Abstract
Purpose
Materials and Methods
Results
Figures and Tables
Fig. 1
(A) Schematic diagram of GNP-HER2 with tumor marker attached to gold nanoparticles (NPs). (B) Comparison of expression levels of HER2 protein by Western blot analysis. The expression level of melanoma cells (G361) was significantly higher than in keratinocytes (HaCaT). GNP-HER2, gold nanoparticles combined with the antibody against HER2.
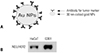
Fig. 2
The effects of GNP-HER2 on the proliferation of G361 cells (A), HaCaT cells (B), and HER2 antibody-only cell lines (C). Cells were incubated with GNP-HER2 for 24, 48, and 72 hours and then analyzed by the WST-1 method. GNP-HER2, gold nanoparticles combined with the antibody against HER2.
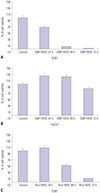
Fig. 3
Apoptosis induced by GNP-HER2. G361 was treated with GNP-HER2 and Hemacolor staining after 24-hour incubation. (A) G361 cells treated with GNP-HER2 (lower) and untreated cells (upper). (B) Cells treated with GNP-HER2 (right) and apoptotic bodies and untreated cells (left) after Hoechst staining (×1000). GNP-HER2, gold nanoparticles combined with the antibody against HER2.
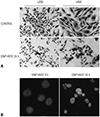
Fig. 4
Photomicrograph of GNP-HER2 for 24 h (lower end) and non-use (upper end). Immunofluorescent staining followed by confocal microscopy (×800). (A) Localization changes of intracellular AIF were observed through immunocytochemistry using anti-AIF-antibodies. (B) Changes in the localization of cytochrome c due to GNP-HER2 treatment for 24 hours were observed by immunocytochemistry using anti-cytochrome c antibody. GNP-HER2, gold nanoparticles combined with the antibody against HER2; AIF, apoptosis inducing factor.
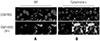
Fig. 5
The activity of GNP-HER2-induced apoptosis-related proteins in G361 cells was observed by Western blotting (A–C). G361 cells were incubated with GNP-HER2 at the scheduled time. Total protein lysates were Bax/Bcl-2 (A), caspase-3/caspase-9N (B), and PARP-1/DFF45 (C) antibodies. Glyceraldehyde-3-phosphate dehydrogenase was used for protein quantification. GNP-HER2, gold nanoparticles combined with the antibody against HER2.
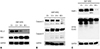
Fig. 6
G361 cells treated with GNP-HER2 induce apoptosis. G361 cells were treated with GNP-HER2 for 12 to 48 hours. At each time point, fluorescence-activated cell sorting analysis was performed using Pl. GNP-HER2, gold nanoparticles combined with the antibody against HER2.
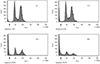
Fig. 7
Western blot analysis showing that GNP-HER2 effectively reduced protein expression for cell cycle arrest. When G361 cells and GNP-HER2 were co-cultured, expression of p21; cyclin D, E, and A; and the cyclin partners cdk2, cdk4, and cdc2 was observed. GNP-HER2, gold nanoparticles combined with the antibody against HER2.
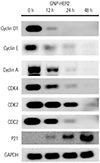
Fig. 8
GNP-HER2 treatment significantly decreased focal adhesion proteins in the cells over time. G361 cells were incubated for the indicated time with GNP-HER2, and then, Western blot tests were performed using p-paxillin, p-FAK, p-HER2, FAK, and HER2 antibodies. (A) GNP-HER2 severely induces the disintegration of filamentous actin. (B) Control group was not treated with GNP-HER2 (left). F-actin staining of G361 cells was treated with GNP-HER2 for 24 h (right). GNP-HER2, gold nanoparticles combined with the antibody against HER2; FAK, focal adhesion kinase.
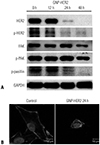
ACKNOWLEDGEMENTS
Notes
AUTHOR CONTRIBUTIONS
Conceptualization: Uk Kyu Kim.
Formal analysis: Gyoo Cheon Kim.
Funding acquisition: Gyoo Cheon Kim.
Investigation: Hyeon Jun Jeon.
Methodology: Hyeon Jun Jeon.
Resources: Byul Bo Ra Choi, Dae Seok Hwang.
Supervision: Uk Kyu Kim.
Validation: Byul Bo Ra Choi.
Visualization: Kwang Ha Park, Dae Seok Hwang.
Writing—original draft: Hyeon Jun Jeon, Gyoo Cheon Kim.
Writing—review & editing: Hyeon Jun Jeon, Gyoo Cheon Kim.
References























