Abstract
Obesity-induced disorders contribute to the development of metabolic diseases such as insulin resistance, fatty liver diseases, and type 2 diabetes (T2D). In this study, we evaluated whether the Aloe QDM complex could improve metabolic disorders related to blood glucose levels and insulin resistance. Male C57BL/6 obese mice fed a high-fat diet for 54 days received a supplement of Aloe QDM complex or pioglitazone (PGZ) or metformin (Met) and were compared with unsupplemented controls (high-fat diet; HFD) or mice fed a regular diet (RD). RT-PCR and western blot analysis were used to quantify the expression of obesity-induced inflammation. Dietary Aloe QDM complex lowered body weight, fasting blood glucose, plasma insulin, and leptin levels, and markedly reduced the impairment of glucose tolerance in obese mice. Also, Aloe QDM complex significantly enhanced plasma adiponectin levels and insulin sensitivity via AMPK activity in muscles. At the same time, Aloe QDM decreased the mRNA and protein of PPARγ/LXRα and scavenger receptors in white adipose tissue (WAT). Dietary Aloe QDM complex reduces obesity-induced glucose tolerance not only by suppressing PPARγ/LXRα but also by enhancing AMPK activity in the WAT and muscles, both of which are important peripheral tissues affecting insulin resistance. The Aloe QDM complex could be used as a nutritional intervention against T2D.
Obesity, metabolic syndrome, and associated insulin resistance are major contributors to cardiovascular disease, the leading cause of mortality in the United States (1-4). Insulin resistance is characterized by hyperglycemia, increased lipolysis and free fatty acid levels and increased hepatic triglyceride secretion (5,6). Excessive expansion of white adipose tissue (WAT), the hallmark of obesity, is a major risk factor for type II diabetes (T2D) and cardiovascular diseases (7). Adipose tissue acts as a central regulator of energy metabolism and vascular homeostasis by secreting a diverse range of adipokines, such as adiponectin, leptin, and proinflammatory mediators (8). Inflammation in WAT, characterized by macrophage infiltration and elevated production of adipokines such as leptin and proinflammatory cytokines, plays a key role in linking obesity with insulin resistance and metabolic dysfunction (9,10).
AMP-activated protein kinase (AMPK) is a phylogenetically conserved intracellular energy sensor that plays a central role in the regulation of glucose and lipid metabolism. AMP-activated protein kinase is a heterotrimeric complex composed of a catalytic subunit and two regulatory subunits, and is activated when cellular energy is depleted (11). Recently, it has been found that AMPK plays an important role in inflammation (12) and accelerates ATP-generating catabolic pathways, including glucose and fatty acid oxidation (13,14). In vitro and in vivo studies have demonstrated that the AMPK agonist, 5-aminoimidazole-4-carboxy-amide-1-D-ribofuranoside (AICAR), enhances insulin-mediated glucose transport and insulin action in the muscle and liver of insulin-resistant rats fed a high-fat diet (15,16).
One of the most effective of the currently available medications for T2D is the thiazolidione (TZDs) class of insulin-sensitizing drugs. The TZDs function by binding to the nuclear receptor peroxisome proliferator-activated receptor γ (PPARγ). In WAT, PPARγ activation promotes adipogenesis and the differentiation of new adipocytes. Despite increasing total adipose tissue mass, TZDs have been suggested to improve systemic insulin-sensitive cells and increase the production of adiponectin, a glucose-sensitizing peptide that has anti-inflammatory properties (17).
Metformin (1,1-dimethylbiguanide hydrochloride) is one of the most widely prescribed drugs for the treatment of type 2 diabetes (18). The main molecular target of metformin is AMPK activation. AMPK is a highly conserved heterotrimeric kinase that functions as a metabolic switch, thereby coordinating the cellular enzymes involved in carbohydrate and fat metabolism to enable ATP conservation and synthesis. AMPK is activated by conditions that increase the adenosine monophosphate (AMP): adenosine triphosphate (ATP) ratio, such as exercise and metabolic stress. The effects of stress, exercise, hypoxia and ischemia on AMPK activation have been extensively examined. When the adenosine monophosphate (AMP): adenosine triphosphate (ATP) ratio increases, AMPK is activated by AMPK kinase, and a conformational change is induced when it combines with AMP, thereby decreasing the AMP: ATP ratio by switching off ATP-consuming pathways and switching on ATP-generating pathways (19).
