Abstract
Background
Microneedle is a method that creates transdermal microchannels across the stratum corneum barrier layer of skin. No previous study showed a therapeutic effect of microneedle itself on hair growth by wounding.
Objective
The aim of this study is to investigate the effect of repeated microwound formed by microneedle on hair growth and hair growth-related genes in a murine model.
Methods
A disk microneedle roller was applied to each group of mice five times a week for three weeks. First, to identify the optimal length and cycle, microneedles of lengths of 0.15 mm, 0.25 mm, 0.5 mm, and 1 mm and cycles of 3, 6, 10, and 13 cycles were applied. Second, the effect of hair growth and hair-growth-related genes such as Wnt3a, β-catenin, vascular endothelial growth factor (VEGF), and Wnt10b was observed using optimized microneedle. Outcomes were observed using visual inspection, real-time polymerase chain reaction, and immunohistochemistry.
Microneedle is a method that creates transdermal microchannels across the stratum corneum barrier layer of skin to increase the skin permeability of small-molecule drugs, protein, and vaccines1. In addition, drug delivery via microneedle is more reliable and consistent than oral routes because it avoids digestive degradation and liver metabolism23. In addition to the drug delivery effect, microneedle could cause microwounds in the skin, which induces the wound healing process1. Among the various types of microneedles, in contrast to conventional patch-like microneedles, a microneedle roller can be easily applied to large areas1. In addition, the efficacy of transdermal drug delivery using microneedle rollers has been shown4.
Hair follicles (HF) are important not only in hair regeneration but also in wound healing and skin regeneration5. The epidermal stem cells that reside in HF provide a substantial portion of wounded skin6. Wound regeneration is delayed in the absence of HF in a murine model7. Wounds heal faster in skin with HF in the anagen phase than in the telogen phase6. Conversely, Ito et al.8 reported that HF neogenesis occurs de novo via the Wnt signaling pathway following wounding in adult mice. A number of developmental pathways are recapitulated during both wound healing and HF cycling6. Above findings suggest that the wound healing process could induce HF regeneration, which is defined as process of HF reentering anagen9.
Although some clinical trials were done using the drug delivery effect of microneedle on patients with alopecia, no previous study showed the therapeutic effect of microneedle itself on hair growth by wounding11011. We hypothesized that microwounds formed by repeated microneedle stimulation could induce hair growth. To investigate the therapeutic effect of microneedle, we conducted a series of studies using disk microneedle rollers in a murine model.
All animal experimental protocols were approved by the Institutional Animal Care and Use Committee of College of Medicine, The Catholic University of Korea, Seoul Korea (PC13DISE0012).
Seven-week-old female C57BL/6 mice were purchased from Lab Animal, Inc. (SLC, Hamamatsu, Japan). The disk microneedle rollers were supplied by DTS™ LAB Co. Ltd. (Seoul, Korea). Rollers were used that contained either no microneedles or microneedles that measured 0.15 mm, 0.25 mm, 0.5 mm, and 1.0 mm in length.
After depilating the dorsal skin of the mice, we conducted a comparative study to examine the effect of microneedle on hair growth. Microneedle stimulation was performed applying the same force and direction to 2×2 cm in the center of the dorsal skin of mice by a single researcher. One cycle was defined as rolling the microneedle from the caudal side to the cranial side and returning to the caudal side. After stimulation, the mouse skin showed mild erythema without swelling or bleeding.
To determine the optimal length and cycle, microneedles of various lengths and cycles were applied five times a week for three weeks to the mice. Microneedles with lengths of 0.15 mm, 0.25 mm, 0.5 mm, and 1.0 mm were applied for 10 cycles, and 0.5 mm length microneedles at 3, 6, 10, 13 cycles were applied to mice (2 mice in each group). Hair growth was measured with visual inspection three weeks after the first microneedle stimulation. The microneedle stimulation that visually showed the most prominent hair growth was considered the optimal length and cycle. Rollers that contained either no microneedles or microneedles with the optimal length and cycle determined in the prior experiment were applied five times a week for three weeks to mice (2 mice in each group). Mice to which rollers were applied that contained no microneedles were used as the control group.
