Abstract
Input signals originating from baroreceptors and vestibular receptors are integrated in the rostral ventrolateral medulla (RVLM) to maintain blood pressure during postural movement. The contribution of baroreceptors and vestibular receptors in the maintenance of blood pressure following hypotension were quantitatively analyzed by measuring phosphorylated extracellular regulated protein kinase (pERK) expression and glutamate release in the RVLM. The expression of pERK and glutamate release in the RVLM were measured in conscious rats that had undergone bilateral labyrinthectomy (BL) and/or sinoaortic denervation (SAD) following hypotension induced by a sodium nitroprusside (SNP) infusion. The expression of pERK was significantly increased in the RVLM in the control group following SNP infusion, and expression peaked 10 min after SNP infusion. The number of pERK positive neurons increased following SNP infusion in BL, SAD, and BL+SAD groups, although the increase was smaller than seen in the control group. The SAD group showed a relatively higher reduction in pERK expression when compared with the BL group. The level of glutamate release was significantly increased in the RVLM in control, BL, SAD groups following SNP infusion, and this peaked 10 min after SNP infusion. The SAD group showed a relatively higher reduction in glutamate release when compared with the BL group. These results suggest that the baroreceptors are more powerful in pERK expression and glutamate release in the RVLM following hypotension than the vestibular receptors, but the vestibular receptors still have an important role in the RVLM.
The maintenance of blood pressure is controlled by a complex mechanism including the baroreceptor and vestibulosympathetic reflexes. The baroreceptor reflex is a feedback control system for maintaining blood pressure during hemorrhage, exercise and postural changes. Baroreceptors in the carotid sinus and aortic arch are stretched by increasing blood pressure, which results in their activation. Baroreceptors in the carotid sinus send afferent signals to the nucleus tractus solitarius (NTS) via the glossopharyngeal nerve, and other baroreceptors in the aortic arch transmit similar information via the vagus nerve to the NTS. Neurons from the NTS project to the caudal ventrolateral medullary nucleus (CVLM) and nucleus ambiguus. The CVLM projects inhibitory neurons to the rostral ventrolateral medullary nucleus (RVLM), an area containing presympathetic neurons that innervate the intermediolateral cell column in the spinal cord [1,2].
Vestibular stimulation through postural changes elicits the activation of the sympathetic nervous system to control blood pressure, which is referred to as the vestibulosympathetic reflex [3,4]. The excitation of vestibular receptors through postural changes induces functional changes to many components of the cardiovascular system, including blood pressure, pulse rate, baroreceptor reflex and blood flow to the extremities [5]. The caudal portion of the medial vestibular nucleus and the inferior vestibular nucleus receive afferent signals from the otolith organs as well as the semicircular canals, and project to the NTS, CVLM and RVLM [6,7,8,9]. The NTS receives primary inputs from cardiovascular, gastrointestinal, and pulmonary afferents and key components of the central pathway regulating the sympathetic vasomotor activity [10]. In addition, the NTS integrates afferent signals from baroreceptors and vestibular receptors to control blood pressure [11].
The RVLM has direct connections with the vestibular nucleus and receives signals from baroreceptors through the CVLM [9]. The RVLM is involved in regulating the activity of the sympathetic tone and integrates afferent signals from vestibular receptors and baroreceptors. Low blood pressure causes lower activation of baroreceptors, inhibition of the CVLM, and eventually increases sympathetic tone through disinhibition of the RVLM [12]. Previous studies in our laboratory have found that both vestibular receptors and baroreceptors could cooperate with each other during acute hypotension to facilitate the maintenance of blood pressure [11,13]. Many kinds of amino acid neurotransmitters are present in the RVLM; glutamate is the principal excitatory neurotransmitter in the sympathetic premotor pathway from the RVLM to the sympathetic preganglionic neurons in the thoracic spinal cord [14].
Orthostatic hypotension results from an impaired capacity to increase vascular resistance during postural change from a lying position to a standing position in patients with autonomic failure or bilateral vestibular loss. An impairment of vascular resistance leads to increased downward pooling of venous blood, and a consequent reduction in stroke volume and cardiac output exaggerates the orthostatic fall in blood pressure [15,16]. However, in normal subjects, reduction in blood pressure evokes a baroreceptor reflex and postural changes stimulate the vestibular receptors [17,18]. Thus, both the baroreceptor and vestibular receptor cooperate with each other to maintain blood pressure during postural changes, however the quantitative role of the baroreceptor and vestibular receptor on blood pressure regulation during hypotension is less well understood.
Extracellular regulated protein kinase (ERK) is activated via phosphorylation in response to various excitatory stimuli, such as membrane depolarization, glutamate, and electrical stimulation in neurons [19]. Fos method has commonly been used to identify central neurons that respond to specific stimuli [20]. However, expression of pERK was measured in this study since pERK can be expressed in multiple subcellular compartments [21] by inhibition as well as excitation [22] and by stimuli lasting second-to-minutes [23]. By contrast, expression of c-Fos protein requires intense and sustained activation, since it should be transcribed and translated from the immediate-early gene c-fos. Therefore, measurement of pERK expression is valuable for analyzing an acute response following hypotension in the RVLM. A previous study in our laboratory has shown that sodium nitroprusside (SNP)-induced hypotension increases the expression of pERK in the vestibular nuclei, which indicates that hypotension elicits activation of vestibular nuclear neurons [24].
In this study, we sought to clarify the quantitative role of vestibular receptors and baroreceptors on the regulation of blood pressure during hypotension. To this end, we analyzed the expression of pERK and glutamate release in the RVLM using immunohistochemical and microdialysis methods, respectively, in conscious rats that received bilateral labyrinthectomy (BL) and/or baroreceptor unloading via sinoaortic denervation (SAD).
