Abstract
Background
Epigenetic alterations in certain genes are now known as at least important as genetic mutation in pathogenesis of cancer. Especially abnormal hypermethylation in or near promoter region of tumor suppressor genes (TSGs) are known to result in gene silencing and loss of gene function eventually. The authors tried to search for new lung cancer-specific TSGs which have CpG islands and HpaII sites, and are thought to be involved in carcinogenesis by epigenetic mechanism.
Methods
Tumor tissue and corresponding adjacent normal tissue were obtained from 10 patients who diagnosed with non small cell lung cancer (NSCLC) and underwent surgery in Konyang university hospital in 2005. Methylation profiles of promoter region of 21 genes in tumor tissue & non-tumor tissue were examined with HpaII-MspI methylation microarray (Methyl-Scan DNA chip®, Genomic tree, Inc, South Korea). The rates of hypermethylation were compared in tumor and non-tumor group, and as a normal control, we obtained lung tissue from two young patients with pneumothorax during bullectomies, methylation profiles were examined in the same way.
Lung cancer is the leading cause of cancer-related mortality worldwide attributing 1 million deaths every year1. There has been no effective screening tool with survival benefit2,3, leading to search for novel, non-invasive method for early detection of lung cancers. Early detection includes the identification of lung cancer-specific biomarkers.
Pathogenesis of cancer is having been elucidated gradually as genetics and molecular biology evolves. Epigenetic alteration of genes with their rolls of tumor suppression is one of the central mechanisms of tumorigenesis other than genetic mutation. This promising field has been being researched for more than two decades, and methylation of the CpG islands in or near of promoter region of tumor suppressor genes is major composition of epigenetic mechanism. When methylation occurs, some other events such as deacetylations of histone residues readily accompany, and conformational change of DNA inhibits transcriptional factors and some proteins from binding for the transcription to be initiated. And loss of function of the tumor suppressor genes (TSGs), for example, is resulted without alterations of genetic sequences4-6. Epigenetic alterations are changes that could be reversed by demethylating agents such as azacitidine and some results from several studies on these agents with some kinds of cancers disclosed the effectiveness in survival rate and symptom relief, so application into clinical field was just started5.
In the present study authors hypothesized that if a gene could be a TSG by epigenetic mechanisms, more frequent methylation profiling of that gene should be observed in tumor tissue than adjacent non-tumor tissue in a subject, or in tissue from cancer-free control group. So we investigated methylation profiles of genes in nonsmall cell lung cancer (NSCLC) patients and compared with corresponding adjacent non-tumor lung tissues. We tried to search for non-small cell lung cancer-specific tumor suppressor genes of which their function is inhibited by epigenetic mechanism.
Total of 10 patients with primary NSCLC who underwent lung surgeries in 2005 in Konyang university hospital were included. This study was approved by the Bioethics Committee of Konyang University Hospital and all of the participants gave written informed consent. All of the tumor and macroscopically normal lung tissue samples were obtained at the time of surgery, and were rapidly frozen in liquid nitrogen and stored at -80℃ until analysis. Also, normal lung tissue was obtained from two young healthy male patients with pneumothoraces through their bullectomies. Tissues samples were histologically confirmed by hematoxylin-eosin staining. DNA was extracted and prepared using the QiaAmp DNA mini kit® (Qiagen, Hilden, Germany).
Methylation status of each promoter was detected by presence or absence of hybridization signals after PCR using samples digested with HpaII (methylation-sensitive enzyme) and MspI (Methylation-resistant enzyme). The hybridization signals on the Methyl-Scan DNA microarray are proportional to PCR status (Figure 1).
To make the microarray, we have chosen 50 genes (Apaf-1, ACCN1, APC, AR, BRCA1, CALCA, CALCR, CDH13, CDKN2A, CDKN2B, CFTR, COMT, DAPK1, EBR, EDN1, EPHA3, EPO, ESR1, FHIT, H19, hMLH1, HPSE, HTR1B, IL-8, JunB, LAMA5, LDHB, LRP2, LTB4R, MDR3. MGMT, MTHFR, MUC2, PGR, PIK3CG, PLS3, PTGS2, RAR-b, RB1, S100A2, SHP1, SKT11, SLC5A5, SMARCA3, SRBC, TFF1, TP73, TUSC3, VHL, and WT1) which have promoter regions for HpaII searched in NCBI database.
