Abstract
Prostate cancer is one of the high incidences and the most invasive cancer that is also highly resistant to chemotherapy. Currently, several natural products have been considering using as the supplements for anti-cancer therapy. This study aims to identify the potential active anti-cancer ingredients in the bran extracts of the native Thai rice (Luempua cultivar). Rice bran fraction enriched in anthocyanins was successively isolated and processed until the major purified compound obtained. The sub-fractions and the purified, rice bran, cyanidin 3-glucoside (RBC3G), were studied for biological effects (cell viability, migration, and invasion assays) on human prostatic cancer (PC3) cells using immunohistochemical-staining and immuno-blotting approaches. The sub-fractions and the purified RBC3G inhibited epithelial mesenchymal transition (EMT) characteristics of PC3 cells by blocking the expression of several cytoskeletal associate proteins in a concentration dependent manner, leading to decreasing of the cancer cell motility. RBC3G reduced the expression of Smad/Snail signaling molecules but enhanced the expression of cell surface protein, E-cadherin, leading to a delay tumor transformation. The RBC3G also inhibited matrix metalloproteinase-9 and nuclear factor-kappa B expression levels and the enzymes activity in PC3 cells, leading to a slow cell migration/invasion process. The results suggested that RBC3G blunt and/or delay the progressive cancer cell behaviors by inhibit EMT through Smad signaling pathway(s) mediating Snail/E-cadherin expression.
Black rice and rice bran have beneficial health effects in human due to its anthocyanins, a phenolic compound in the flavonoid family, and phytosterol compositions [1-4]. Anthocyanins derived from the black rice recognized for their antioxidant properties that can play a significant role in cancer prevention [5]. Anthocyanins inhibit many human cancer cells included tongue carcinoma (SCC-4), hepatocellular carcinoma (Huh-7), and cervical carcinoma (HeLa) through the induction of apoptosis, anti-angiogenesis and anti-invasiveness [6]. The anti-invasion effects of anthocyanins are correlated with the repression of proteolytic enzymes; matrix metalloproteinase-2 (MMP-2), matrix metalloproteinase-9 (MMP-9) or urokinase-plasminogen activator (u-PA) [7] that the transformed cells secret to assist their invasive and migratory capabilities. Although not derived from rice, anthocyanin- and proanthocyanidin-enriched fractions from blueberry (Vaccinium angustifolium) similarly down-regulate MMP-2/MMP-9 in prostate cancer cells [1]. In addition, some studies have demonstrated that crude extract of anthocyanins can inhibit human lung cancer cells invasion via alteration of epithelial mesenchymal transition (EMT) by decreasing Akt phosphorylation and suppression of vimentin, N-cadherin, and Snail1/2 [2]. The aim of this study is to search for the major constituent in the Thai’s rice bran anthocyanins and investigated for its underlining mechanism(s) to inhibit cancer metastatic and invasive ability using an aggressive prostate cancer cell line, PC3 cell, as a model.
Prostate cancer is one of the most common solid organ cancers that cause a high mortality rate worldwide. The cancer forms and develops slowly in the adult males under the influencing of genetic and environmental factor. Once developed, the cancer becomes one of the most metastatic cancers that has no specific treatment and often resistant to chemotherapy and radiotherapy [8]. Previously, research studies have purposed the alternative treatment by the usage of several plant extracts and plant-derived bioactive compounds that shown the in vitro and in vivo anti-cancer properties via different modes of action. For example, the crude extracts containing polysaccharides significantly inhibited growth and proliferation of the high-metastatic, androgen-insenitive prostate cancer cells (PC3 and DU145 cells) [9-11], and suppressed migration of DU145 cell [12], whereas it induced the low-metastatic, androgen-senitive prostate cancer LNCaP cell apoptosis [13]. The proliferation inhibition effect from the polyphenol contents of the extract is stronger in the cancer cells (PC3, DU145, and LNCaP cell) comparing to the normal prostate epitelial cells (hPrEC) [14]. In addition, most research investigations reported the correlative and variable mechanisms of action depending on the specific chemical-derivatives of the compounds and the study methods being applied [15]. The aglycone derivative of aromatic phenolic compounds such as anthocyanidins, apigenin and delphinidin had showed inhibitory effect on cells proliferation, migration and invasion in aggressive PC3 and DU145 cells [16]. Cyanidin-3-O-β-glucopyranoside effectively induced apoptosis via caspase-3 activation and increased p21 protein expression in DU145 and LNCaP cells, and also increased P75NGFR tumor suppressor protein that regulate cell differentiation in DU145 [17]. However, studying on the synthetic aromatic compounds and sugar-aromatic molecule conjugates glucosides-derivative containing a monosaccharide and disaccharide treatments in PC3 cells showed their strength of anti-proliferation and anti-metastatic potential comparing to the natural isolated compounds suggesting that the presentation of sugar moiety/polarity is important for the pure compound activities [18, 19]. The study in other aromatic compound, benzofuran and benzodifuran exert the antiproliferative properties on PC3 cell line. Benzofuran derivative show the ability to bind telomeric DNA and inhibited cell cycle progression when compare with benzodifuran [20]. Therefore, advance knowledge with the conclusive results acquired before promoting the medical contribution of compound constituents from plant extracts to cancer prevention and anti-cancer therapy.