Adult male Sprague-Dawley rats (Changchun, China) weighing 220~250 g were used in this study. Efforts were made to minimize the number of animals used and suffering. Experimental animals were divided into four groups for immunohistochemical analysis (n=6/group) and for microdialysis (n=6/group): the control group, in which both the sinoaortic baroreceptors and vestibular receptors were intact; bilateral labyrinthectomy (BL) group, in which the sinoaortic baroreceptors were intact, but a bilateral labyrinthectomy was performed; sinoaortic denervation (SAD) group, in which the sinoaortic baroreceptors were denervated, but the vestibular receptors remained intact; and BL+SAD group, in which both the bilateral vestibular receptors and sinoaortic baroreceptors were removed. All animal protocols and procedures were performed in accordance to the rules and regulations by the Animal Research Committee of the Yanbian University.
A chemical labyrinthectomy was performed as described previously [25]. After anesthesia with isoflurane (Ilsung Co., Seoul, Korea), 100 µL of sodium arsanilate (100 mg· mL-1) was injected intratympanically into the bilateral middle ear of the rats, which chemically destroyed the membranous labyrinth. The destruction of epithelial cells in peripheral vestibular receptors was confirmed by confocal microscope after rhodamine-palloidin staining [26]. As a control, saline was injected intratympanically in the sham and SAD rats. The labyrinthectomies were performed 48 hours prior to experimentation.
After anesthesia with isoflurane, the carotid sinus nerve was sectioned bilaterally following a midventral incision in the neck. The internal, external, and common carotid arteries were stripped off connective tissue at the level of bifurcation and painted with 10% phenolethanol to denervate the carotid sinus. For aortic arch denervation, the aortic arch nerve was severed bilaterally proximal to its junction with the vagus nerve [27]. In the sham and BL groups, rats received similar cervical incisions leaving nerves, vessels, and baroreceptors intact. After surgery, the animals were breathing spontaneously without significant changes in respiratory rhythm. SAD was performed 48 hours prior to experimentation. In case of BL+SAD group, labyrinthectomy and sinoaortic denervation were performed simultaneously 48 hours prior to experimentation.
Two heparinized polyethylene tubes were inserted into the femoral artery to record blood pressure and into the femoral vein for sodium nitroprusside (SNP) infusion, under isoflurane anesthesia. The tubes were guided toward the skull percutaneously, fixed into the skull, and connected to the tubes of a cybernation metabolism cage to allow free movement in a conscious state during the experiment. Blood pressure was recorded from the unilateral femoral artery using a pressure transducer and physiography (Grass model 7400; USA). SNP was infused for 1 min at a dose of 15 µg·kg-1·min-1, decreasing blood pressure by 30~40 mmHg during this period.
After deep anesthesia with an intraperitoneal injection of sodium pentobarbital (100 mg·kg-1), animals were sacrificed for immunohistochemical analysis of ERK at 10, 20, 40 or 60 min following administration of SNP. The rats were perfused transcardially with 500 mL of 0.9% NaCl, fixed with 4% paraformaldehyde, and the sucrose-embedded brain stem was sectioned on a cryostat. After nonspecific binding sites were blocked with normal goat serum, primary anti-rabbit polyclonal anti-pERK 1/2 antibody (1:1000; Cell Signaling Technology; MA, USA) was applied to tissue sections overnight at 4℃. Thereafter, tissue sections were incubated with a biotinylated goat anti-rabbit secondary antibody (1:200; Vector Lab., Burlingame, CA, USA) and then an avidin-biotin complex. The bound complex was visualized by incubating the tissue with diaminobenzadine plus H2O2. Sections were then dehydrated, cleared in xylene, and cover-slipped with Permount (Fisher Scientific; Pittsburgh, PA, USA). For quantification, pERK 1/2-immunopositive neurons in the RVLM were counted using a digital image analysis system (Image-Pro; Media Cybernetics; MD, USA) at four different levels in a rostral-to-caudal continuum [28].
Rats were anesthetized with isoflurane and placed in a stereotaxic frame (Narishige, Japan). The skull was exposed and a microdialysis probe (Terumo, Japan) was stereotaxically implanted into the RVLM (12.7 mm from the bregma, AP 7.3 mm, ML 2.4 mm), according to a rat atlas [28]. Two polyethylene tubes inserted into the femoral vessels and a microdialysis probe were connected to the tubes of the cybernation metabolism cage to allow free movement in a conscious state during the experiment. The RVLM was perfused with modified Ringer's solution (147 mmol·L-1 NaCl, 4 mmol·L-1 KCl, 2.3 mmol·L-1 CaCl2; pH 6.5) through the implanted microdialysis probe at a constant rate of 1.5 µL·min-1 using a microsyringe pump (ESP-64; Eicom, Japan). The dialysate was collected in an Eppendorf tube using a fraction collector (EFC-82, Eicom, Japan) every 10 min. Previous experiments show that the concentration of amino acids becomes stable after 90 min [29]. After a 90 min stabilization period, samples of dialysate were collected. All samples of collected dialysates were kept at -80℃ for later analysis. Glutamate levels were measured using HPLC with an electrochemical detector system (HPLC-ECD, Japan) according to the precolumn derivatization method [30]. We prepared a 2 µmol·L-1 standard solution [13.31 mg L-Asp, 14.71 mg L-Glu, 14.62 mg L-glutamine, 7.51 mg Gly, 12.51 mg Tau, and 8.91 mg L-Ala in 50 mL of 0.1 mol/L HCl; diluted 1000 times in ACSF] and a 40 mmol/L o-phthalaldehyde (OPA) solution [13.5 mg OPA and 10 µL 2-mercaptoethanol in 2.5 mL of 0.1 mol·L-1 K2CO3 buffer (pH 9.5) with 10% ethanol]. The solutions were stored at ~0~4℃, and the OPA solution was diluted in 0.1 mol·L-1 K2CO3 buffer to yield a 4 mol·L-1 OPA solution immediately prior to analysis. The dialysate (12 µL) was mixed with 3 µL of 4 mmol·L-1 OPA solution and allowed to react for 2.5 min at 25℃. After completing the reaction, 10 µL of the reaction mixture was manually injected into a high-performance liquid chromatography (HPLC) system with an Eicompak MA-5 ODS column (4.6 mm inner diameter ×150 mm; Eicom, Japan) to assay the amino acids. We used electrochemical detection (ECD; Eicom, Japan) with +700 mV Ag/AgCl electrodes. The mobile phase (0.1 mol·L-1 NaH2PO4·2H2O, 0.1 mol·L-1 Na2HPO4·12H2O, 30% MeOH, 0.5 mmol·L-1 EDTA·2Na, adjusted to pH 6.0) was delivered at 1.0 mL·min-1 through an HPLC pump, and the ECD potential was set at +700 mV.
