Abstract
Figures and Tables
![]() | Figure 1Relationship of fasting glucose with oxidative stress and inflammation status. The relationships were tested by Pearson and partial correlation analyses; tested after log-transformation; r0: correlation coefficient (unadjusted), r1: correlation coefficient (adjusted for age and gender), r2: correlation coefficient (adjusted for age, gender, cigarette smoking, alcohol consumption, and body mass index). LDL: low density lipoprotein, MDA-TBARS: malondialdehyde-thiobarbituric acid-reactive substances, hs-CRP: high-sensitivity C-reactive protein, TNF-α: tumor necrosis factor-alpha, IL-6: interleukin-6. *p < 0.1; †p < 0.05; ‡p < 0.001, §p < 0.00001. |
![]() | Figure 2Association of fasting glucose and oxidative stress status in control individuals and patients with stroke. Values are presented as means ± standard errors. tested after log-transformation. (n) indicates the number of subjects in each group; tested by a general linear model followed by Bonferroni correction with adjustments for age, gender, cigarette smoking, alcohol consumption, and body mass index. NFG: 70-99mg/dL, HFG: 100-125 mg/dL. *p<0.1; †p<0.05; ‡p<0.001, compared with the corresponding NFG control individuals or patients with stroke; §p<0.05, compared with HFG control individuals. |
![]() | Figure 3Association of fasting glucose and inflammation status in control individuals and patients with stroke. Values are presented as means ± standard errors. tested after log transformation. (n) indicates the number of individuals in each group; tested by a general linear model followed by Bonferroni correction with adjustments for age, gender, cigarette smoking, alcohol consumption, and body mass index. NFG: 70-99 mg/dL, HFG: 100-125 mg/dL. hs-CRP: high-sensitivity C-reactive protein, IL-6: interleukin-6, TNF-α: tumor necrosis factor-alpha. *p<0.05; †p<0.001; compared with the corresponding NFG control individuals or patients with stroke; §p<0.05, compared with NFG control individuals; ‡p<0.05, compared with HFG control individuals. |
Table 1
General characteristics of the study participants
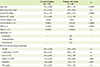
Data are means ± S.E. or percentage (%). p-values tested by Student's t-test. n.s. indicates not statistically significant.
BP: blood pressure, TEE: total energy expenditure (kcal/day), TEI: total energy intake (kcal/day), PUFA: polyunsaturated fatty acid (g/day), SFA: saturated fatty acid (g/day).
*Obesity: body mass index ≥ 25 kg/m2.
Table 2
Basic biochemical parameters, oxidative stress, and inflammatory markers in control individuals and patients with stroke
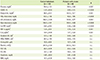
Data are means ± S.E. n.s. indicates not statistically significant.
HDL: high density lipoprotein, LDL: low density lipoprotein, hs-CRP: high-sensitivity C-reactive protein, TNF-α: tumor necrosis factor-alpha, IL-6: interleukin-6, MDA-TBARS: malondialdehyde-thiobarbituric acid-reactive substances, AST: aspartate aminotransferase, ALT: alanine aminotransferase, BUN: blood urea nitrogen.
*Tested after log-transformation, p-values tested by Student's t-test.
Table 3
Basic parameters and lipid profiles according to fasting glucose status in control individuals and patients with stroke
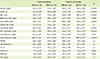
Data are means ± S.E. Values with the same letter are not significantly different. (n) indicates the number of subjects in each group. n.s. indicates not statistically significant. NFG (normal fasting glucose): 70-99mg/dL, HFG (higher fasting glucose): 100-125 mg/dL, Sharing the same alphabet indicates no statistical significance between the values in the same row. BP: blood pressure, n.s.: no significance, HDL: high density lipoprotein, LDL: low density lipoprotein, TEE: total energy expenditure (kcal/day), TEI: total energy intake (kcal/day), PUFA: polyunsaturated fatty acid (g/day), SFA: saturated fatty acid (g/day).
*Tested after log-transformation. Tested by one-way analysis of variance followed by Bonferroni correction.
Acknowledgements
Notes
Authors' contributions All the authors were involved in the development of the study protocol and the experimental design. All the authors read, commented on, and contributed to the submitted manuscript. Sample collection and experiments were performed by JHL, SRY GYN, MJ, MRA, JKC and OYK. Data were analyzed by JHL, SRY, GYN and OYK. The manuscript was written by JHL and OYK, and finalized by OYK. None of the authors have any conflicts of interest in relation to the materials presented in this paper.
References
