Accumulative data revealed that at the initial stage of cancer development, the abnormal prostate cells switch phenotype from the polarized epithelial cells to the mesenchymal phenotype. This EMT is facilitated by the loss of cell adhesion molecule, E-cadherin, and the translocation of β-catenin linkage protein from the cytoplasm into the nucleus [21, 22]. EMT characteristic is allowed for cell movement, and E-cadherin loss appears to be an important step in the invasion and metastasis of cancers. Significant down-regulation of E-cadherin expression during EMT process allows this molecule to be used as a molecular marker of EMT [23]. EMT is also involved in progressive redistribution of mesenchymal molecules, such as vimentin and N-cadherin [23-25]. The over-expression of vimentin occurs during EMT allowing this molecule to be used as a molecular marker of EMT. Previously, studies in the in vitro models demonstrate that E-cadherin expression is extensively reduced in human prostate cancer cell line [25], and β-catenin accumulation in the nucleus is correlated with invasive phenotype of most cancers [26, 27]. The key factors regulating EMT process are growth factors, such as Transforming Growth Factor-β (TGF-β) and Epidermal Growth Factor (EGF), located in the extracellular matrix nearby the tumor mass. Growth factors mediate WNT and Notch signaling pathways by activation of transcription factors such as Smad (Sma and Mad proteins from Caenorhabditis elegans and Drosophila), Snail, ZEB, and Twist [28, 29]. After activation, Smad is phosphorylated and formed a complex that moves into the nucleus to regulate the transcription of many genes including Snail [30, 31]. Snail, is a prominent inducer of EMT that strongly represses the expression of E-cadherin [32]. Snail is also involved in the acquisition of resistance to apoptosis and in promoting tumor survival [23, 29], thereby it is likely potentiating invasion and metastasis of cancer cells. Attempts in this study were to amplify the mechanisms of the specific compound from the rice bran that inhibited metastatic signals underlining the EMT of prostate cancer cell using the lived-cells relative proteins expression analysis.
The dark purple glutinous rice (Oryza sativa L.) cultivar Luem Pua (LP) used in this study collected from experimental field in Phitsanulok Rice Research Center, Phitsanulok province, Thailand in October 2015. One kilogram of the bran of LP rice was successively macerated in n-hexane, followed by methanol at room temperature (2×1,000 ml for each solvent) (Fig. 1A). The maceration period for each extraction was 7 days. After filtration, the combined extracts were evaporated to dryness under reduced pressure at temperature about 40°C to give the hexane extract (LBH) and the methanol extract (LBM). Forty grams of the LBM was further isolated and purification. The extract was fraction by column chromatography (CC) over silica gel (Merck cat. No. 9385, 40–63 μm, 250 g) and eluted under gradient condition: n-hexane, n-hexane-ethyl acetate, ethyl acetate-methanol and methanol with increasing amount of the more polar solvent, to afford three sub-fractions LBM1, LBM2, and LBM3. LPBM2 (3.0 g) was separated into three sub-fractions including LBM2.1, LBM2.2, LBM2.3 on a Sephadex LH-20 and methanol was used as eluent. LBM2.2 (200 mg) was fractionated by CC over Sephadex LH-20 and eluted under isocratic condition (CH2Cl2: MeOH 1:1). The solvents were evaporated to dryness, affording 2 fractions: LBM2.2.1 and LBM2.2.2. Separation of LBM2.2.2 (100 mg) was performed using HPLC to yield 7 sub-fractions: LBM2.2.2.1–2.2.2.7. These sub-fractions were subjected to structural analysis by a Z-Spray electrospray source of a Micromass Q-TOF 2TM hybrid quadrupole time of flight mass spectrometer (Micromass, Manchester, UK), which was operated in a positive ionisation mode at a temperature of 80°C. Nitrogen was used