Aloe species have been used for centuries for their laxative, antiinflammatory, immunostimulant, antiseptic (20), burn healing (21), antiulcer (22), and antitumour (23) activities. In the past 15 years, there have also been reports regarding the antidiabetic activity of aloe extracts (24,25).
Our experiments revealed that Aloe QDM complex reduces obesity-induced glucose tolerance and insulin resistance by enhancing AMPK activity in WAT. Hence, Aloe QDM complex may be useful as a dietary adjuvant for reducing obesity-induced metabolic disorders.
Aloe QDM complex (26) was provided by Univera, Inc. (Seoul, Republic of Korea). Pioglitazone (Actos®) was purchased from Eli Lilly (Toronto, Canada) and metformin (Diabex®) was purchased from Daewoong Pharm. Co., Ltd. (Seoul, Republic of Korea); chromium (Cr) was purchased from Lallemand, Inc. (Montreal, Canada); and leupeptin, aprotinin, and phenylmethylsulfonyl fluoride (PMSF) were purchased from Sigma Chemical Co. (St. Louis, MO, USA). Anti-mouse AMP-activated protein kinase (AMPK) and phospho-AMPK (p-AMPK) were purchased from Cell Signaling (Cell Signaling Technology, Beverly, MA, USA), and all other chemicals and reagents used in this study were reagent grade.
Male C57BL/6NCrjBgi mice were purchased from the Charles River Laboratory of Animal Science (Orient Co., Seoul, Republic of Korea) at four weeks old and fed a normal diet for one week. Animals were housed in individual cages with free access to water and food in a temperature-controlled animal facility under a 12 h light-dark cycle at 22±2℃ and 55±5% humidity. Mice were fed either a high-fat diet (HFD) (Open Source diets #D12492; Research Diets Inc., New Brunswick, NJ) to induce obesity, or a regular diet (RD; Open Source diets #D12450B; Research Diets Inc.). The nutritional contents of the HFD were similar to those of the regular diet except for the low carbohydrate content and high level of fat.
At 26 weeks of age, mice exhibiting blood glucose levels >160 mg/dl were selected as non-insulin-dependent diabetes mellitus (NIDDM) animals and were divided into five groups of 20 animals per group. One group was treated with PBS only and served as diabetic controls; one group received daily 100 mg/kg of PAG (Processed Aloe vera gel) containing 2% ALS (Aloe QDM) plus 500 mg/kg of Cr-enriched yeast containing 0.2% Cr (Aloe QDM complex), the other group was administered pioglitazone (PGZ, 2.5 mg/kg), and the fifth group was administered metformin (Met, 250 mg/kg), an anti-diabetic drug currently in clinical use. Mice were weighed and blood samples were collected weekly by tail bleeding into heparin-coated tubes after 4-h fasts.
At the end of the experimental period, mice were sacrificed and blood samples were taken from the inferior vena cava to determine plasma insulin. After collecting blood, the liver, thymus, pancreas, kidney, lung, heart, and spleen were removed, rinsed with physiological saline solution, and immediately stored at -70℃. White adipose tissues were immediately removed from periepididymal and perirenal fat for morphological examination. Mice were treated in accordance with the guidelines issued by Sahmyook University for the care and use of laboratory animals.
Blood glucose concentrations were monitored after 4-hour fasts from venous blood from the tail vein using a glucometer (MediSence Optimum, Abbott Laboratories, Bedford, MA, USA) at 7, 14, 24, 34, 44, and 54 days of age, equivalent to days of the supplementation period.
Blood was collected via cardiac puncture, allowed to clot for 30 minutes, and spun at 7,000 rpm for 10 minutes. Isolated serum was stored at -80℃. Plasma insulin, plasma adiponectin, and plasma leptin levels were assayed using enzyme-linked immunosorbent assay (ELISA) kits (Shibayagi's Insulin Assay Kit, Shibayagi Co., Gunma, Japan, and Millipore, Bedford, MA, USA).
Intraperitoneal glucose tolerance testing was performed during the last week of the experimental period. Mice that had fasted overnight received an intraperitoneal injection of glucose (1.5 g of glucose/kg of body weight), and blood samples in Aloe QDM complex-treated groups were obtained for glucose measurement at 0, 30, 60, 90, 120, and 150 minutes. Blood glucose was measured using a glucometer (MediSence Optimum, Abbott Laboratories, Bedford, USA) for blood collected from the tail vein after glucose administration.