At the end of the in vivo microdialysis study, animals were sacrificed under deep anesthesia with an intraperitoneal injection of sodium pentobarbital. The brains were removed and fixed in 10% neutral phosphate-buffered formalin. After 3 days, the brains were placed in a 30% sucrose solution and cut into 50-µm sections to ascertain the location of the tip of the dialysis probe using a cryomicrotome (Wheel microtome KD1508, China). Sections were stained with neutral red and then examined using light microscopy. Only the data obtained from animals in which the microdialysis probe was positioned in the appropriate dialysis site were processed and included in the results. Other samples were discarded (Fig. 1).
To minimize between-subject variation, glutamate levels are expressed as percentages of the mean basal level for each animal. We defined the mean basal level as the mean level of 3 samples of dialysate collected 10 min apart, beginning 90 min after the start of perfusion through the microdialysis probe. Stat View version 11.5 software for SPSS (SPSS Inc, USA) was used for all statistical procedures. All data are expressed as the mean±standard deviation (SD). The statistical significance of differences was assessed using a one-way ANOVA followed by the least-significant difference test for multiple comparisons [Fisher's least-significant differences test (LSD) protected t-test]. Values of p<0.05 were considered statistically significant.
The baseline mean arterial pressure in control, BL, SAD, and BL+SAD groups was not different; 103.6±7.2, 97.8±10.8, 98.5±10.1, and 94.9±9.2 mmHg in control, BL, SAD, and BL+SAD groups, respectively. Intravenous infusion of SNP decreased blood pressure within 1 min of the beginning of the infusion, and hypotension was maintained for 2 min after the infusion. Infusion of SNP resulted in a significant decrease in mean arterial pressure in all four groups; 71.7±7.3, 65.1±6.7, 70.8±6.9, and 68.2±5.5 mmHg in control, BL, SAD, and BL+SAD groups, respectively, when compared with the non-SNP groups (p<0.01). There was no significant difference in mean arterial pressure between control and experimental groups following SNP infusion (Fig. 2).
The RVLM showed a few pERK-immunoreactive neurons at resting condition in rats with intact vestibular receptors and baroreceptors. The number of pERK neurons was 2.1±0.5 in rats with a saline infusion. However, SNP-induced hypotension increased the number of pERK in the RVLM (56.6±8.1, 28.3±3.8, 15.2±3.3, and 3.6±2.3 at 10, 20, 40, and 60 min after SNP infusion, respectively). The number of pERK neurons peaked 10 min after SNP infusion, and then decreased gradually. The number of pERK neurons was analyzed 10 min after saline or SNP infusion in BL, SAD, and BL+SAD groups. Saline infusion groups showed 1.2±0.7, 1.3±0.5, 6.6±2.1, and 6.4±2.4 pERK neurons in control, BL, SAD, and BL+SAD groups, respectively. However, SNPinduced hypotension increased the number of pERK neurons to 56.6±8.1, 40.3±3.1, 25.7±2.4, and 16.5±1.8 in control, BL, SAD, and BL+SAD groups, respectively. The level of pERK neurons in the BL group significantly decreased when compared with the control group (p<0.01), and the SAD group showed a significant reduction in the number of pERK neurons when compared with the BL group (p<0.01). Considering the number of pERK neurons in the RVLM, approximately 40.3 pERK neurons were activated by inputs mainly originating from the baroreceptors without vestibular receptors following hypotension and approximately 25.7 pERK neurons were activated by inputs mainly originating from the vestibular receptors without baroreceptors. And approximately 16.5 pERK neurons were activated by inputs originating from others except the vestibular receptors and baroreceptors (Fig. 3, 4).
There was no significant difference in the level of glutamate release in the RVLM between control and experimental groups before SNP infusion. SNP-induced hypotension increased the level of glutamate release in the RVLM. In the control group, glutamate release increased to 207.5±74.3% and 192.3±69.0% of the base level at 10 and 20 min, respectively, after SNP infusion. The level of glutamate release peaked 10 min after SNP infusion and returned to control levels 50 min. In the BL group, glutamate release increased to 172.4±61.4% and 168.4±68.3% of the base level at 10 and 20 min, respectively, after SNP infusion. In the SAD group, glutamate release increased to 122.1±48.1% and 121.6±44.9% of the basal level at 10 and 20 min, respectively, after SNP infusion. However, the BL+SAD group did not show any significant increase in glutamate release after SNP infusion. The level of glutamate release in the control group was the highest among all 4 groups, and the BL group showed more glutamate release than the SAD group. The level of glutamate release peaked 10 min after SNP infusion in all groups (Fig. 5).
In the present study, we found that: (1) SNP-induced hypotension increased expression of pERK and glutamate release in the RVLM of control, BL, SAD, and BL+SAD groups; (2) the number of pERK neurons and the level of glutamate release in the RVLM after SNP infusion were higher in the BL group than in the SAD group; (3) expression of pERK and glutamate release peaked 10 min after SNP infusion in all 4 groups.
Patients with bilateral vestibular loss complain of orthostatic hypotension [15], and animals with bilateral vestibular loss have reduced blood pressure when body posture changes from a lying to a standing position [17]. A reduction in blood pressure causes peripheral vasospasm, which is a sympathetic effect via the baroreceptor reflex, thus leading to ischemic conditions in the labyrinth, which cause vertigo, a symptom of orthostatic hypotension [31]. The vestibulosympathetic reflex prevents orthostatic intolerance during postural movement by activation of the sympathetic nervous system. Although both the baroreceptors and vestibular receptors are sensitive to blood pressure changes, the vestibular receptors are more sensitive to low blood pressure than high blood pressure, whereas the baroreceptors regulate blood pressure during both hypotension and hypertension [13].