both as a nebulising gas at a flow rate of 12 l.min-1 and as a desolvation gas at a temperature of 150°C. Meanwhile, capillary and cone voltages were set at 3.00 kV and 30 V, respectively. Ultra-high-purity-grade argon was used as the collision gas at a 10 psi inlet pressure for collision-induced dissociation. Collision energy was 35 eV. A scan time of 0.5 s with m/z range of 100–800 was used to obtain the product ions mass spectra. The software used for data acquisition and processing was Mass-Lynx NT, version 4.0 (Micromass). The positive ion scan ESI-MS spectrum of the sub-fractions LBM2.2.2.5 showed the major ions at m/z 449 and its fragment ion of m/z 287, suggesting the loss of one glucose moiety (m/z 162). The product ion mass spectrum of the parent aglycone ion of m/z 287, obtained from liquid chromatography/tandem mass spectrometry (LC-MS/MS) is in agreement with that shown in our previous report [5]. It indicated the prominent ions at m/z 137 and 121, which were indicative of the C-ring cleavage. The possible pathways which was initiated by the loss of H2O, and followed by a series of CO losses, yielding the major product ions of m/z 269, 241, 213, 185, and 157 are in agreement with the structure of the rice bran, cyanidin 3-glucoside (RBC3G).
Human prostate carcinoma cells (PC3) was commercial purchased from American Type Culture Collection (ATCC, Manassas, VA, USA). According to the source, the cells were poor-differentiated adenocarcinoma (neoplastic cell of epithelial origin), having highly metastatic potential. This PC3 cells do not respond to androgens, glucocorticoids or fibroblast growth factors, but are influence by EGF. The cells were cultured in modified Dulbecco’s Modified Eagle F12 Medium (DMEM-F12) (Gibco, Dublin, Ireland) supplemented with 10% fetal bovine serum (FBS; Gibco), 0.1% streptomycin-penicillin, 1% sodium pyruvate, 1% non-essential amino acid and 3.7 g/l of sodium bicarbonate (NaHCO3) as pH buffer agent. The cells were incubated in a 75-cm3 T flask (Corning, NY, USA) under humidified air with 5% CO2 incubator at 37°C.
PC3 cells were cultured in a 96-well plate at a density of 1.8×105 cells/well in 100 µl of DMEM-F12 medium (with 5% FBS) for 24 hours. Then, treated with varied concentrations of LBM, the sub-fractions or the purified fraction for 24, 48, and 72 hours. Water grade I was as vehicle control. After incubation at 24 and 48 hours, the media was removed and 100 μl of PBS containing 10 μl of MTT solution (Sigma-Aldrich, St. Louis, MO, USA) was added, and incubation was continued at 37°C for 4 hours, then replaced with MTT solubilizing solution (100 μl). The color reaction was measured using spectrophotometry, at 570 nm with the reference wavelength at 690 nm. Cell viability calculation was from percentage of survival in the treated and untreated groups.
PC3 cells were cultured on FN-coated glass slide chamber (2×105 cells/well in 500 µl of medium free-serum) and incubated at 37°C, in 5% CO2 for 24 hours then, treated with each test sample for 48 hours. The treated-cells were 4% paraformaldehyde fixed for 20 minutes, and 15 minutes permeabilized with 0.1% Triton X-100 in PBS solution. Cells on cover slips were blocked with 5% BSA/0.25% Triton X-100 for 30 minutes, then co-incubated with 1:500–1:200 dilution of the primary antibodies, β-catenin, α-tubulin, vimentin, F-actin or pFAK (all from Cell Signaling, Danvers, MA, USA); Snail1/2 (Abcam, Cambridge, UK); or Smad2/3 (Santa Cruz Biotechnology, Santa Cruz, CA, USA) in blocking solution, at 4°C overnight. They were then incubated with 1:1,000 dilution FITC-conjugated or Alexa Fluor 555 conjugated secondary antibodies in TBS for 1 hour, incubated with Alexa Fluor 488-Phalliodin for 15 minutes, washed and counter-stained with DAPI. Then, mounted cover slips on slides, and examined the slides by laser scanning confocal microscopy (Olympus FV1000; Olympus, Tokyo, Japan).