Intraperitoneal insulin tolerance testing was performed during the last week of the experimental period. Mice that had fasted overnight received an intraperitoneal injection of insulin (0.75 U of insulin/kg of body weight), and blood samples in Aloe QDM complex-treated groups were obtained for glucose measurement at 0, 30, 60, 90, 120, and 150 minutes. Blood glucose was measured using a glucometer (MediSence Optimum, Abbott Laboratories, Bedford, USA) on blood collected from the tail vein after glucose administration.
Total RNA was extracted from frozen tissues using the RNeasy Mini kit (QIAGEN, Valencia, USA) in an RNase-free environment. RNA was then quantified based on the absorbance at 260 nm. The reverse transcription of 1µg of RNA was carried out using M-MLV reverse transcriptase (Promega, USA), an oligo (dT) 16 primer, dNTP (0.5µM), and 1 U RNase inhibitor. After incubation at 65℃ for 5 min and 37℃ for 60 min, M-MLV reverse transcriptase was inactivated by heating at 70℃ for 15 min. The polymerase chain reaction (PCR) was performed in 50 mM KCl, 10 mM Tris-HCl (pH 8.3), 1.5 mM MgCl2, and 2.5 mM dNTPs with 5 units of Taq DNA polymerase and 10 pM of each primer set for peroxisome proliferator-activated receptor gamma (PPARγ), liver X receptor alpha (LXRα), CD36 and scavenger receptor A (SR-A). The cDNA was amplified by 35 cycles of denaturing at 94℃ for 45 s, annealing at 62℃ for 45 s, and extension at 72℃ for 1 min. The final extension was performed at 72℃ for 5 min. The PCR products were then electrophoresed on 1.5% agarose gels and stained with ethidium bromide. The primers selected were 5' GAG CCT GTG AGA CCA ACA GC 3' (forward) and 5' GAT TCC GAA GTT GGT GGG CC 3' (reverse) for PPARγ, 5' AGG GTT GGA GTC AGC AGA GC 3' (forward) and 5' GGA AGA ATC CCT TGC AGC CC 3' (reverse) for LXRα, 5' AGT AGG CGT GGG TCT GAA GG 3' (forward) and 5' CTT GCT TGC CCA GTC ACA GG 3' (reverse) for CD36, 5' GGA GAC AGA GGG CTT ACT GG 3' (forward) and 5' GTT GAT CCG CCT ACA CTC CC 3' (reverse) for SR-A, and 5' CAA CTT TGG CAT TGT GGA AGG 3' (forward) and 5' ATG GAA ATT GTG AGG GAG ATG C 3' (reverse) for GAPDH, which was used as an internal control.
Frozen muscles were homogenized in 3 volumes of ice-cold lysis buffer [20 mM Trizma base, 50 mM NaCl, 250 mM sucrose, 50 mM NaF, 5 mM Na4P2O7 · 10H2O, 1% Triton-X100, 5µg/ml leupeptin, 1 mM phenylmethylsulfonyl fluoride (PMSF), and 5µg/ml aprotinin]. Twenty micrograms of protein from cell lysates were applied to 8~12% SDS-polyacrylamide gels and then transferred to nitrocellulose membranes. The membranes were blocked with 5% skim milk in TBST solution for 1 hr. They were then incubated with anti-AMPK and anti-p-AMPK monoclonal antibodies for 2 hrs and washed 3 times with TBST. After incubation with alkaline phosphatase-labeled secondary antibody for 2 hrs, the bands were visualized using a Western Blot Kit with an alkaline phosphatase substrate (Vector, Burlingame, USA).
The obese mice supplemented with Aloe QDM complex had a lower body weight than that of obese mice on a HFD (Fig. 1A). The effects of the Aloe QDM complex, pioglitazone (PGZ), and metformin (Met) supplementation on blood glucose levels are shown in Fig. 1B. The HFD-fed mice exhibited a significant increase in blood glucose concentrations compared with RD-fed mice. On the other hand, Aloe QDM complex-treated mice significantly decreased fasting blood glucose levels, similar to Met. Further, the fasting plasma insulin levels of HFD-fed mice were significantly (3.5-fold) higher than those of regular diet-fed mice. And, treatment of HFD-fed mice with Aloe QDM complex for 8 weeks significantly reduced plasma insulin levels (Fig. 2A).
To test whether Aloe QDM complex reduced obesity-induced insulin resistance, we compared the degree of glucose or insulin intolerance in the mice. At the near end of the 8-week experimental period (3 days before sacrifice), glucose tolerance was examined using the IPGTT method. As shown in Fig. 2B, glucose levels following the i.p. injection were significantly higher than those in RD-fed mice. Aloe QDM complex treatment reduced blood glucose levels at 60 min and 90 min. Insulin tolerance tests revealed that the obese mice were insulin intolerant, and as shown in Fig. 2C, those supplemented with dietary Aloe QDM complex had significantly lower levels of plasma glucose than control obese mice at 30 min and 60 min after oral glucose infusion and the levels tended to remain lower. These findings indicated that Aloe QDM complex protected against obesity-induced diabetes.