The purified DNA (100 ng for each digestion) was digested with HpaII (20 units / 2 uL) and MspI (40 units / 2 uL), respectively, and then genomic DNA was re-digested with same enzymes to ensure complete digestion and reduce the background noise signals. Each digestion was followed by incubation at 37℃ for 2 hours and 15 hours, respectively. The second digested samples were purified with GeneClean Turbo kit® (Qbio, gene, Baton Rouge, LA, USA) according to the manufacturer's instructions. To ensure scanning, we used exogenous GAPDH cDNA as control.
Multiplex PCR amplification was done with un-digested and HpaII-, MspI-digested DNA with 9 primer sets to label 50 target promoter regions. During amplification step, fluorescent dyes were incorporated into the amplicons; Cy3-dUTP in MspI-digested targets, Cy5-dUTP in HpaII-digested targets, and undigested samples labeled with Cy5-dUTP by same multiplex PCR.
After PCR amplification all the amplicons were mixed, microarray hybridization was performed at 65℃ for 4 hours in the humidified chamber (GenomicTree, Daejeon, South Korea). The hybridized microarray was imaged by scanner (Axon Instruments, CA, USA). If the signal intensity of HpaII amplicon is 2-fold greater than that of MspI amplicon, the target region was considered to be methylated, while less than 2-fold was considered to be unmethylated.
There were 5 male and 5 female patients, the mean age was 62.9 years old. All of 5 male patients had smoking history with forty six of mean pack-years, two of them had squamous cell carcinoma, and the remainders had adenocarcinomas. 5 female patients were all non-smokers, 3 of them had adenocarcinomas, one had squamous cell carcinoma, and the remainder had other kind of NSCLC, respectively (Table 1).
The methylation microarray assay was performed for tumor tissue and adjacent normally-appearing tissue using HpaII-MspI methylation microarray. The PCR was successful for 21 genes (APC, AR, BRCA1, CALCA, CDH13, CFTR, EDN1, EPHA3, H19, HTR1B, LAMA5, LDHB, LTB4R, MTHFR, MUC2, PIK3CG, PTGS2, RAR-b, S100A2, SHP1, and SRBC) from 50 genes, others were not available because of PCR failure (Figure 2). APC, AR, RAR-b, HTR1B, EDHA3, CFTR and were hypermethylated in tumor tissue than adjacent normally appearing tissue. CDH13, CALCA, LAMA5, MTHFR, PIC3CG, S100A2, SHP1, EDN1, H19, MUC2, and LTB4R were commonly methylated in tumor and non-tumor sample and we found that these genes were hypermethylated in normal control, as well (Figure 3). Among the rest of genes excluding aforementioned eleven genes, four genes (SRBC, LDHB, BRCA1, and PTGS2) were not methylated in tumor tissue. Then six genes, APC, AR, RAR-b, HTR1B, EPHA3, and CFTR, finally turned out to be more frequently methylated in tumor tissue and unmethylated in non-tumor tissue and control group (Table 2).
The results for 22 genes including control (IFN) from four patients among subjects with gel electrophoresis were completely identical with those of DNA microarray (Figure 4).
Aberrant promoter hypermethylation in the field of cancer has been researched vigorously for two decades. After hematologic malignancies with this mechanism and efficacy of administration of demethylating agents were studied, many other solid tumors are having been under investigation for years. Present studies are focusing largely on identification of TSGs functioning as DNA repair genes, cell cycle regulatory genes or chromosomal loci by epigenetic mechanism, and some of them more frequently methylated in specific cancers seem to be possible candidates7-10. While, hypermethylation profiles of several genes are being proposed as prognostic markers11-13.