Photographs were taken 13 days and 17 days after the first microneedle stimulation. To investigate the uniformity of hair growth and hair density, 50-fold magnification photographs were taken at the center of hair growth area 7 days and 14 days after the first microneedle stimulation using a phototrichogram system (Folliscope®; LeadM Corporation, Seoul, Korea).
After three weeks, the mice were sacrificed; 1ug of total RNA from mouse skin was prepared using TRIzol reagent (Invitrogen, Carlsbad, CA, USA), and cDNA was synthesized with a QuantiTect Rev Transcription kit (Qiagen, Hilden, Germany) according to the manufacturer's instructions. The primer sequences are listed in Table 1. The real-time polymerase chain reaction (PCR) of the cDNA was performed using SYBR green (Takara, Otsu, Japan). PCR products were quantified using analysis software (CFX96; Bio-Rad, Hercules, CA, USA). The results are normalized relative to the expression of glyceraldehyde 3-phosphate dehydrogenase (GAPDH).
After three weeks, the mice were sacrificed, and skin tissues were dissected, embedded in paraffin and sectioned at 4 µm. Immunohistochemistry (IHC) was performed using a standard protocol. Signals were detected using the 3, 3-diaminobenzidine Reagent Set (DAKO, Glostrup, Denmark) according to the manufacturer's instructions, and counterstaining was carried out with Mayer's hematoxylin (DAKO). The following primary antibodies were used: rabbit anti-vascular endothelial growth factor (VEGF) monoclonal antibody (ABCAM, Cambridge, MA, USA), Wnt3a antibody (ABCAM), Wnt10b antibody (Santa Cruz Biotechnology Inc., Santa Cruz, CA, USA), and β-catenin antibody (Cell Signaling Technology, Beverly, MA, USA).
In the 10-cycle group, visual inspection of the 0.25 mm and 0.5 mm groups 3 weeks after the first microneedle stimulation revealed more prominent hair growth than that in the 0.15 mm and 1.0 mm groups (Fig. 1A). In the 0.5 mm length group, the mice treated with 10 cycles revealed more prominent hair growth 3 weeks after the first microneedle stimulation than did the 3, 6, and 13 cycle groups (Fig. 1B). Therefore, 0.25 mm/10 cycles and 0.5 mm/10 cycles were considered the optimal conditions for microneedle simulation.
Using the optimal length and cycle of the microneedle, visual inspection 13 days and 17 days after the first microneedle stimulation showed more prominent hair growth than that among the controls in both the 0.25 mm/10 cycles and 0.5 mm/10 cycles groups (Fig. 2A). Fifty-fold magnified photography in 7 days and 14 days after the first microneedle stimulation showed uniform hair growth and no visible structural abnormalities (Fig. 2B).
In the 0.25 mm/10 cycles group, β-catenin and VEGF mRNA levels showed no significant differences from the control group, but Wnt3a (22.2-fold) and Wnt10b (7.8-fold) were significantly increased compared with the controls (respectively p<0.01, p<0.05). In the 0.5 mm/10 cycles group, Wnt3a (6.1-fold), β-catenin (1.6-fold), and VEGF mRNA expression (16.0-fold) were significantly increased compared with the control group (p<0.05). Wnt10b mRNA expression (23.2-fold) was more prominently increased than it was in the control group (p<0.01) (Fig. 3). IHC revealed that expression of Wnt3a, β-catenin, VEGF, and Wnt10b all increased in both the 0.25 mm/10 cycles and 0.5 mm/10 cycles groups compared with the control group, especially in the epidermis and HF epithelium. Wnt10b showed marked increased expression in the HF epithelium in the 0.5 mm/10 cycles group compared with the control group (Fig. 4).
In the present study, we show that repeated microneedle stimulation induces hair growth and increases Wnt3a, β-catenin, VEGF, Wnt10b mRNA and protein expression in a murine model. We also demonstrated that among various lengths and cycles of microneedle stimulation, 0.25 mm/10 cycles and 0.5 mm/10 cycles showed the best results. From our results, we suggested that microneedle stimulation itself could induce hair growth via Wnt/β-catenin signaling and VEGF. Activation of Wnt/β-catenin signaling is important not only for initiation and maintenance of hair morphogenesis but also for HF regeneration and growth of the hair shaft1213. Wnt3a and Wnt10b both mediate the canonical Wnt signaling pathway, which induces β-catenin stabilization14. In particular, Wnt10b prominently promoted proliferation and maintained trichogenesis-promoting ability; however Wnt3a had a limited extended effect of hair growth and is known to be mainly involved in HF melanocyte homeostasis15. Expression of VEGF is induced after cutaneous injury, and it mediates angiogenesis and lymphangiogenesis during wound repair16. VEGF is also a major mediator of hair growth and cycling via improving follicle vascularization17. The increased VEGF in our results suggests that wound healing also occurs after microneedle stimulation. Wnt10b/β-catenin and VEGF expression were more prominent in the 0.5 mm/10 cycles group than in the 0.25 mm/10 cycles group, which indicates that the length of the microneedle could be involved in the amount of wound healing mediators. Although the concept that wound healing could induce hair growth is natural, it is unclear which specific signaling pathways are involved. A number of growth factors, prostaglandin, the sonic hedgehog, and Wnt/β-catenin signaling pathways are considered to be involved18. Wound of microneedle is not enough to cause full-thickness wound, and both Wnt/β-catenin signaling and VEGF are involved in anagen induction1317. Therefore effect of hair growth is considered due to modulating hair cycle rather than de novo HF regeneration.