The nuclear receptor, PPARγ, is endogenously activated by some polyunsaturated fatty acids and products of lipid metabolism. PPARγ activation has been shown to significantly attenuate adipocyte hypertrophy and inhibit WAT inflammation, whereas it increases the total WAT mass. We examined whether the aloe formula could affect mRNA expression of the nuclear receptor PPARγ/LXRα in WAT. PPARγ and LXRα mRNA expression of Aloe QDM complex treatment group was lower in WAT than in the HFD group (Fig. 3A).
CD36 and SR-A are scavenger receptors for oxidized low-density lipoprotein (oxLDL) and cellular transporters of long-chain fatty acid (27). In the present study, we examined changes in the level of expression of scavenger receptors and their effect on the amount of adipose tissue in obese mice. We found that WAT and levels of scavenger receptors such as SR-A and CD36 in the WAT were significantly lower in obese mice supplemented with dietary aloe formula than in the HFD group (Fig. 3B).
This was noteworthy, and it also confirmed that the aloe formula could be adopted as a PPAR antagonist for inhibition of scavenger receptors in ATM (adipose tissue macrophage).
To further define the possible underlying mechanisms, we measured the expression of adiponectin and leptin and AMPK activity. As shown in Fig. 4, gene expressions of adiponectin and leptin were significantly increased by aloe formula as with PGZ or Met. Circulating adiponectin was significantly reduced by HFD feeding when compared with normal chow fed, whereas leptin production was increased. Aloe QDM complex increased production of adiponectin while circulating leptin was reduced as with PGZ and Met.
Next, we measured the AMPK expression in the muscles. Since the AMPK cascade has been shown to activate insulin signaling (16), we hypothesized that AMPK might be involved in the effect cellular Energy Metabolism on tissues. As shown in Fig. 5, the ratio of phospho-AMPKα to AMPKα was significantly higher in the Aloe QDM complex-fed mice than in the HFD-fed mice, indicating that the dietary aloe formula enhanced energy metabolism on WAT.
This study demonstrated that Aloe QDM complex can reduce obesity-induced insulin resistance. Herbal prescriptions have been recognized as potentially valid by the scientific medical establishment, and their use has been increasing. Since traditional herbal prescriptions are generally prepared from a combination of crude drugs, on the basis of oriental prescriptions and herbology, they may exert combined effects that differ from the sum of the effects of the individual constituents (27).
Dietary aloe formula has been demonstrated to affect inflammation via immunosuppression. Recent studies have suggested that inflamed adipocytes in the obese trigger the development of obesity-related metabolic disorders such as insulin resistance and T2D, indicating that a reduction of tissue inflammation may be beneficial in obesity-related metabolic diseases. Our previous in vivo study demonstrated a potential effect of PAG, an aloe formula, on hypoglycemia and hypolipidemia (26,28). In particular, our results demonstrated that the administration of aloe formulas including PAG, ALS, Aloe QDM, and Aloe QDM complexes to these mice prevented the development of T2D-related symptoms. However, the prevention of or therapeutic effect on obesity-induced metabolic disorders has never been fully established.
The administration of Aloe QDM complex to HFD-fed mice reduced body weight and blood glucose concentration to normal levels despite being continued throughout the 8-week treatment period (Fig. 1). The current understanding of disease progression in T2D is that insulin resistance in peripheral tissues leads to compensatory hyperinsulinemia followed by β-cell failure (29). In the present study, Aloe QDM complex significantly improved insulin resistance (Fig. 2). These results showed that Aloe QDM complex increased insulin sensitivity by decreasing blood glucose and insulin levels. Improved blood glucose homeostasis was also observed in the IPGTT in Aloe QDM complex-supplemented HFD-fed mice (Fig. 2). High-fat intake and increased levels of free fatty acids in the circulation lead to insulin resistance. Oral administration of aloe formula reduced plasma leptin whereas adiponectin was significantly induced (Fig. 4) by Aloe