Abstract
Background
Single nucleotide polymorphisms (SNPs), which consist of a substitution of a single nucleotide pair, are the most abundant form of genetic variations occurring with a frequency of approximately 1 per 1000 base pairs. SNPs by themselves do not cause disease but can predispose humans to disease, modify the extent or severity of the disease or influence the drug response and treatment efficacy. Single nucleotide polymorphisms (SNPs), particularly those within the regulatory regions of the genes often influence the expression levels and can modify the disease. Studies examining the associations between SNP and the disease outcome have provided valuable insight into the disease etiology and potential therapeutic intervention. Traditionally, the genotyping of SNPs has been carried out using polymerase chain reaction-restriction fragment length polymorphism(PCR-RFLP), which is a low throughput technique not amenable for use in large-scale SNP studies. Recently, TaqMan real-time PCR chemistry was adapted for use in allelic discrimination assays. This study validated the accuracy and utility of real-time PCR technology for SNPs genotyping.
Methods
The SNPs in promoter sequence (-37 and -524) of lung cancer suppressor gene, RRM1 (ribonucleotide reductase M1 subunit) with the genomic DNA samples of 89 subjects were genotyped using both real-time PCR and PCR-RFLP.
Results
The discordance rates were 2.2% (2 mismatches) in -37 and 16.3% (15 mismatches) in -524. Auto-direct sequencing of all the mismatched samples(17 cases) were in accord with the genotypes read by real-time PCR. In addition, 138 genomic DNAs were genotyped using real-time PCR in a duplicate manner (two separated assays). Ninety-eight percent of the samples showed concordance between the two assays.
Figures and Tables
![]() | Figure 1Allelotyping of RRM1 by restriction enzyme digestion.
(A) RRM1-37 C/A polymorphism. Restriction enzyme Bbs I cuts when -37 base is A. Homozygous A allelle type (lane 3 from left shows complete digestion into 156 bp bands. Homozygous C allele type (lane 4 and 7) have original 217 bp bands only without digestion. The primer dimers are seen in all the lanes that are barely distinguishable from 61 bp bands in lanes with CA or AA. (B) RRM1 -524 T/C polymorphism. Restriction enzyme Apo I cuts when -524 base is T. Homozygous T allelotype (lane 2, 5 and 6 from left) shows complete digestion into 70 bp bands. Homozygous C allele type (lane 3 and 4) have original 176 bp bands only without digestion.
|
![]() | Figure 2Allelotyping of RRM1 by real-time PCR (TaqMan probe assay) Allelic discrimination of genotyping by auto analysis software 6.0 program of Roter-Gene after RRM1 amplication. (A) genotype analysis result (B) scatter graph analysis result. |
Table 3
Comparison of allele frequency at -37 and -524 region of RRM1 gene between PCR-RFLP and RT-PCR. The results from RT-PCR were validated using Hardy-Weinberg equilibrium.
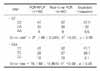
References
1. Shastry BS. SNP alleles in human disease and evolution. J Hum Genet. 2002. 47:561–566.
2. Syvanen AC. Accessing genetic variation: genotyping single nucleotide polymorphisms. Nat Rev Genet. 2001. 12:930–942.
3. Brookes AJ. The essence of SNPs. Gene. 1999. 234:177–186.
4. Collins FS, Brooks LD, Chakravarti A. A DNA polymorphism discovery resource for research on human genetic variation. Genome Res. 1998. 8:1229–1231.
5. Knudson AG. Hereditary predisposition to cancer. Ann N Y Acad Sci. 1997. 833:58–67.
6. Ohnishi Y, Tanaka T, Yamada R, Suematsu K, Minami M, Fujii K, et al. Identification of 187 single nucleotide polymorphisms(SNPs)among 41 candidate genes for ischemic heart disease in the Japanese population. Hum Genet. 2000. 106:288–292.
7. Kamide K, Kokubo Y, Yang J, Matayoshi T, Inamoto N, Takiuchi S, et al. Association of genetic polymorphisms of ACADSB and COMT with human hypertension. J Hypertens. 2006. 25:103–110.