There are several points to consider for human application of microneedle. Length of microneedle is needed to be long enough to penetrate through the skin barrier for enhanced drug delivery, and also short enough to cause minimal skin injury and pain19. Increased collagen and elastin deposition was shown after microneedle on human20. Therefore, when it applied to scalp, perifollicular fibrosis could be evoked, and extensive fibrosis could hinder hair growth. When combined with minoxidil, microneedle could cause overdose of minoxidil which can increase possibility of irritant reaction or cardiovascular side effect. Further studies of not only optimized length and cycle, but also efficacy and safety of microneedle are needed for demonstrating therapeutic effect of microneedle on alopecia.
Our study has a number of limitations. First, the results were confined to a murine model. Second, although all experiments were done by a single researcher and all mice showed only mild erythema without swelling or bleeding after stimulation, microneedle stimulation could be applied at different forces. Despite the limitations, because the precise mechanism of microneedle in terms of hair growth is not well-known except for the drug delivery effect, our study provides basis evidence of the therapeutic effect of microneedle. Additional studies regarding mediators of Wnt/β-catenin signaling pathways and the wound healing process could clarify the exact therapeutic mechanism of microneedle on hair growth.
In conclusion, microneedle stimulation affects hair growth and upregulates the hair growth-related genes in a murine model. Among various lengths and cycles, 0.25 mm/10 cycles and 0.5 mm/10 cycles were the most effective. The Wnt/β-catenin signaling pathways and VEGF were upregulated after microneedle stimulation. Combined with the drug delivery effect of microneedle, we believe that microneedle stimulation could lead to new approaches for alopecia.
Figures and Tables
Fig. 1
Microneedles 0.15 mm, 0.25 mm, 0.5 mm, and 1.0 mm in length were applied in 10 cycles for 3 weeks, and 0.5 mm length microneedles were applied in 3, 6, 10, 13 cycles for 3 weeks. (A) In the 10-cycle group, visual inspection of the 0.25 mm and 0.5 mm groups 3 weeks after the first microneedle stimulation revealed more prominent hair growth than that in the 0.15 mm and 1.0 mm groups. (B) In the 0.5 mm group, 10 cycles revealed the most prominent hair growth 3 weeks after the first microneedle stimulation.
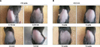
Fig. 2
(A) Using the optimized length and cycle of the microneedle, visual inspection 13 days and 17 days after the first microneedle stimulation showed more prominent hair growth than that in the control group in both the 0.25 mm/10 cycles and 0.5 mm/10 cycles groups. (B) Fifty-fold magnified photographs taken 7 days and 14 days after the first microneedle stimulation showed uniform hair growth and no visible structural abnormalities in both the 0.25 mm/10 cycles and 0.5 mm/10 cycles groups.
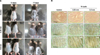
Fig. 3
In the 0.25 mm/10 cycles group, (A) Wnt3a was significantly increased compared with the control group (p<0.01). (B) β-catenin and (C) vascular endothelial growth factor (VEGF) mRNA levels showed no significant changes compared with the controls. (D) Wnt10b was significantly increased compared with the control group (p<0.05). In the 0.5 mm/10 cycles group, (A) Wnt3a, (B) β-catenin, and (C) VEGF mRNA levels were significantly increased compared with the control group (p<0.05). (D) Wnt10b mRNA was more prominently increased than it was in the control group (p<0.01) (Results are normalized relative to glyceraldehyde 3-phosphate dehydrogenase expression; each bar indicates±standard deviation, *p<0.05, **p<0.01). CTL: control group.
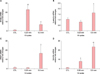
Fig. 4
Immunohistochemistry revealed that expression of Wnt3a, β-catenin, vascular endothelial growth factor (VEGF), and Wnt10b all increased in both the 0.25 mm/10 cycles and 0.5 mm/10 cycles groups compared with the control group, especially in the epidermis and hair follicle epithelium. Wnt10b showed marked increased expression in the hair follicle epithelium in the 0.5 mm/10 cycles group compared with the control group (×200).

