Abstract
Background
The underlying pathogenesis of fat embolism-induced acute lung injury (ALI) has not been elucidated. In the present study, the pathogenesis of fat embolism-induced ALI was probed in association with neutrophilic oxidative stress in oleic acid (OA)-induced ALI of S-D rats.
Methods
OA was injected intravenously to provoke ALI in experimental rats. Five hours later, indices of ALI were measured to confirm the role of the neutrophilic respiratory burst. The effect of an inhibition of phospholipase A2 (PLA2) was also evaluated.
Acute respiratory distress syndrome (ARDS) is characterized by an acute inflammatory pulmonary edema resulting in severe hypoxemia and respiratory failure1. One of the etiologies of this morbid malady is the pulmonary fat embolism encountered in multiple traumas including multiple fracture2. Multiple fractures can be corrected by suitable surgical intervention but the leakage of minute fat droplets into microvasculatures is unavoidable during operation or injury. Even during major operations including abdominal surgery, minute fat droplets are leaked into the vasculatures and cause fat embolism.
Among many types of lipids, oleic acid (OA) is well known to provoke acute lung injury during pulmonary fat embolism3. Up to now, however, the underlying pathogenesis of the fat embolism-induced acute lung injury (ALI) has not been well known. Some insist that neutrophils play a key role in the genesis of acute alveolar flooding by the release of elastase and free radicals during pulmonary fat embolism4. Whereas Hill et al5 suggested that neutrophils were not involved in the ALI caused by infusion of OA in rats.
In addition, although free radicals are one of the candidates altering the function of alveolar capillary barrier in OA-induced (ALI), the main source of free radicals has not been elucidated. Since fat embolisminduced ALI or ARDS are currently serious clinical problem, especially closely related to sepsis or severe form of infection, some authors reported that the phospholipase A2 (PLA2), a rate limiting enzyme of inflammation, regulated the inflammatory reaction in the lung during ARDS6. The activation of PLA2 prior to that of NADPH oxidase is indispensable for the initiation of neutrophilic respiratory burst7. Many evidences have been issued that the activation of the PLA2 evokes neutrophilic respiratory burst in several organs8. Several proinflammatory lipid molecules released by the PLA2 activation are thought to be responsible for the respiratory burst9. Needless to say, neutrophils constitute a very important mobile defensive system of the body by releasing protease and free radicals to the vicinity of the inflamed area. But the altering or imbalance of the production of the free radicals and deterring of the antioxidant system by the neutrophils in the body ensue hazardous tissue injury10.
On the bases of these reports and hypotheses, we attempted to elucidate whether the neutrophilic respiratory burst is involved in the pathogenesis of OA-induced ALI. By carrying out these experiments we would like to confirm the role of neutrophils in OA-induced ALI, from which could be followed by the development of new modalities for the treatment or prevention of multiple trauma or fracture-induced ARDS.
Mepacrine was used for the inhibition of PLA2 of which activation is crucial for the neutrophilic respiratory burst.
Neutrophils were isolated from fresh human blood. Male Sprague-Dawley rats (BW 250 g) were pathogen free and purchased from Korean Scientific Animal Supplier. All chemicals were purchased from Sigma-Aldrich Chemical Co, if not commented otherwise.
To know the effect of OA on the generation of free radicals, neutrophils were isolated according to the methods of Haslett et al11. Briefly, 30 mL of blood containing heparin (100 IU) mixed with equal volume of Pentaspan in 50 mL syringe and stand for 45 minutes in two syringes.
After separation of plasma which contained neutrophils, it was overlayed on the gradient-made percoll solution (74, 55%) and centrifuged 1,500 rpm for 20 minutes at room temperature. Layer of neutrophils was collected and washed twice and resuspended with Hank's balance salt solution (HBSS with 1 mM CaCl2, pH 7.4).
According to the method of Botha12, the generation of superoxide anion was assessed by cytochrome-c reduction assay. In tubes containing neutrophils (1×107 cell/mL), HBSS, sodium oleate (100 µM), mepacrine were added and incubated for 30 minutes at 37℃ in water bath. The amount of produced superoxide anion was evaluated as the amount of reduced cytochrome-c (nmol/1×107 cells).