With the recent development of large-throughput analysis technology, assays allowing CpG island methylation to be analyzed at a genome level were developed. Oligonucleotide-based methylation assays using PCR after bisulfite treatment were developed by Adorjan et al.14 and Shi et al.15 Methylation assays using DNA microarray, first introduced in 2001 by Yan et al.16, include a method comprising modifying the cytosine of genomic DNA into uracil, amplifying the modified DNA, polymerizing the amplification product into oligonucleotide or DNA-oligomer, and hybridizing the polymer in a DNA microarray17-19. To show the high accuracy of the method, we performed gel electrophoresis on IFN, which doesn't have a promoter region for HpaII, as a control. The results of gel electrophoresis were exactly identical with that of microarray. We suggest that microarray could be a promising method for mass screening in the future, with extended range of genes.
In the present study, CDH13, CALCA, LAMA5, MTHFR, PIC3CG, S100A2, SHP1, EDN1, H19, MUC2, and LTB4R were commonly in all three group; Among them CHD13, CALLA and EDN1 were described in previous studies to have hypermethylated promoter regions and relation with lung cancer; Takai et al. reported that DNA fragment B3 was hypermethylated in 14 of 20 (70%) primary cancers, and decreased EDN1 (endothelin-1) expression was observed in 16 of 20 (80%)20. In the meanwhile, in present study, EDN1 was hypermethylated in tumor and non-tumor tissue with equal frequency of 60%, and in all of the control tissue. Hypermethylation of the promoter of CHD13, a gene encoding H-cadherin, was frequently associated with silencing in a few cancers, including lung cancer21-23. Kim et al. reported the frequency of hypermethylation in tumor and non-tumor tissue is 26 of 88 tissue samples (29.5%) and 7 of 88 tissue samples (8%), respectively24. In present study, frequency of methylation of tumor and non-tumor tissue was 40%, and 30%, respectively with hypermethylation in all from control group. CALLA (NEP; CD10, EC 3.4.24.11) is a gene for cell surface endopeptidase inactivating the autocrine growth factors and inhibiting cell proliferation, and decreased activation of this gene have been found in lung cancers25-27. There could be some considerations for hypermethylation in normal tissue seen in present study; (1) the method with enzyme could have been too sensitive, that allowed false-positive results; (2) the expressions of genes are controlled by normal methylation process during the course of embryogenesis and development, as 'aging process', and the persistently functioning genes in adults are localized to specific tissue for specific functions28-30, that some of the genes above could be normally silenced by methylation in lung tissue.
Findings from our present study showed the 6 genes of APC, AR, CFTR, EPHA3, HTR1B, and RAR-b more frequently hypermethylated in tumor tissue than corresponding normal tissue or control, which suggests that their methylation may be a tumor-restricted event. And AR, APC and RAR-b are well-known to be TSGs silenced with epigenetic alteration for NSCLC from the previous studies7,31-35, that CFTR, EPHA3 and HTR1B are worthy to be studied further as possible candidates of TSGs by epigenetic mechanism for NSCLC.
Cystic fibrosis transmembrane regulator gene (CFTR) is known to be related to chronic idiopathic pancreatitis, a well known risk factor of pancreatic cancer and there were several studies to show that mutation of CFTR (Δ F508) would be associated with malignancies, including pancreatic cancer36,37. While, recently a few novel functions of CFTR has been reported other than as ion channels in airway epithelial cells in cystic fibrosis36-38; (1) As part of a multiprotein complex at the cell surface, CFTR might interfere with the expression of several gene products, and participate in the signaling pathways of inflammatory response conjunctly with NF-κB, mitogen-activated protein kinase/extracellular signal-regulated kinase (MAPK/ERK) and activated protein-1 (AP-1). And when AP-1 is acting associated with transcription co-factors which promote cell proliferation and inhibit apoptosis, this would result in respiratory epithelium carcinogenesis39. (2) it also permeate anti-oxidant-reduced glutathione (GSH) out of epithelial cells, resulting in the low level of intracellular glutathione, that leads to insufficient protection from oxidative stress such as smoking, and damage and apoptosis of cells occur. This mechanism is related with high cellular level of BAX which is a pro-apoptotic member of Bcl-2 family, and in cystic fibrosis which has CFTR mutation, the level of BAX would be lowered.