Table 1
Primers used for polymerase chain reaction amplications

ACKNOWLEDGMENT
This study was supported by a National Research Foundation of Korea grant funded by the Korean government (2011-0008687).
References
1. Park JH, Choi SO, Seo S, Choy YB, Prausnitz MR. A microneedle roller for transdermal drug delivery. Eur J Pharm Biopharm. 2010; 76:282–289.


3. Park KY, Jang WS, Lim YY, Ahn JH, Lee SJ, Kim CW, et al. Safety evaluation of stamp type digital microneedle devices in hairless mice. Ann Dermatol. 2013; 25:46–53.


4. Kim HM, Lim YY, An JH, Kim MN, Kim BJ. Transdermal drug delivery using disk microneedle rollers in a hairless rat model. Int J Dermatol. 2012; 51:859–863.


5. Millar SE. Molecular mechanisms regulating hair follicle development. J Invest Dermatol. 2002; 118:216–225.


6. Ansell DM, Kloepper JE, Thomason HA, Paus R, Hardman MJ. Exploring the "hair growth-wound healing connection": anagen phase promotes wound re-epithelialization. J Invest Dermatol. 2011; 131:518–528.


7. Langton AK, Herrick SE, Headon DJ. An extended epidermal response heals cutaneous wounds in the absence of a hair follicle stem cell contribution. J Invest Dermatol. 2008; 128:1311–1318.


8. Ito M, Yang Z, Andl T, Cui C, Kim N, Millar SE, et al. Wnt-dependent de novo hair follicle regeneration in adult mouse skin after wounding. Nature. 2007; 447:316–320.


10. Yoo KH, Lee JW, Li K, Kim BJ, Kim MN. Photodynamic therapy with methyl 5-aminolevulinate acid might be ineffective in recalcitrant alopecia totalis regardless of using a microneedle roller to increase skin penetration. Dermatol Surg. 2010; 36:618–622.


11. Lee YB, Eun YS, Lee JH, Cheon MS, Park YG, Cho BK, et al. Effects of topical application of growth factors followed by microneedle therapy in women with female pattern hair loss: a pilot study. J Dermatol. 2013; 40:81–83.


12. Leirós GJ, Attorresi AI, Balañá ME. Hair follicle stem cell differentiation is inhibited through cross-talk between Wnt/β-catenin and androgen signalling in dermal papilla cells from patients with androgenetic alopecia. Br J Dermatol. 2012; 166:1035–1042.


13. Myung PS, Takeo M, Ito M, Atit RP. Epithelial Wnt ligand secretion is required for adult hair follicle growth and regeneration. J Invest Dermatol. 2013; 133:31–41.


14. Ouji Y, Nakamura-Uchiyama F, Yoshikawa M. Canonical Wnts, specifically Wnt-10b, show ability to maintain dermal papilla cells. Biochem Biophys Res Commun. 2013; 438:493–499.


15. Guo H, Yang K, Deng F, Ye J, Xing Y, Li Y, et al. Wnt3a promotes melanin synthesis of mouse hair follicle melanocytes. Biochem Biophys Res Commun. 2012; 420:799–804.


16. Werner S, Grose R. Regulation of wound healing by growth factors and cytokines. Physiol Rev. 2003; 83:835–870.


17. Yano K, Brown LF, Detmar M. Control of hair growth and follicle size by VEGF-mediated angiogenesis. J Clin Invest. 2001; 107:409–417.


18. Hyun MY, Suk JM, Yoo KH, Kim BJ, Kim MN, Hong CK. Unwanted hair growth induced by topical epidermal growth factor during wound healing: true or myth? Int Wound J. 2014; DOI: 10.1111/iwj.12354. [Epub ahead of print].