8. Risch N, Merikangas K. The future of genetic studies of complex human diseases. Science. 1996. 273:1516–1517.
9. Sousa MC, Morais JB, Machado JE, Poiares-DA-Silva J. Genotyping of Giardia lamblia Human Isolates from Portugal by PCR-RFLP and Sequencing. J Eukaryot Microbiol. 2006. 53:174–176.
10. Hatzaki A, Razi E, Anagnostopoulou K, Iliadis K, Kodaxis A, Papaioannou D, et al. A modified mutagenic PCR-RFLP method for K-ras codon 12 and 13 mutations detection in NSCLC patients. Mol Cell Probes. 2001. 15:243–247.
11. Bravo-Villalta HV, Yamamoto K, Nakamura K, Baya A, Horiuchi R. A novel intronic mutation that may affect genotyping result of CYP2C8 by PCR-RFLP is strongly associated with CYP2C8*3 in a South American population. J Hum Genet. 2006. 52:195–199.
12. Ruiz-Ponte C, Carracedo A, Barros F. Duplication and deletion analysis by fluorescent real-time PCR-based genotyping. Clin Chim Acta. 2006. 363:138–146.
13. Gibson NJ. The use of real-time PCR methods in DNA sequence variation analysis. Clin Chim Acta. 2006. 363:32–47.
14. Joneson VJ, Yucesoy B, Luster MI. Genotyping of single nucleotide polymorphisms in cytokine genes using real-time PCR allelic discrimination technology. Cytokine. 2004. 27:135–141.
15. Bepler G, Zheng Z, Gautam A, Sharma S, Cantor A, Sharma A, et al. Ribonucleotide reductase M1 gene promoter activity, polymorphisms, population frequencies, and clinical relevance. Lung cancer. 2005. 47:183–192.
16. Ueda T, Ugawa S, Ishida Y, Shibata Y, Murakami S, Shimada S. Identification of coding single nucleotide polymorphisms in human taste recepter genes involving bitter tasting. Biochem Biophys Res Commun. 2001. 285:147–151.
17. Shi MM. Technologies for individual genotyping: detection of genetic polymorphisms in drug targets and disease genes. Am J Pharmacogenomics. 2002. 2:197–205.
18. Abraham J, Earl HM, Pharoah PD, Caldas C. Pharmacogenetics of cancer chemotherapy. Biochim Biophys Acta. 2006. 1766:168–183.
19. Shi MM, Bleavins MR, de la iglesia FA. Technologies for detecting genetic polymorphisms in pharmacogenomics. Mol Diagn. 1999. 4:343–351.
20. Lazarus R, Vercelli D, Palmer LJ, Klimecki WJ, Silverman EK, Richter B, et al. Single nucleotide polymorphisms in innate immunity genes: abundant variation and potential role in complex human disease. Immunol Rev. 2002. 190:9–25.
21. Palmer LJ, Cookson WO. Using single nucleotide polymorphisms as a means to understanding the pathophysiology of asthma. Respir Res. 2001. 2:102–112.
22. Iannone MA, Taylor JD, Chen J, Li MS, Rivers P, Slentz-Kesler KA, et al. Multiplexed single nucleotide polymorphism genatyping by oligonucleotide ligation and flow cytometry. Cytometry. 2000. 39:131–140.
23. Blievernicht JK, Schaeffeler E, Klein K, Eichelbaum M, Schwab M, Zanqer UM. MALDI-TOF mass spectrometry for multiplex genotyping of CYP2B6 single nucleotide polymorphisms. Clin Chem. 2007. 53:24–33.
24. Podder M, Welch WJ, Zamar RH, Tebbutt SJ. Dynamic variable selection in SNP genotype autocalling from APEX microarray data. BMC Bioinformatics. 2006. 7:521.
25. Jobs M, Howell WM, Stromqvist L, Mayr T, Brookes AJ. DASH-2: flexible, low-cost, and high throughput SNP genotyping by dynamic allele-specific hybridization on membrane arrays. Genome Res. 2003. 13:916–924.
26. Shen GO, Luo A, Wang OK. High-throughput single nucleotide polymorphisms genotyping: TaqMan assay and pyrosequencing assay. Methods Mol Med. 2006. 128:209–224.