in patients with resected non-small-cell lung cancer. Chin Med J (Engl). 2007. 120:125–131.
21. Bacharach SL, Sundaram SK. 18F-FDG in cardiology and oncology: the bitter with the sweet. J Nucl Med. 2002. 43:1542–1544.
22. Toyooka S, Tsuda T, Gazdar AF. The TP53 gene, tobacco exposure, and lung cancer. Hum Mutat. 2003. 21:229–239.
23. D'Amico TA, Massey M, Herndon JE 2nd, Moore MB, Harpole DH Jr. A biologic risk model for stage I lung cancer: immunohistochemical analysis of 408 patients with the use of ten molecular markers. J Thorac Cardiovasc Surg. 1999. 117:736–743.
24. Mitsudomi T, Hamajima N, Ogawa M, Takahashi T. Prognostic significance of p53 alterations in patients with non-small cell lung cancer: a meta-analysis. Clin Cancer Res. 2000. 6:4055–4063.
25. Steels E, Paesmans M, Berghmans T, Branle F, Lemaitre F, Mascaux C, et al. Role of p53 as a prognostic factor for survival in lung cancer: a systematic review of the literature with a meta-analysis. Eur Respir J. 2001. 18:705–719.
26. McCarty KS Jr, Szabo E, Flowers JL, Cox EB, Leight GS, Miller L, et al. Use of a monoclonal anti-estrogen receptor antibody in the immunohistochemical evaluation of human tumors. Cancer Res. 1986. 46:8 Suppl. 4244s–4248s.