QDM complex. We also examined the mRNA expression of adipogenic genes in WAT by semiquantitive RT-PCR to determine whether Aloe QDM complex reduced lipogenesis. Aloe QDM complex suppressed the expressions of the adipogenesis genes, PPARγ/LXRα (Fig. 3A), suggesting that the aloe formula may be able to improve insulin resistance through a reduction of fat in the WAT. Also, scavenger receptors, specifically SR-A and CD36, play crucial roles in the pathogenesis of atherosclerotic lesions by identifying and facilitating the uptake of oxLDL (30). In phagocytes, CD36 primarily functions as a scavenger receptor, recognizing specific self and nonself molecular patterns and triggering internalization and inflammatory signaling pathways to eliminate pathogens and altered self components, such as apoptotic cells (31,32). CD36 cooperates with toll-like receptor (TLR)-4 and -6 to mediate the sterile inflammatory response to altered self components oxLDL (33) and also acts as a coreceptor with TLR2 and -6 in the recognition of microbial diacylglycerides (34,35).
Despite the importance of adipose tissue-resident macrophages in the pathogenesis of insulin resistance, this is the first study to our knowledge that measures the expression of scavenger receptor genes in diet-induced obesity (DIO) mice supplemented with Aloe QDM complex. Our data demonstrated that the Aloe QDM complex decreased gene expression of scavenger receptors in WAT of obese mice (Fig. 3B) in WAT, leading to reduced atherosclerotic lesions in obese mice.
The most important finding in this study was that Aloe QDM complex reduced body fat via activation of AMPK in the muscle (Fig. 5). These results reveal that the Aloe QDM complex increased mitochondrial biogenesis in both WAT and muscles by activating AMPK and further reduced body fat by down-regulating the expression of scavenger receptors in the WAT.
In almost all of the data, Aloe QDM complex significantly improved insulin resistance and suppressed adipogenesis genes in WAT by activating AMPK, suggesting that Aloe QDM complex is a useful dietary phytochemical for improving obesity-related metabolic disorders such as insulin resistance.
Figures and Tables
Figure 1
Effects of Aloe QDM complex on body weight and blood glucose change. C57BL/6 mice were fed a high-fat diet supplemented with an aloe formula. (A) Weekly changes in body weight of Aloe QDM complex or anti-diabetic drug-supplemented mice. (B) Blood glucose levels in plasma. Data are means±SEM.values. ††p<0.01 compared with RD-fed mice. *p<0.05, **p<0.01 compared with untreated HFD-fed mice.
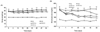
Figure 2
Effects of Aloe QDM complex on insulin homeostasis. (A) Change of plasma insulin levels. At the end of the 8-week experimental period, blood samples were taken from the inferior vena cava, and plasma insulin concentration was measured using commercial insulin ELISA kits. (B) Glucose tolerance and (C) insulin tolerance were improved by Aloe QDM complex. Three days before sacrifice, mice were fasted overnight and then injected intraperitoneally with glucose (1.5 g/kg body weight) or insulin (0.75 U/kg body weight), respectively. Blood glucose levels were measured using tail blood samples at the indicated times post-injection. Data are means±SEM.values. ††p<0.01 compared with RD-fed mice. **p<0.01 compared with untreated HFD-fed mice.
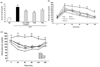
Figure 3
Effects of Aloe QDM complex on adipogenesis. WAT were isolated from HFD-fed mice. mRNA expression of (A) PPARγ and LXRα and (B) SR-A and CD36 in the WAT were measured by RT-PCR. Experiments were performed in triplicate with similar results.
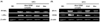
Figure 4
Effects of Aloe QDM complex on adiponectin and leptin. WAT were isolated from HFD-fed mice. (A) mRNA expression of adiponectin and leptin in the WAT were measured by RT-PCR. Secretion of (B) adiponectin and (C) leptin protein in the WAT was measured by ELISA. Experiments were repeated in triplicate with similar results. Data are means±SEM.values. ††p<0.01 compared with RD-fed mice. **p<0.01 compared with untreated HFD-fed mice.
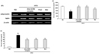
ACKNOWLEDGMENTS
This work was supported by Univera, Inc. as one of CAP projects and also by the Sahmyook University Research fund in 2011.
References
1. Weiser M, Frishman WH, Michaelson MD, Abdeen MA. The pharmacologic approach to the treatment of obesity. J Clin Pharmacol. 1997. 37:453–473.