To potentiate cell attachment, PC3 cells were grown on fibronectin (FN)-coated plates (FN 5 μg/ml in PBS). A 2×105 cells/well PC3 cells were plated in 6 well culture plates and cultured in a humidified incubator for 24 hours. The cells were treated with LBM, the subsequent fractions, or RBC3G for 48 hours. The treated-cells were trypsinized with 0.25% Trypsin/EDTA and centrifuged at 4,000 rpm, 4°C for 5 minutes. The pellets were washed with cold PBS and RIPA buffer and the cell suspensions were transferred into the tubes, incubated on ice for 30 minutes, mixed and centrifuged at 15,000 rpm, 4°C, for 15 minutes. The supernatants were collected, and total protein concentrations were measured using BCA protein assay kit (Merck, Darmstadt, Germany). Proteins were separated using SDS-polyacrylamide gel, and transferred to nitrocellulose membrane (0.45 μm). The membrane was blocked with a 5% BSA solution for 2 hours before incubation with 1:1,000 dilution of the primary antibodies including; β-catenin, pFAK, vimentin, E-cadherin, MMP9, NF-kB (all from Cell Signaling); Snail1/2; or Smad2/3 overnight at 4°C. After washing, the membrane was incubated with HRP conjugated secondary antibodies for 90 minutes before being developed using ECL Prime Western Blotting Detection kit (BioRad, Hercules, CA, USA). Anti β-actin antibody was used to control for equal loading of proteins.
PC3 cells were cultured in 6-well FN-coated plates with serum free culture medium for 24 hours. The cells were treated with fresh serum free medium containing LBM (0, 250, and 500 µg/ml) or RBC3G (0, 10, and 50 μM) for 24 and 48 hours at 37°C. The conditioned media was collected and cold centrifuged at 3,500 rpm, for 10 minutes. The supernatant was collected and kept in lyophilized form. On assay, the samples were dissolved, and protein concentration was determined. An equal amount of protein was loaded with 2 times of non-reducing loading dye in gel, composed of 4% polyacrylamide stacking gel and 8% polyacrylamide separating gel, containing 0.8% gelatin w/v, 10%SDS PAGE. After electrophoresis, the gels were washed with 50 mM Tris-HCL pH 7.5, 5 mM CaCl2 and 2.5% (v/v) Triton X-100 for 30 minutes. The gel was incubated in buffer (0.05M Tris buffer, pH 7.5 containing 200 mM NaCl, 5 mM CaCl2 and 0.05% NaN3) at 37°C for 18 hours. Then, stained with 0.5% Coomassie brilliant blue R-250 in 5% methanol and 10% acetic acid for 2 hours and de-stained with solution containing 30% methanol and 10% acetic acid at room temperature until the clear bands of MMPs were observed. Protein standards were run to compare the approximate molecular weights that determine the MMPs subtypes.
PC3 cells were cultured in 6 well FN-coated plates with serum free culture medium for 24 hours. Monolayer cells were scratched with a pipette tip to create wounds. The plates were washed with PBS before treatment with various concentrations of the compound or fractions. After 24 and 48 hours incubation at 37°C, the culture supernatant was collected for enzymatic assay (gel zymography). Cell migration images were inspected and photographed under light microscopy. The number of migrating cells was analyzed by counting the total number of cells approaching the area between edges of the scratch wound.
PC3 cells were resuspended to 1.2×105 cells/μl in 500 ml of DMEM-F12 medium. The cells were treated with or without RBC3G (10 and 50 µM) or LBM2.2 (0.5 and 1 mg/ml) for 24 hours before plating onto the upper chamber of extracellular matrix pre-coated transwell plate (Corning Inc., Flintshire, UK). 20% FBS was added in the lower chamber of the wells to serve as a chemo-attractant. The cells were incubated for 24 hours at 37°C, under 5% CO2. After the incubation, the non-invaded cells were removed from the upper surface of the membrane. The invaded cells on the lower surface of the membrane were fixed with 25% methanol for 20 minutes and stained with 5% crystal violet for 15 minutes and counted.