Recently, Brantley et al.40 reported that EPHA2 and EPHA3, which are in soluble form, are thought to have a role in inhibiting angiogenesis and tumor growth, and treatment with soluble EphA2-Fc or EphA3-Fc receptors inhibited tumor angiogenesis in cutaneous window assays, and tumor growth in vivo, in the report of the first functional evidence for Eph A class receptor regulation of pathogenic angiogenesis induced by tumors and support the function of EPHA receptors in tumor progression. As far as we know, there is no study undertaken on hypermethylation of promoter region of EPHA3 with decreased expression.
Takai et al.20 reported aberrant hypermethylation of CpG island in the promoter region of HTR1B (hydroxy-tryptamine receptor 1B) was seen in two squamous lung cancer cell lines, EBC-a and LK-2, while it was demethylated in normal human bronchial endothelium (NHBE). And when LK-w cells were treated with 5-aza-2-deoxycytidine, H6 fragment was demethylated and expression of HTR1B was restored. Maybe due to the heterogeneity of clinical samples of both lung cancer tissue and normal tissue, the results of methylation profile and expression of HTR1B was not exactly consistent with that with lung cancer cell lines. The role of HTR1B silencing in lung carcinogenesis is not clear but the authors suggested that it is of great interest to examine the role of serotonin and its signaling from its type B receptors.
Some limits in this study was the small number of patients and subject genes, but this study showed hypermethylation profiles of several genes that could be TSGs of lung cancer with microarray, which is not widely used in Korea yet, with high accuracy of the method.
DNA microarray has strengths of rapidity, mass analysis, easy to managing and also, high accuracy, suggesting the role of screening tool for cancers with DNA hypermethylation profiles as novel biologic markers. In the present study, CpG islands of 6 genes including APC, AR, CFTR, EPHA3, HTR1B, and RAR-b were hypermethylated in tumor tissue than tumor adjacent tissue or control group which was assessed with microarray. The results from the present studies and previous reports on CFTR, EPHA3 and HTR1B support that these genes possibly be novel candidates of TSGs in NSCLC. The functional study for each candidate gene is required for the confirmation of the genes as TSG by hypermethylation mechanism. Further study with this design with larger number of patients and whole human genes would be required.
Figures and Tables
Figure 1
Schematic diagram of methylation microarray. Purified genomic DNA is digested with HpaII and MspI. If the genomic DNA has hypermethylation in CpG islands of promoter region, HpaII is sensitive to methylation status, whereas MspI is still insensitive. Multiplex PCR amplification is accomplished with un-digested and HpaII-, MspI-digested DNA. Each of 9 promoter regions can be amplified simultaneously in a single tube using 9 specific primer pairs, and for 50 promoters and control, 6 sets of PCR are required. During amplification step, fluorescent dyes (Cy3- or Cy5-dUTP) are incorporated into the amplicons. After PCR amplification, all amplicons are mixed and hybridized on Methyl-Scan DNA chip, on which 50 specific promoter regions are spotted. When we observe the hybridization signal of HpaII-amplicon is 2.0-fold greater than MspI-amplicon's signal, we considered 'methylated'.
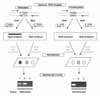
Figure 2
Genes commonly methylated in three groups. There were 11 commonly methylated genes; CDH13, CALCA, LAMA5, MTHFR, PIC3CG, S100A2, SHP1, EDN1, H19, MUC2, and LTB4R.
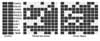
Figure 3
Genes of hypermethylation profiles in tumor tissue. Promoter regions of 6 genes of APC, AR, RAR-b, HTR1B, EPHA3, and CFTR were more frequently methylated in tumor tissue than non-tumor tissue and not methylated in control group.
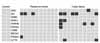
Figure 4
Validation of microarray with gel electrophoresis. An example of gel electrophoresis (patient 1). The signal of CFTR was revealed 'negative' in normal tissue (dashed arrow) and 'positive' in tumor tissue (solid arrow) by microarray. And the same results were seen in gel electrophoresis; undigested sample (first lane) only seen in normal tissue (A) and undigested and HpaII-digested sample (second lane) seen with the absence of third lane for tumor tissue (B).