2. Surwit RS, Kuhn CM, Cochrane C, McCubbin JA, Feinglos MN. Diet-induced type II diabetes in C57BL/6J mice. Diabetes. 1988. 37:1163–1167.


4. Nicolai A, Li M, Kim DH, Peterson SJ, Vanella L, Positano V, Gastaldelli A, Rezzani R, Rodella LF, Drummond G, Kusmic C, L'Abbate A, Kappas A, Abraham NG. Heme oxygenase-1 induction remodels adipose tissue and improves insulin sensitivity in obesity-induced diabetic rats. Hypertension. 2009. 53:508–515.


5. Peterson SJ, Kim DH, Li M, Positano V, Vanella L, Rodella LF, Piccolomini F, Puri N, Gastaldelli A, Kusmic C, L'Abbate A, Abraham NG. The L-4F mimetic peptide prevents insulin resistance through increased levels of HO-1, pAMPK, and pAKT in obese mice. J Lipid Res. 2009. 50:1293–1304.


6. Porstmann T, Santos CR, Griffiths B, Cully M, Wu M, Leevers S, Griffiths JR, Chung YL, Schulze A. SREBP activity is regulated by mTORC1 and contributes to Akt-dependent cell growth. Cell Metab. 2008. 8:224–236.


7. Wellen KE, Hotamisligil GS. Inflammation, stress, and diabetes. J Clin Invest. 2005. 115:1111–1119.


8. Rajala MW, Scherer PE. Minireview: The adipocyte--at the crossroads of energy homeostasis, inflammation, and atherosclerosis. Endocrinology. 2003. 144:3765–3773.


9. Weisberg SP, McCann D, Desai M, Rosenbaum M, Leibel RL, Ferrante AW Jr. Obesity is associated with macrophage accumulation in adipose tissue. J Clin Invest. 2003. 112:1796–1808.


10. Xu H, Barnes GT, Yang Q, Tan G, Yang D, Chou CJ, Sole J, Nichols A, Ross JS, Tartaglia LA, Chen H. Chronic inflammation in fat plays a crucial role in the development of obesity-related insulin resistance. J Clin Invest. 2003. 112:1821–1830.


11. Hardie DG, Carling D. The AMP-activated protein kinase--fuel gauge of the mammalian cell? Eur J Biochem. 1997. 246:259–273.


12. Sag D, Carling D, Stout RD, Suttles J. Adenosine 5'-monophosphate-activated protein kinase promotes macrophage polarization to an anti-inflammatory functional phenotype. J Immunol. 2008. 181:8633–8641.


13. Makinde AO, Gamble J, Lopaschuk GD. Upregulation of 5'-AMP-activated protein kinase is responsible for the increase in myocardial fatty acid oxidation rates following birth in the newborn rabbit. Circ Res. 1997. 80:482–489.


14. Zong H, Ren JM, Young LH, Pypaert M, Mu J, Birnbaum MJ, Shulman GI. AMP kinase is required for mitochondrial biogenesis in skeletal muscle in response to chronic energy deprivation. Proc Natl Acad Sci U S A. 2002. 99:15983–15987.


15. Ju JS, Gitcho MA, Casmaer CA, Patil PB, Han DG, Spencer SA, Fisher JS. Potentiation of insulin-stimulated glucose transport by the AMP-activated protein kinase. Am J Physiol Cell Physiol. 2007. 292:C564–C572.


16. Iglesias MA, Ye JM, Frangioudakis G, Saha AK, Tomas E, Ruderman NB, Cooney GJ, Kraegen EW. AICAR administration causes an apparent enhancement of muscle and liver insulin action in insulin-resistant high-fat-fed rats. Diabetes. 2002. 51:2886–2894.