The RVLM contains many barosensitive neurons, and is activated by low blood pressure and inhibited by high blood pressure. Disinhibition of the RVLM by low blood pressure causes an increase in sympathetic activity and increases norepinephrine release in the heart and blood vessels, which increases blood pressure [32]. The RVLM receives afferent inputs from vestibular receptors via the vestibular nuclei and baroreceptors via the CVLM, and projects to sympathetic neurons; therefore, measurement of RVLM activity in BL and SAD animals is valuable for quantitatively analyzing the individual role of vestibular receptors and baroreceptors in the regulation of blood pressure.
Resting mean arterial pressure in the present study was not changed by BL or SAD, which was consistent with our previous study [13]. Theoretically, removal of the baroreceptor input should result in a hypertension since the inhibitory inputs from the CVLM are abolished [1]. However, when the influence of baroreceptors is removed, cardiopulmonary receptors and skeletal muscle pumps may assume an important role [33]. SNP decreases mean arterial pressure in all 4 groups, which is resulting from the release of nitric oxide [34].
The small amount of pERK expression in the RVLM of control animals indicates that the RVLM did not receive any significant afferent signals in conscious animals during the experiment. Expression of pERK or glutamate release in the RVLM is known to be affected by anesthesia; therefore, conscious animals were used during all experiments in this study [35]. SNP-induced hypotension increased pERK expression in the RVLM, and this expression peaked 10 min after SNP infusion and decreased gradually with time. The number of pERK neurons in the RVLM following hypotension was higher in BL group than in SAD group, which represents inputs originating from the baroreceptors are higher than inputs originating from the vestibular receptors. pERK expression in the RVLM following hypotension in BL+SAD animals was markedly reduced, which was slightly greater than observed in the saline control animals, indicating that some residual activation of neurons in the RVLM occurred even in the absence of vestibular receptor and baroreceptor inputs. The residual activation of neurons in the RVLM could be explained as a consequence of the unloading of vagus-innervated cardiopulmonary receptors in response to the hypotensive stimuli or cardiac sympathetic afferents [11,33,36]. These results are consistent with our previous study which described that BL animals showed increased expression of c-Fos protein in the NTS following hypotension when compared with SAD animals [11]. Activation of NTS and RVLM neurons following hypotension showed a similar response, as the NTS receives afferent signals from both the vestibular nuclei and baroreceptors and the RVLM receives afferent signals from both the vestibular nuclei and NTS via the CVLM. pERK immunohistochemistry in this study showed that RVLM neurons are mainly activated by the baroreceptors following hypotension, and less activated by the vestibular receptors.
Although the effect of vestibular receptor inputs on pERK expression in the RVLM is less than the baroreceptor inputs following hypotension, the vestibular receptor inputs may have an important role in maintenance of blood pressure and orthostatic hypotension. Our previous studies reported that acute hypotension induced by SNP infusion or hemorrhage increases neuronal activity, expression of c-Fos protein and pERK in the medial vestibular nuclei, but these effects are abolished following BL [24,37,38]. Therefore, reduced blood pressure stimulates the peripheral vestibular receptors, and then activation of RVLM neurons is induced by excitation of the vestibular nuclei.
Glutamate is a major excitatory neurotransmitter in the vestibulomedullary neurons that arise from the vestibular nuclei [9], and the bulbospinal neurons arising from the RVLM that contribute to the regulation of cardiovascular function [39,40]. RVLM neurons receive excitatory inputs mediated by excitatory amino acid and inhibitory inputs mediated by GABA from peripheral receptors and higher centers in the brain [12]. There are many sources of glutamate inputs to the RVLM, including the parabrachial nucleus [41], pontine reticular formation [42], lateral tegmental field in the medulla [43], and paraventricular nucleus in the hypothalamus [44]. RVLM presympathetic neurons receiving excitatory glutamatergic synaptic inputs are activated by phasic stimulation of peripheral receptors and by tonic stimulation of inputs originating from supramedullary regions [44]. In this study, SNP-induced hypotension increased glutamate release in the RVLM with the level of glutamate release peaking 10 min after SNP infusion. Hypotension-induced glutamate release in the RVLM showed more reduction in SAD group than in BL group. These results are consistent with our results showing a greater reduction in pERK expression in the SAD group compared with the BL group. Although baroreceptors have a more powerful role in glutamate release in the RVLM following hypotension than the vestibular receptors, vestibular receptors still have an important role in glutamate release in the RVLM, particularly during postural movement. Therefore, it is postulated that one of major sources of glutamate inputs to the RVLM following hypotension is the vestibular receptors. Excitatory glutamate inputs to the RVLM have both phasic and tonic effects [44], but glutamate inputs from baroreceptors and vestibular receptors could be a phasic drive. These results are based on the evidence of direct projections from the caudal vestibular nuclei to the RVLM [9,45]. As hypotension, as well as postural movement, activates vestibular receptors, orthostatic hypotension caused by postural changes is closely related to the vestibular system.
In summary, SNP-induced hypotension increased pERK expression and glutamate release in the RVLM. Although baroreceptor inputs are predominant at increasing pERK expression and glutamate release in the RVLM following hypotension when compared with vestibular inputs, the vestibular inputs still have an important role in the maintenance of blood pressure, particularly during postural movement. But still we need double immunostaining study to identify excitatory or inhibitory neurons in which were expressed pERK in the RVLM following hypotension.
ACKNOWLEDGEMENTS
This work was supported by grant 31160212 from the National Natural Science Foundation of China.
References
1. Guyenet PG. The sympathetic control of blood pressure. Nat Rev Neurosci. 2006; 7:335–346. PMID: 16760914.