Nitro blue tetrazolium is reduced by superoxide anion and to form formazan granules. If neutrophils are in the state of respiratory burst, neutrophils react with NBT and engulf formazan granules which can be shown in the cytoplasm. For NBT test, isolated human neutrophils (2×107 cells/mL) were reacted in tubes containing 0.02% NBT, 100 µM sodium oleate and 100 µM mepacrine at 37℃ for 20 minutes. After incubation, the suspension of cells was centrifuged at 800 rpm for 10 minutes, the pellet was put on the slides, then the slides were wright stained. NBT positive neutrophils were counted per 200 neutrophils and the percentage of NBT positive cells was calculated.
To confirm the chemotactic effect of OA to neutrophils, neutrophil aggregation test was performed. In tube containing isolated neutrophils (1×107 cell/mL), NBT, sodium oleate and mepacrine (0.02%, 100 µM, 100 µM, respectively) were added. Then tubes were incubated at 37℃ for 20 minutes and the formation of clump consisted of neutrophils, OA and formazan was identified. Mepacrine was tried to know the effect of the inhibition of PLA2 which is known to be essential for the neutrophilic respiratory burst.
For the induction of ALI simulating OA-induced ARDS, OA (30 µg) was mixed with 0.1% bovine serum albumin (270 µL) and was injected into the saphenous vein of the rats13. Twenty four hours after the injection, rats were sacrificed by overdose injection of the mixture of ketamine hydrochloride and xylazine. The rats were thoracotomized and lungs were resected. Then the lungs were weighed, dipped into the liquid nitrogen for being frozen immediately and stored at -70℃ till analyzed. To know the effect of the inhibition of PLA2, mepacrine (20 mg/kg) was given intraperitoneally 30 minutes after OA injection.
At the time of sacrifice, body weights and resected lung weights were measured before the calculation of the lung weight/body weight ratio (L/B ratio). The ratio denotes the accumulation of lung water after treatment of OA and mepacrine.
The lung MPO activity (U/g of wet lung) was measured according to the Goldblum's method14. Briefly, left lungs were thawed and homogenized in 4.0 mL of phosphate buffer (20 mM, pH 7.4) before centrifugation at 30,000×g for 30 minutes at 4℃. Then the pellet was resuspended in 4.0 mL of phosphate buffer (50 mM, pH 6.0) with 0.5% hexadecyl tetramethyl ammonium bromide. Samples were sonicated (Polytron, Swizland) for 90 seconds on ice at maximal power and then incubated for 120 minutes at 60℃. MPO activity was measured using o-dianisidine as the chromogenic substrate and hydrogen peroxide to initiate the reaction. Absorbance at 460 nm was proportional to MPO activity.
To obtain BALF, bronchoalveolar lavage was carried out with Trisbuffered saline (pH 7.4). With 8.0 mL of buffer solution, 3 times of pushing and withdrawing through the trachea was done and collected 6.0 mL of BALF. The BALF was centrifuged 1,500 rpm for 20 minutes at room temperature to sediment cellular components. The supernatants were used for the determination of protein according to the method of Brown et al15.
To assess the migration of white blood cells into the alveolar lumen, we counted the number of WBC in the BALF. After BAL, BALFs were centrifuged and supernatants were collected for the measurement of protein. Then the sediments were resuspended with 0.1 mL of Tris-buffered saline and aliquots were used to count WBC with hemocytometer.
Following experimental results were obtained by the study to elucidate the role of neutrophilic oxidative stress in OA-induced ALI.
In cytochrome-c reduction assay, OA stimulated isolated human neutrophils to increase the generation of superoxide anion which resulted in reduction of 103.4±6.42 nmol of cytochrome-c/1.0×107 PMNs (n=9). Comparing with 46.1±1.51 nmol of cytochromec/1.0×107 PMNs (n=9) in control neutrophils, OA increased the production of free radicals from neutrophils significantly (p<0.001). And mepacrine (100 µM) suppressed the OA-provoked superoxide generation effectively (p<0.001) which resulted in the reduction of 38.9±0.3 nmol of cytochrome-c/1×107 PMNs (n=9) (Table 1).
In NBT test for the confirmation of neutrophilic respiratory burst, OA intensified neutrophilic respiratory burst which resulted in the increase of formazan positive cells to 96.1±2.42% (n=10) compared with 4.6±2.9% (n=10) of non-stimulated neutrophils. Mepacrine (100 µM) suppressed the neutrophilic respiratory burst which was resulted in the decrease of NBT positive cells to 9.1±5.72% (n=10, p<0.001) compared with 96.1±2.42% (n=10) of OA-stimulated neutrophils (Table 2).