17. Josep BR, Amir G, Jennifer K, Raquel H. Peroxisome proliferator activated receptors: the nutritionally controlled molecular networks that integrate inflammation, immunity and metabolism. Current Nutrition & Food Science. 2005. 1:179–187.
18. Hardie DG. AMP-activated protein kinase as a drug target. Annu Rev Pharmacol Toxicol. 2007. 47:185–210.


19. Hardie DG, Carling D, Carlson M. The AMP-activated/SNF1 protein kinase subfamily: metabolic sensors of the eukaryotic cell? Annu Rev Biochem. 1998. 67:821–855.


20. Capasso F, Borrelli F, Capasso R, Di Carlo G, Izzo A, Pinto L, Mascolo N, Castaldo S, Longo R. Aloe and its therapeutic use. Phytother Res. 1998. 12:S124–S127.


21. Heggers JP, Kucukcelebi A, Stabenau CJ, Ko F, Broemeling LD, Robson MC, Winters WD. Wound healing effects of Aloe gel and other topical antibacterial agents on rat skin. Phytother Res. 1995. 9:455–457.


23. Winters WD, Benavides R, Clouse WJ. Effects of aloe extracts on human normal and tumor cells in vitro. Econ Bot. 1981. 35:89–95.


24. Yongchaiyudha S, Rungpitarangsi V, Bunyapraphatsara N, Chokechaijaroenporn O. Antidiabetic activity of Aloe vera L. juice. I. Clinical trial in new cases of diabetes mellitus. Phytomedicine. 1996. 3:241–243.


25. Bunyapraphatsara N, Yongchaiyudha S, Rungpitarangsi V, Chokechaijaroenporn O. Antidiabetic activity of Aloe vera L. juice: II. Clinical trial in diabetes mellitus patients in combination with glibenclamide. Phytomedicine. 1996. 3:245–248.


26. Kong H, Lee S, Shin S, Kwon J, Jo TH, Shin E, Shim KS, Park YI, Lee CK, Kim K. Down-regulation of adipogenesis and hyperglycemia in diet-induced obesity mouse model by Aloe QDM. Biomolecules & Therapeutics. 2010. 18:336–342.


27. Kim JO, Kim KS, Lee GD, Kwon JH. Antihyperglycemic and antioxidative effects of new herbal formula in streptozotocin-induced diabetic rats. J Med Food. 2009. 12:728–735.


28. Kim K, Kim H, Kwon J, Lee S, Kong H, Im SA, Lee YH, Lee YR, Oh ST, Jo TH, Park YI, Lee CK, Kim K. Hypoglycemic and hypolipidemic effects of processed Aloe vera gel in a mouse model of non-insulin-dependent diabetes mellitus. Phytomedicine. 2009. 16:856–863.


29. Martín-Fuentes P, Civeira F, Recalde D, García-Otín AL, Jarauta E, Marzo I, Cenarro A. Individual variation of scavenger receptor expression in human macrophages with oxidized low-density lipoprotein is associated with a differential inflammatory response. J Immunol. 2007. 179:3242–3248.


30. Stewart CR, Stuart LM, Wilkinson K, van Gils JM, Deng J, Halle A, Rayner KJ, Boyer L, Zhong R, Frazier WA, Lacy-Hulbert A, El Khoury J, Golenbock DT, Moore KJ. CD36 ligands promote sterile inflammation through assembly of a Toll-like receptor 4 and 6 heterodimer. Nat Immunol. 2010. 11:155–161.


31. Sahoo D, Drover V. The role of scavenger receptors in signaling, inflammation and atherosclerosis. Biochemistry of Atherosclerosis. 2006. 1:70–91.


32. Peiser L, Gordon S. The function of scavenger receptors expressed by macrophages and their role in the regulation of inflammation. Microbes Infect. 2001. 3:149–159.


33. Stewart CR, Stuart LM, Wilkinson K, van Gils JM, Deng J, Halle A, Rayner KJ, Boyer L, Zhong R, Frazier WA, Lacy-Hulbert A, El Khoury J, Golenbock DT, Moore KJ. CD36 ligands promote sterile inflammation through assembly of a Toll-like receptor 4 and 6 heterodimer. Nat Immunol. 2010. 11:155–161.