2. Pilowsky PM, Goodchild AK. Baroreceptor reflex pathways and neurotransmitters: 10 years on. J Hypertens. 2002; 20:1675–1688. PMID: 12195099.


3. Yates BJ. Vestibular influences on the sympathetic nervous system. Brain Res Brain Res Rev. 1992; 17:51–59. PMID: 1638275.


4. Yates BJ, Miller AD. Properties of sympathetic reflexes elicited by natural vestibular stimulation: implications for cardiovascular control. J Neurophysiol. 1994; 71:2087–2092. PMID: 7931504.


5. Normand H, Etard O, Denise P. Otolithic and tonic neck receptors control of limb blood flow in humans. J Appl Physiol (1985). 1997; 82:1734–1738. PMID: 9173934.
6. Porter JD, Balaban CD. Connections between the vestibular nuclei and brain stem regions that mediate autonomic function in the rat. J Vestib Res. 1997; 7:63–76. PMID: 9057160.


7. Balaban CD, Beryozkin G. Vestibular nucleus projections to nucleus tractus solitarius and the dorsal motor nucleus of the vagus nerve: potential substrates for vestibulo-autonomic interactions. Exp Brain Res. 1994; 98:200–212. PMID: 8050507.


8. Yates BJ, Grélot L, Kerman IA, Balaban CD, Jakus J, Miller AD. Organization of vestibular inputs to nucleus tractus solitarius and adjacent structures in cat brain stem. Am J Physiol. 1994; 267:R974–R983. PMID: 7524372.
9. Holstein GR, Friedrich VL Jr, Kang T, Kukielka E, Martinelli GP. Direct projections from the caudal vestibular nuclei to the ventrolateral medulla in the rat. Neuroscience. 2011; 175:104–117. PMID: 21163335.


10. Kalia M, Mesulam MM. Brain stem projections of sensory and motor components of the vagus complex in the cat: II. Laryngeal, tracheobronchial, pulmonary, cardiac, and gastrointestinal branches. J Comp Neurol. 1980; 193:467–508. PMID: 7440778.


11. Jiang X, Li LW, Lan Y, Yang YZ, Jin GS, Kim MS, Park BR, Jin YZ. Comparative analysis of vestibular receptor and baroreceptor inputs to the nucleus tractus solitarius following acute hypotension in conscious rats. Neurosci Lett. 2014; 563:70–74. PMID: 24486893.


12. Dampney RA. Functional organization of central pathways regulating the cardiovascular system. Physiol Rev. 1994; 74:323–364. PMID: 8171117.


13. Lan Y, Yang YZ, Jiang X, Li LW, Jin GS, Kim MS, Park BR, Jin YZ. Additive role of the vestibular end organ and baroreceptors on the regulation of blood pressure in rats. Korean J Physiol Pharmacol. 2013; 17:367–373. PMID: 23946697.


14. Morrison SF. Glutamate transmission in the rostral ventrolateral medullary sympathetic premotor pathway. Cell Mol Neurobiol. 2003; 23:761–772. PMID: 14514030.
15. Aoki M, Sakaida Y, Tanaka K, Mizuta K, Ito Y. Evidence for vestibular dysfunction in orthostatic hypotension. Exp Brain Res. 2012; 217:251–259. PMID: 22205233.


16. Smit AA, Halliwill JR, Low PA, Wieling W. Pathophysiological basis of orthostatic hypotension in autonomic failure. J Physiol. 1999; 519(Pt 1):1–10. PMID: 10432334.


17. Doba N, Reis DJ. Role of the cerebellum and the vestibular apparatus in regulation of orthostatic reflexes in the cat. Circ Res. 1974; 40:9–18. PMID: 4543723.


18. Mori RL, Cotter LA, Arendt HE, Olsheski CJ, Yates BJ. Effects of bilateral vestibular nucleus lesions on cardiovascular regulation in conscious cats. J Appl Physiol (1985). 2005; 98:526–533. PMID: 15475594.


19. Sgambato V, Pagès C, Rogard M, Besson MJ, Caboche J. Extracellular signal-regulated kinase (ERK) controls immediate early gene induction on corticostriatal stimulation. J Neurosci. 1998; 18:8814–8825. PMID: 9786988.


20. Morgan JI, Curran T. Stimulus-transcription coupling in the nervous system: involvement of the inducible proto-oncogenes fos and jun. Annu Rev Neurosci. 1991; 14:421–451. PMID: 1903243.


21. Koh YH, Ruiz-Canada C, Gorczyca M, Budnik V. The Ras1-mitogen-activated protein kinase signal transduction pathway regulates synaptic plasticity through fasciclin II-mediated cell adhesion. J Neurosci. 2002; 22:2496–2504. PMID: 11923414.


22. Xia Z, Dudek H, Miranti CK, Greenberg ME. Calcium influx via the NMDA receptor induces immediate early gene transcription by a MAP kinase/ERK-dependent mechanism. J Neurosci. 1996; 16:5425–5436. PMID: 8757255.