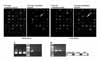
References
1. Jemal A, Siegel R, Ward E, Murray T, Xu J, Thun MJ. Cancer statistics, 2007. CA Cancer J Clin. 2007. 57:43–66.
2. Ellis JR, Gleeson FV. Lung cancer screening. Br J Radiol. 2001. 74:478–485.
3. Marcus PM. Lung cancer screening: an update. J Clin Oncol. 2001. 19:83S–86S.
4. Jones PA, Baylin SB. The fundamental role of epigenetic events in cancer. Nat Rev Genet. 2002. 3:415–428.
5. Worm J, Guldberg P. DNA methylation: an epigenetic pathway to cancer and a promising target for anticancer therapy. J Oral Pathol Med. 2002. 31:443–449.
6. Baylin SB. DNA methylation and gene silencing in cancer. Nat Clin Pract Oncol. 2005. 2:Suppl 1. S4–S11.
7. Esteller M, Corn PG, Baylin SB, Herman JG. A gene hypermethylation profile of human cancer. Cancer Res. 2001. 61:3225–3229.
8. Zöchbauer-Müller S, Minna JD, Gazdar AF. Aberrant DNA methylation in lung cancer: biological and clinical implications. Oncologist. 2002. 7:451–457.
9. Palmisano WA, Divine KK, Saccomanno G, Gilliland FD, Baylin SB, Herman JG, et al. Predicting lung cancer by detecting aberrant promoter methylation in sputum. Cancer Res. 2000. 60:5954–5958.
10. Park JC, Chae YK, Son CH, Kim MS, Lee J, Ostrow K, et al. Epigenetic silencing of human T (brachyury homologue) gene in non-small-cell lung cancer. Biochem Biophys Res Commun. 2008. 365:221–226.
11. Gu J, Berman D, Lu C, Wistuba II, Roth JA, Frazier M, et al. Aberrant promoter methylation profile and association with survival in patients with non-small cell lung cancer. Clin Cancer Res. 2006. 12:7329–7338.
12. Harden SV, Tokumaru Y, Westra WH, Goodman S, Ahrendt SA, Yang SC, et al. Gene promoter hypermethylation in tumors and lymph nodes of stage I lung cancer patients. Clin Cancer Res. 2003. 9:1370–1375.
13. Kim DH, Kim JS, Ji YI, Shim YM, Kim H, Han J, et al. Hypermethylation of RASSF1A promoter is associated with the age at starting smoking and a poor prognosis in primary non-small cell lung cancer. Cancer Res. 2003. 63:3743–3746.
14. Adorján P, Distler J, Lipscher E, Model F, Müller J, Pelet C, et al. Tumour class prediction and discovery by microarray-based DNA methylation analysis. Nucleic Acids Res. 2002. 30:e21.
15. Shi H, Maier S, Nimmrich I, Yan PS, Caldwell CW, Olek A, et al. Oligonucleotide-based microarray for DNA methylation analysis: principles and applications. J Cell Biochem. 2003. 88:138–143.
16. Yan PS, Chen CM, Shi H, Rahmatpanah F, Wei SH, Caldwell CW, et al. Dissecting complex epigenetic alterations in breast cancer using CpG island microarrays. Cancer Res. 2001. 61:8375–8380.
17. Fukasawa M, Kimura M, Morita S, Matsubara K, Yamanaka S, Endo C, et al. Microarray analysis of promoter methylation in lung cancers. J Hum Genet. 2006. 51:368–374.
18. Hatada I, Kato A, Morita S, Obata Y, Nagaoka K, Sakurada A, et al. A microarray-based method for detecting methylated loci. J Hum Genet. 2002. 47:448–451.
19. Hou P, Ji M, He N, Lu Z. Microarray-based method to evaluate the accuracy of restriction endonucleases HpaII and MspI. Biochem Biophys Res Commun. 2004. 314:110–117.
20. Takai D, Yagi Y, Wakazono K, Ohishi N, Morita Y, Sugimura T, et al. Silencing of HTR1B and reduced expression of EDN1 in human lung cancers, revealed by methylation-sensitive representational difference analysis. Oncogene. 2001. 20:7505–7513.
21. Kim JS, Han J, Shim YM, Park J, Kim DH. Aberrant methylation of H-cadherin (CDH13) promoter is associated with tumor progression in primary nonsmall cell lung carcinoma. Cancer. 2005. 104:1825–1833.