Neutrophilic aggregation test for the confirmation of the neutrophilic chemotaxis of OA showed dramatic aggregation and chemotaxis of neutrophils (Figures 1, 2). The light microscopic examination revealed that the formed clumps were composed of neutrophils containing formazan granules and lipid droplets. Without OA, neutrophils did not form the aggregate and mepacrine (100 µM) inhibited the formation of clumps or aggregate completely. That is, the formation of the aggregate in OA stimulated neutrophils was 100% (n=10). However, in control and mepacrine treated neutrophils, the formation of aggregates was nil (n=10, respectively) (Table 3).
As one of the indices of the accumulation of the fluid in the lung, L/B ratio (lung weight×103/body weight) was calculated. Comparing with 5.45±0.45 of control rats (n=11), the L/B ratios of OA given rats were 8.07±0.54 (n=6) and higher (p<0.001) than that of control rats. Mepacrine (100 µM) decreased (p<0.001) L/B ratios to 5.36±0.60 (n=15) in OA-given rats (Table 4).
The protein content (O.D at 562 nm) in the BALF of OA-given rats were 0.45±0.15 (n=12) and was higher (p<0.001) than 0.27±0.05 of control rats (n=17). In comparison, mepacrine (100 µM) decreased (p<0.001) protein content to 0.33±0.09 (n=10) in OA given rats (Table 5).
The number of WBC (millions/two lungs) in BALF after OA treatment increased (p<0.001) to 20.15±0.39 (n=11) compared with 5.82±1.50 (n=10) of contol rats. Mepacrine (100 µM) decreased the number of WBC effectively to 6.76±2.47 (n=16, p<0.001) in OA given rats (Table 6).
The lung MPO activities (U/g of wet lung) of OA given rats were increased (p<0.001) to 33.38±10.44 (n=19) by OA compared with 9.96±2.64 (n=8) of control rats. The treatment of mepacrine (100 µM) resulted in the MPO activity of 35.98±10.53 (n=7) and did not change the increased MPO activities by OA (Table 6).
The present study was performed to know whether neutrophilic oxidative stress played a role in the pathogenesis of acute inflammatory edema in OA-induced ALI.
As is known, the exact pathogenesis of ARDS has not been explained, and accordingly the appropriate way of management of ARDS has not been established. Even if the pathological findings of full-blown ARDS shows very similar traits regardless of the various etiologies, still there is a possibility of diverse pathogeneses of ARDS according to their etiologies. In this regards, ARDS caused by pulmonary fat embolism is entitled to be studied meticulously to get a clue to understand the pathogenesis and a way of novel treatment of this notorious malady. In other words, we do not think ARDS is a single disease entity in spite of its identical pathological and physiological outcomes regardless of multiple etiologies.
In this point of view, we tried to figure out the role of neutrophils in OA-induced ARDS. Despite some authors16 insisted that neutrophilic oxidative stress had no functional, pathophysiological relationship with pathogenetic pathway of OA-induced ARDS, we have found opposite evidences to confirm the neutrophilic roles in OA-induced ALI.
Firstly, OA stimulated the generation of neutrophilic superoxide anion conspicuously and it was suppressed by the inhibition of PLA2 with mepacrine (100 µM). The effect of OA on the neutrophilic oxidative stress was confirmed again by the NBT test. OA increased NBT positive cells, which was inhibited by mepacrine (100 µM). As Dana et al17 demonstrated, the activation of PLA2 was prerequisite of neutrophilic superoxide generation. Therefore OA appeared to activate PLA2 of neutrophils causing the release of superoxide anion from neutrophils. This assumption was confirmed by cytochrome-c reduction assay and NBT test in this study. Very interestingly, in our neutrophil aggregation test, OA-induced neutrophilic aggregation and respiratory burst. In microscopic examination of the aggregates, it was revealed that the formazan positive neutrophils were intermingled with minute OA-droplets. These findings are thought to be very important to understand the pathogenesis of OA-induced ALI, and one step forward, fat embolism-induced ARDS. If fat droplets in the capillaries, induces chemotaxis of neutrophils into the microvasculatures and is followed by respiratory burst, these processes definitely ensues the destruction of alveolar and endothelial barriers very rapidly. As was confirmed in our study, OA was chemotactic and provoked the generation of superoxide anion, which could promote the oxidative stress in pulmonary capillaries. Thus OA is the instigator of neutrophilic oxidative stress in the pulmonary capillaries and the initiator of pulmonary edema, at least in OA-induced ARDS.