23. Bobrovskaya L, Odell A, Leal RB, Dunkley PR. Tyrosine hydroxylase phosphorylation in bovine adrenal chromaffin cells: the role of MAPKs after angiotensin II stimulation. J Neurochem. 2001; 78:490–498. PMID: 11483651.


24. Kim MS, Choi DO, Choi MA, Kim JH, Kim KY, Lee MY, Rhee JK, Chun SW, Park BR. Immunohistochemical detection of phosphorylated form of extracellular signal-regulated kinase 1/2 in rat vestibular nuclei following hemorrhagic hypotension. Neurosci Lett. 2004; 360:49–52. PMID: 15082176.


25. Hunt MA, Miller SW, Nielson HC, Horn KM. Intratympanic injection of sodium arsanilate (atoxyl) solution results in postural changes consistent with changes described for labyrinthectomized rats. Behav Neurosci. 1987; 101:427–428. PMID: 3606813.


26. Choi MA, Lee JH, Hwang JH, Choi SJ, Kim MS, Park BR. Signaling pathway of glutamate in the vestibular nuclei following acute hypotension in rats. Brain Res. 2008; 1229:111–117. PMID: 18639534.


27. Wei S, Lei M, Tong M, Ding J, Han Q, Xiao M. Acute baroreceptor unloading evokes Fos expression in anesthetized rat brain. Brain Res Bull. 2008; 76:63–69. PMID: 18395612.


28. Paxinos G, Watson C. The rat brain in stereotaxic coordinates. Orlando, FL, USA: Academic Press;2007.
29. Yu HL, An Y, Jiang HY, Jin QH, Jin YZ. Changes of amino acid concentrations in the rat medial vestibular nucleus following unilateral labyrinthectomy. Sheng Li Xue Bao. 2007; 59:71–78. PMID: 17294045.
30. Jin QH, Ueda Y, Ishizuka Y, Kunitake T, Kannan H. Cardiovascular changes induced by central hypertonic saline are accompanied by glutamate release in awake rats. Am J Physiol Regul Integr Comp Physiol. 2001; 281:R1224–R1231. PMID: 11557631.


31. Pirodda A, Brandolini C, Carlo Modugno G. Hypotension associated with autonomic dysfunction: a possible cause of vertigo? Med Hypotheses. 2004; 63:1086. PMID: 15504583.


32. Freeman R. Clinical practice. Neurogenic orthostatic hypotension. N Engl J Med. 2008; 358:615–624. PMID: 18256396.
33. Cornish KG, Gilmore JP. Sino-aortic denervation in the monkey. J Physiol. 1985; 360:423–432. PMID: 3989722.


34. Murad F. Cyclic guanosine monophosphate as a mediator of vasodilation. J Clin Invest. 1986; 78:1–5. PMID: 2873150.


35. Takamura H, Ichisaka S, Watanabe K, Toigawa M, Hata Y. Effects of anesthesia on immunohistochemical detection of phosphorylated extracellular signal-regulated kinase in cerebral cortex. J Neurosci Methods. 2008; 170:300–304. PMID: 18294698.


36. Guo ZL, Lai HC, Longhurst JC. Medullary pathways involved in cardiac sympathoexcitatory reflexes in the cat. Brain Res. 2002; 925:55–66. PMID: 11755900.


37. Park BR, Kim MS, Kim JH, Jin YZ. Effects of acute hypotension on neuronal activity in the medial vestibular nuclei of rats. Neuroreport. 2001; 12:3821–3824. PMID: 11726802.


38. Kim MS, Kim JH, Kry D, Choi MA, Choi DO, Cho BG, Jin YZ, Lee SH, Park BR. Effects of acute hypotension on expression of cFos-like protein in the vestibular nuclei of rats. Brain Res. 2003; 962:111–121. PMID: 12543461.


39. Singewald N, Zhou GY, Chen F, Philippu A. Corticotropinreleasing factor modulates basal and stress-induced excitatory amino acid release in the locus coeruleus of conscious rats. Neurosci Lett. 1996; 204:45–48. PMID: 8929974.


40. Zhou W, Fu LW, Guo ZL, Longhurst JC. Role of glutamate in the rostral ventrolateral medulla in acupuncture-related modulation of visceral reflex sympathoexcitation. Am J Physiol Heart Circ Physiol. 2007; 292:H1868–H1875. PMID: 17158649.


41. Len WB, Chan JY. Glutamatergic projection to RVLM mediates suppression of reflex bradycardia by parabrachial nucleus. Am J Physiol. 1999; 276:H1482–H1492. PMID: 10330230.
42. Krassioukov AV, Weaver LC. Connections between the pontine reticular formation and rostral ventrolateral medulla. Am J Physiol. 1993; 265:H1386–H1392. PMID: 8238426.


43. Barman SM, Gebber GL, Orer HS. Medullary lateral tegmental field: an important source of basal sympathetic nerve discharge in the cat. Am J Physiol Regul Integr Comp Physiol. 2000; 278:R995–R1004. PMID: 10749789.
44. Dampney RA, Horiuchi J, Tagawa T, Fontes MA, Potts PD, Polson JW. Medullary and supramedullary mechanisms regulating sympathetic vasomotor tone. Acta Physiol Scand. 2003; 177:209–218. PMID: 12608991.


45. Holstein GR, Friedrich VL Jr, Martinelli GP. Projection neurons of the vestibulo-sympathetic reflex pathway. J Comp Neurol. 2014; 522:2053–2074. PMID: 24323841.