22. Sato M, Mori Y, Sakurada A, Fujimura S, Horii A. The H-cadherin (CDH13) gene is inactivated in human lung cancer. Hum Genet. 1998. 103:96–101.
23. Toyooka KO, Toyooka S, Virmani AK, Sathyanarayana UG, Euhus DM, Gilcrease M, et al. Loss of expression and aberrant methylation of the CDH13 (H-cadherin) gene in breast and lung carcinomas. Cancer Res. 2001. 61:4556–4560.
24. Kim DS, Kim MJ, Lee JY, Kim YZ, Kim EJ, Park JY. Aberrant methylation of E-cadherin and H-cadherin genes in nonsmall cell lung cancer and its relation to clinicopathologic features. Cancer. 2007. 110:2785–2792.
25. Bunn PA Jr, Helfrich BA, Brenner DG, Chan DC, Dykes DJ, Cohen AJ, et al. Effects of recombinant neutral endopeptidase (EC 3.4.24.11) on the growth of lung cancer cell lines in vitro and in vivo. Clin Cancer Res. 1998. 4:2849–2858.
26. Cohen AJ, Franklin WA, Magill C, Sorenson J, Miller YE. Low neutral endopeptidase levels in bronchoalveolar lavage fluid of lung cancer patients. Am J Respir Crit Care Med. 1999. 159:907–910.
27. Kristiansen G, Schluns K, Yongwei Y, Dietel M, Petersen I. CD10 expression in non-small cell lung cancer. Anal Cell Pathol. 2002. 24:41–46.
28. Jones PA, Takai D. The role of DNA methylation in mammalian epigenetics. Science. 2001. 293:1068–1070.
29. Sachan M, Raman R. Developmental methylation of the regulatory region of HoxB5 gene in mouse correlates with its tissue-specific expression. Gene. 2006. 380:151–158.
30. Yung RL, Julius A. Epigenetics, aging, and autoimmunity. Autoimmunity. 2008. 41:329–335.
31. Tsoli E, Gorgoulis VG, Zacharatos P, Kotsinas A, Mariatos G, Kastrinakis NG, et al. Low levels of p27 in association with deregulated p53-pRb protein status enhance tumor proliferation and chromosomal instability in non-small cell lung carcinomas. Mol Med. 2001. 7:418–429.
32. Virmani AK, Tsou JA, Siegmund KD, Shen LY, Long TI, Laird PW, et al. Hierarchical clustering of lung cancer cell lines using DNA methylation markers. Cancer Epidemiol Biomarkers Prev. 2002. 11:291–297.
33. Winters ZE. P53 pathways involving G2 checkpoint regulators and the role of their subcellular localisation. J R Coll Surg Edinb. 2002. 47:591–598.
34. Zöchbauer-Müller S, Fong KM, Virmani AK, Geradts J, Gazdar AF, Minna JD. Aberrant promoter methylation of multiple genes in non-small cell lung cancers. Cancer Res. 2001. 61:249–255.
35. Tsou JA, Hagen JA, Carpenter CL, Laird-Offringa IA. DNA methylation analysis: a powerful new tool for lung cancer diagnosis. Oncogene. 2002. 21:5450–5461.
36. Jacquot J, Tabary O, Le Rouzic P, Clement A. Airway epithelial cell inflammatory signalling in cystic fibrosis. Int J Biochem Cell Biol. 2008. 40:1703–1715.
37. Cantin AM, Bilodeau G, Ouellet C, Liao J, Hanrahan JW. Oxidant stress suppresses CFTR expression. Am J Physiol Cell Physiol. 2006. 290:C262–C270.
38. Shaulian E, Karin M. AP-1 in cell proliferation and survival. Oncogene. 2001. 20:2390–2400.
39. Karamouzis MV, Konstantinopoulos PA, Papavassiliou AG. The activator protein-1 transcription factor in respiratory epithelium carcinogenesis. Mol Cancer Res. 2007. 5:109–120.
40. Brantley DM, Cheng N, Thompson EJ, Lin Q, Brekken RA, Thorpe PE, et al. Soluble Eph A receptors inhibit tumor angiogenesis and progression in vivo. Oncogene. 2002. 21:7011–7026.