As Repine18 pointed out, localized, abrupt and explosive generation of free radicals from neutrophils can't be opposed or neutralized by antioxidant system in the body. In OA-induced ALI, the OA-droplets block the pulmonary capillaries, attract neutrophils and make aggregates which are the massive source of the generation of superoxide anion.
Secondly, the role of PLA2 is also crucial for the evoking of OA-induced ARDS. Long before, Lee et al19 stressed that PLA2 is the pivot of the inflammatory cascade during ARDS. Similarly, Furue et al20 demonstrated that PLA2 was crucial in OA-induced ARDS in rabbits. In our present study, mepacrine suppressed the generation of superoxide anion from neutrophils, resulted in the suppression of oxidative stress and decreased the number of NBT positive neutrophils. Even if mepacrine (100 µM) suppressed the respiratory burst, it could not prevent the accumulation of neutrophils in the microvessels because it did not have the resolving activity on chemotactic OA droplets. As was pointed out, OA is a chemotactic, especially to neutrophils, which was the reason why OA increased lung MPO activity. The increased lung MPO activity was not decreased by mepacrine because of the chemotactic property of OA. Only the production of superoxide anion was suppressed by mepacrine by way of the inhibition of PLA2.
In association with OA-induced neutrophilic aggregation, another possible assumption is that the neutrophilic plug induced pulmonary hypertension. Young and his colleagues21 demonstrated that the strong antihypertensive nitroprusside ameliorated pulmonary edema and pulmonary hypertension in OA-induced ALI. In conjunction with our experimental evidences to Young's report, it is highly probable that OA induced neutrophilic aggregation in microvasculature which blocks the capillary lumen, then increases hydrostatic pressure causing the pulmonary edema. In the present study, the inhibition of PLA2 with mepacrine suppressed the formation of the aggregates.
From these results, we would like to propose that oxidants and OA were the initiator of the formation of neutrophilic clump in the microvessels. When we take into account of Vadasz's report22 that OA inhibition of alveolar fluid absorption, the OA's role to cause pulmonary edema is much more evident in association with neutrophilic oxidative stress. It's analagous to increase pressure into the punctured tube increases the output of the fluid through the holes but not through the impermeable walls.
Thirdly, in our in vivo study, OA increased lung MPO activity. Since OA is regarded as a chemotactic material for neutrophils, the increased accumulation of neutrophils in the lung is natural. The accumulated neutrophils are thought to be the source of free radicals causing oxidative stress, thus the increased amount of protein in BALF after OA is not unexpected. By the inhibition of PLA2 with mepacrine, the generation of superoxide anion was diminished and followed by the decrease of protein content in BALF. Although mepacrine did not decrease the MPO activity in OA-induced ALI, it decreased the migration of the neutrophils into the alveolar lumen. Thus the inhibition of PLA2 appears to decrease the oxidative stress in OA-induced ALI.
Taken together, on the basis of our present study, we concluded that neutrophilic oxidative stress played a pivotal role in OA-induced ALI in Sprague-Dawley rats. And some appropriate interventions to decrease the aggregation and oxidative stress of neutrophils could lead to the novel modalities for prevention and cure of the OA-induced ALI.
Figures and Tables
Figure 1
Microscopic finding of OA-neutrophil aggregate after NBT reaction in vitro. Massive accumulation of formazan-positive neutrophils (asterisk) was noted at the vicinities of OA droplets (arrows). Original magnification ×100.
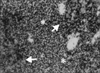
Figure 2
Morphological evidence of chemotactic activity of OA on neutrophils. Around OA droplets (asterisk), dense accumulation of formazan positive neutrophils was noted. Almost all the neutrophils were formazan positive. The accumulation of neutrophils at the vicinities of OA droplets was much more dense compared with that of the remote area from OA droplets (arrow head). Original magnification ×40.