Fig. 1
A photomicrograph showing the placement of microdialysis probe in the RVLM. Arrow indicates a location of microdialysis membrane in the right RVLM (12.7 mm from the bregma, AP 7.3 mm, ML 2.4 mm). White dotted circle indicates the area of RVLM. RVLM, rostral ventrolateral medulla.
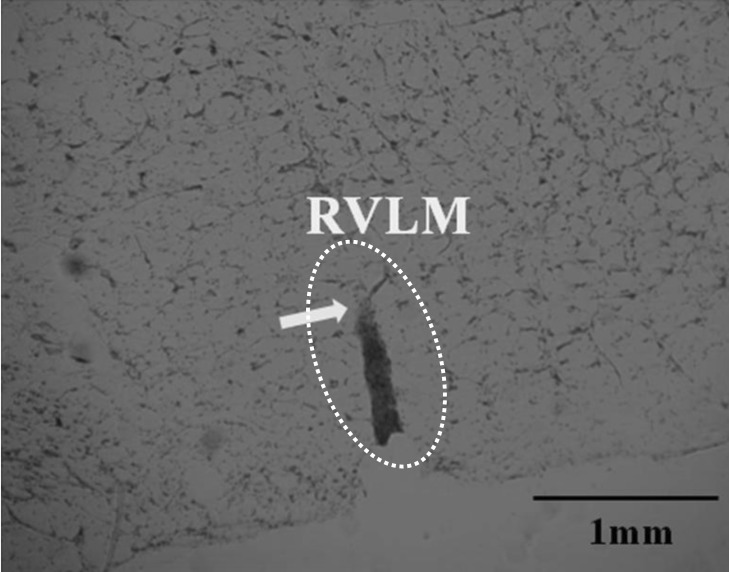
Fig. 2
Effect of sodium nitroprusside (SNP) infusion on mean arterial pressure. SNP was infused for 1 min at a dose of 15 µg·kg-1·min-1, decreasing blood pressure by 30~40 mmHg during this period. Control, control group; BL, bilateral labyrinthectomy group; SAD, sinoaortic denervation group; BL+SAD, bilateral labyrinthectomy and sinoaortic denervation group.
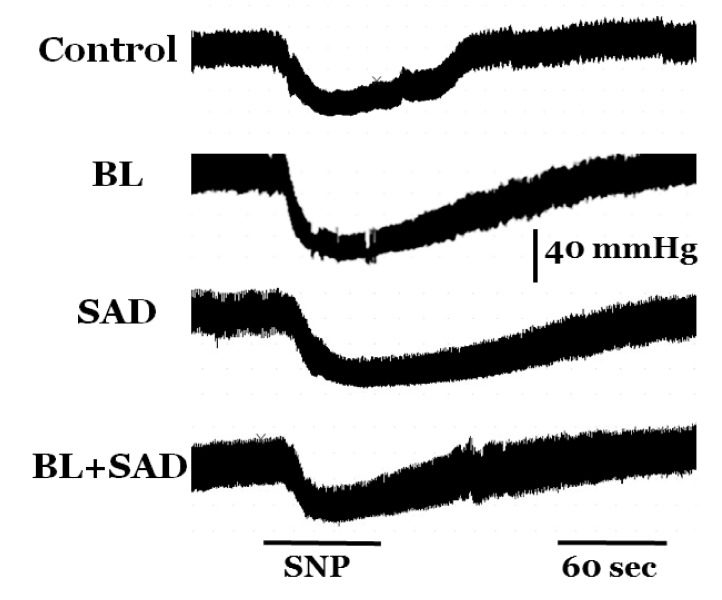
Fig. 3
Expression of pERK in the RVLM following a 30% reduction in blood pressure, induced by SNP, in conscious rats with intact labyrinths and baroreceptors. (A) Photomicrographs showing the effect of SNP-induced hypotension on the expression of pERK in the RVLM. Circular area indicates the RVLM (coordinates: Bregma 12.7 mm; ML 2.0 mm; depth 7.0 mm). (B) Number of pERK expression in the RVLM following SNP infusion. Controls received intravenous administration of saline at the same volume as SNP; Time indicates the number of minutes after SNP administration. There were 6 rats in each group. * indicates a significant difference from the control group (**p<0.01).
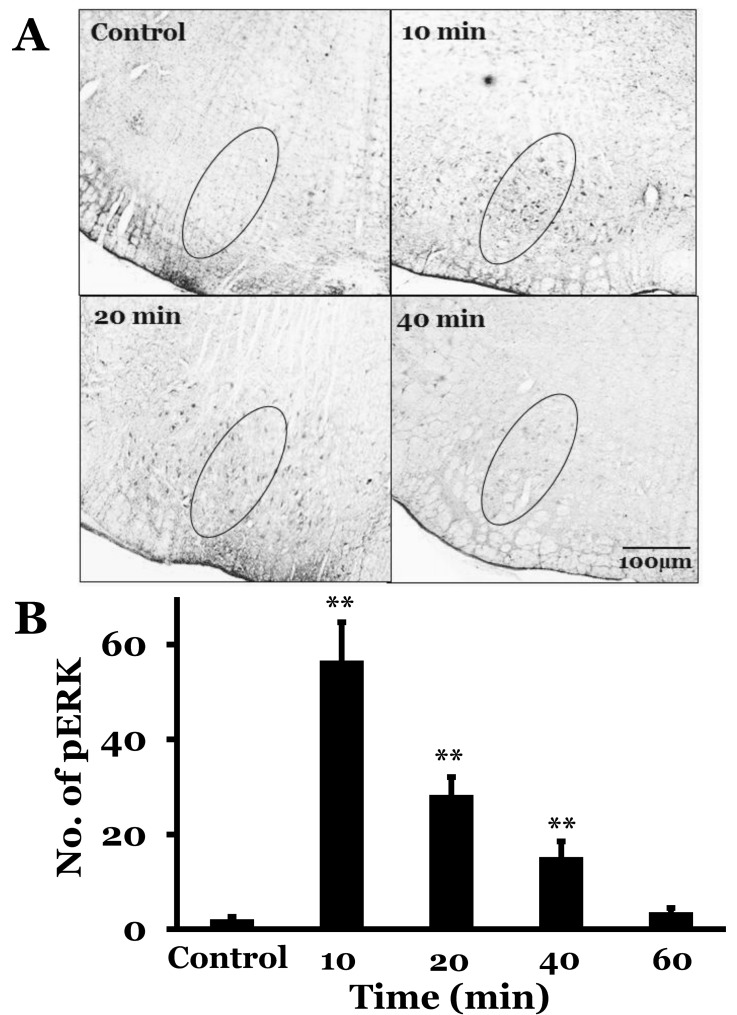
Fig. 4
The effect of SNP-induced hypotension on the expression of pERK in the RVLM in conscious rats with bilateral labyrinthectomy (BL), sinoaortic denervation (SAD), or both bilateral labyrinthectomy and sinoaortic denervation (BL+SAD). (A) Photomicrographs showing the effect of SNP-induced hypotension on the expression of pERK in the RVLM. Circular area indicates the RVLM (coordinates: Bregma 12.7 mm; ML 2.0 mm; depth 7.0 mm). (B) Number of pERK expression in the RVLM following SNP infusion. Control, control group. The expression of pERK was measured 10 min after SNP infusion. SNP, a 30% reduction in blood pressure that was induced by SNP infusion. Saline, intravenous administration of saline at the same volume as SNP. Number of rats was 6 in each group. * indicates a significant difference from the Control group (**p<0.01); ‡ indicates a significant difference from the BL group (‡p<0.01); and ≠indicates a significant difference from the SAD group (≠p<0.01).
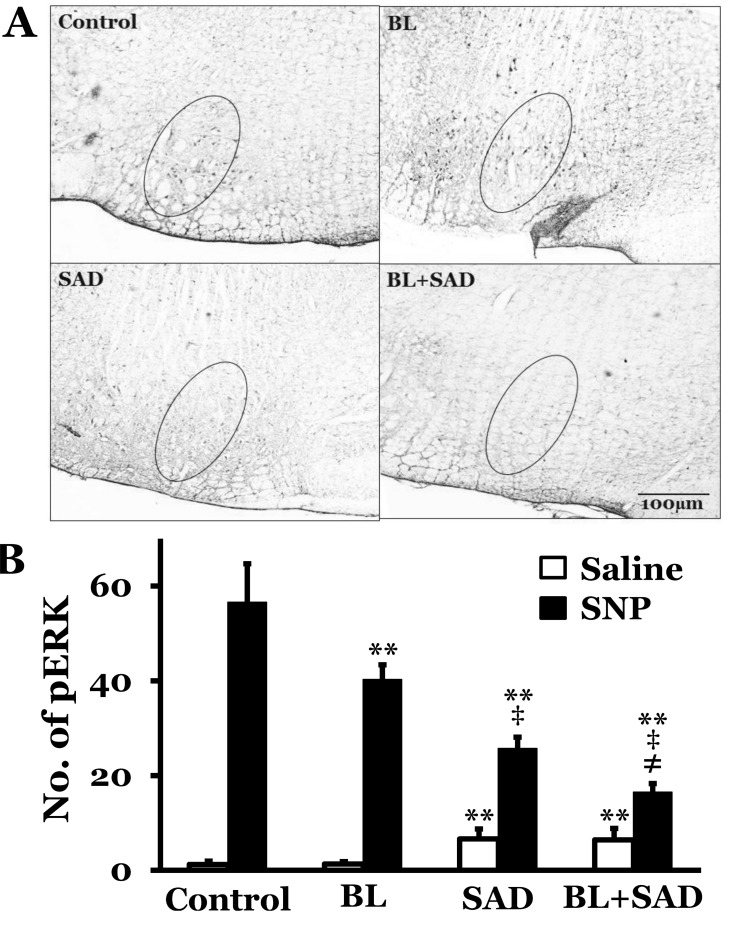
Fig. 5
The effect of SNP-induced hypotension on glutamate release in the RVLM of conscious rats with bilateral labyrinthectomy (BL), sinoaortic denervation (SAD), or both bilateral labyrinthectomy and sinoaortic denervation (BL+SAD). Arrow indicates SNP infusion. Control, control group. Number of rats was 6 in each group. * indicates a significant difference from the sham group (*p<0.05, **p<0.01).
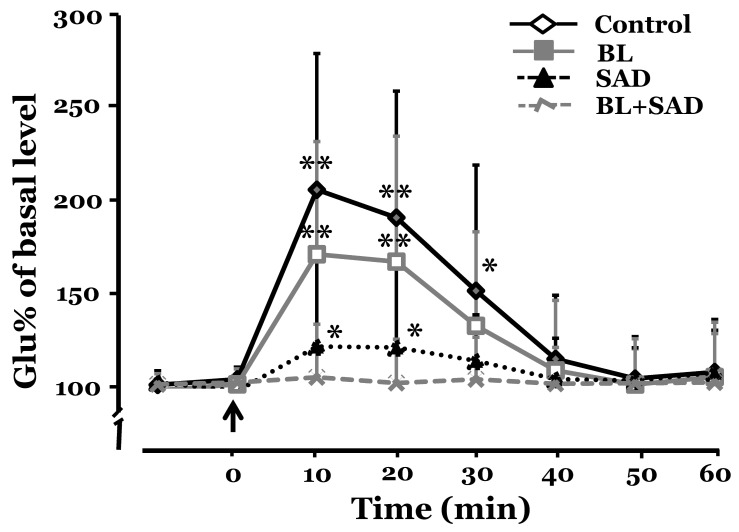