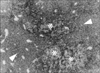
References
1. Ware LB, Matthay MA. The acute respiratory distress syndrome. N Engl J Med. 2000. 342:1334–1349.
2. Bernard GR. Acute respiratory syndrome: a historical perspective. Am J Respir Crit Care Med. 2005. 172:798–806.
3. Zhou Z, Kozlowski J, Schuster DP. Physiologic, biochemical, and imaging characterization of acute lung injury in mice. Am J Respir Crit Care Med. 2005. 172:344–351.
4. Liu H, Zhang D, Zhao B, Zhao J. Superoxide anion, the main species of ROS in the development of ARDS induced by oleic acid. Free Radical Res. 2004. 38:1281–1287.
5. Hill LL, Chen DL, Kozlowski J, Schuster DP. Neutrophils and neutrophil products do not mediate pulmonary hemodynamic effects of endotoxin on oleic acid-induced lung injury. Aneth Analg. 2004. 98:452–457.
6. Tighe D, Moss R, Boghossian S, Heath MF, Chessum B, Bennett ED. Multi-organ damage resulting from experimental faecal peritonitis. Clin Sci (Lond). 1989. 76:269–276.
7. Dana R, Malech HL, Levy R. The requirement for phospholipase A2 for activation of the assembled NADPH oxidase in human neutrophils. Biochem J. 1994. 297:217–223.
8. Jaeschke H, Smith CW. Mechanisms of neutrophil-induced parenchymal cell injury. J Leukoc Biol. 1997. 61:647–653.
9. Bulger EM, Maier RV. Lipid mediators in the pathophysiology of critical illness. Crit Care Med. 2000. 28:N27–N36.
10. Babior BM. Phagocytes and oxidative stress. Am J Med. 2000. 109:33–44.
11. Haslett C, Guthrie LA, Kopaniak MM, Johnston RB Jr, Henson PM. Modulation of multiple neutrophil functions by preparative methods or trace concentrations of bacterial lipopolysaccharide. Am J Pathol. 1985. 119:101–110.
12. Botha AJ, Moore FA, Moore EE, Fontes B, Banerjee A, Peterson VM. Postinjury neutrophil priming and activation states: therapeutic challenges. Shock. 1995. 3:157–166.
13. Shiue S, Thrall RS. Effect of corticosteroid therapy on the acute lung injury and recovery stage of oleic acid induced lung injury in the rat. Exp Lung Res. 1991. 17:629–638.
14. Goldblum SE, Wu KM, Jay M. Lung myeloperoxidase as a measure of pulmonary leukostasis in rabbits. J Appl Physiol. 1985. 59:1978–1985.
15. Brown RE, Jarvis KL, Hyland KJ. Protein measurement using bicinchoninic acid: elimination of interfering substances. Anal Biochem. 1989. 180:136–139.
16. McGuigan RM, Mullenix P, Norlund LL, Ward D, Walts M, Azarow K. Acute lung injury using oleic acid in the laboratory rat: establishment of a working model and evidence against free radicals in the acute phase. Curr Surg. 2003. 60:412–417.
17. Dana R, Leto TL, Malech HL, Levy R. Essential requirement of cytosolic phospholipase A2 for activation of the phagocyte NADPH oxidase. J Biol Chem. 1998. 273:441–445.
18. Repine JE. Scientific perspectives on acute respiratory distress syndrome. Lancet. 1992. 339:466–469.
19. Lee YM, Hybertson BM, Terada LS, Repine AJ, Cho HG, Repine JE. Mepacrine decreases lung leak in rats given interleukin-1 intratracheally. Am J Respir Crit Care Med. 1997. 155:1624–1628.
20. Furue S, Kuwabara K, Mikawa K, Nishina K, Shiga M, Maekawa N, et al. Crucial role of group IIA phospholipase A2 in oleic acidinduced acute lung injury in rabbits. Am J Respir Crit Care Med. 1999. 160:1292–1302.
21. Young JS, Rayhrer CS, Edmisten TD, Cephas GA, Tribble CG, Kron IL. Sodium nitroprusside mitigates oleic acid-induced acute lung injury. Ann Thorac Surg. 2000. 69:224–227.
22. Vadász I, Morty RE, Kohstall MG, Olschewski A, Grimminger F, Seeger W, et al. Oleic acid inhibits alveolar fluid reabsorption: a role in acute respiratory distress syndrome? Am J Respir Crit Care Med. 2005. 171:469–479.