Cell viability assay by MTT showed that the LP rice bran crude extracts (LBMs) and their successive purified fractions possessed a time and concentration-dependent effect on PC3 cell, after 24 and 48 hours incubation (Fig. 1B). Among the three methanol extracts (LBM1, LBM2, and LBM3), LBM2 displayed the greatest inhibitory action on cell viability. At 48 hours incubation assay, LBM2 sub-fractions (LBM2.2 and LBM2.3) maintained their potency on cell viability. The successive LBM2.2 fraction, LBM2.2.2 displayed the highest concentration-dependent activity. After LBM2.2.2 was further fractionated by HPLC and LC/MS/MS a de-glycosylated (aglycone) form of anthocyanins, C3G was identified as a major constituent in the rice bran [20], the RBC3G was assessed to compare the potential effect with the commercial available C3G (Sigma-Aldrich). Both RBC3G and C3G displayed a similar result on PC3 cell viability at concentrations that reduced 50% cell survival (IC50; approximately 167.8±0.06 µM). To avoid the cytotoxic induced cell death effect, the experiments in this study were performed using RBC3G concentrations less than 100 µM.
The immuno-fluorescent studies illustrated the untreated control PC3 cells cultured on FN-coated plate that expressed an EMT signaling protein, β-catenin, in a clumping pattern around the perinuclear region (Fig. 2A, left row), while some distributed in a dotted/linear pattern, on the cell surface and/or the cell-cell adhesion area (Fig. 2B, left row). A profuse distribution of polymerized alpha-tubulin forming microtubules appeared in the cytoplasm (Fig. 2A, left row). Both suggested the potentially active cell motility. In addition, the immune-staining for F-actin cytoskeleton appeared on the cell surface was co-localized with its organizing protein, p-FAK, which was in a linear pattern and dotted pattern, respectively (Fig. 2B and C, left rows), suggesting the potentially active migration capability of PC3 cell. Treatment of PC3 cells with the RBC3G (Fig. 2, middle & right rows) demonstrated a concentration-dependent effect on the reduction of β-catenin (Fig. 2A and B, middle & right rows), F-actin (Fig. 2B and C, middle and right rows) and p-FAK (Fig. 2C, right row) immuno-intensity and alteration of their distribution patterns comparing to the untreated controls. The distribution of EMT associated-proteins; E-cadherin (epithelial phenotypic marker) and vimentin (mesenchymal phenotypic marker) in the untreated PC3 cells showed vimentin expression as a clump at the perinuclear area of almost every single cell (Fig. 2D, left row). RBC3G treatment, especially with 100 μM concentration, decreased vimentin positive cells and its staining pattern (Fig. 2D, right row). Unfortunately, attempts to demonstrate the expression of E-cadherin by immunofluorescent assay was unsatisfied (data not shown). The distribution of Smad proteins involved in classical signaling pathway for growth factor mediated cell proliferation, differentiation and migration in most cancer cells revealed that in the untreated PC3 cells (control), Smad2/3 immuno-fluorescent staining was distributed in nuclei as a dense aggregation complex (Fig. 2E, left row). RBC3G treatment significantly decreased the immuno-intensity of Smad2/3 in the nucleus and deccreased the number of Smad positive cells (Fig. 2E, right row), suggesting it’s active role in transcriptional regulaion of the cancer cells. In addition, expression of Snail, the downstream effectors of Smad, demonstrated a profuse Snail1/2 immuno-fluorescent staining localized in the nucleus and some distributed in the cytoplasm of the untreated PC3 cells (Fig. 2F, left row). Treatment of PC3 cells with high concentration RBC3G (100 μM) markedly reduced the distribution of Snail1/2 both in the nucleus and the cytoplasm (Fig. 2F, right row). These immuno-stained results were in good correlated with the Western blot analysis of β-catenin and p-FAK (Fig. 2G), Vimentin and E-cadherin (Fig. 2H), Smad2/3 and Snail1/2 (Fig. 2I and J) demonstrated the stage of PC3 cell transformation which could be inhibited with RBC3G.
Western blots analysis for proteins involved in metastasis and invasion; MMP-9 and NF-κB (nuclear factor kappa-light-chain-enhancer of activated B cells), revealed significant reduction of MMP-9 and NF-κB proteins production in PC3 cells treated with RBC3G in a concentration dependent manner (Fig. 3A). Assessment for the activity of matrix degradation enzyme that potentiate metastasis revealed significantly reduction of this matrix degradation enzyme MMP-9 also in a concentration-dependent manner (Fig. 3B).
On Scratch/wound healing assay (Fig. 4A), treatment of monolayer cultured PC3 cells with RBC3G (10–50 µM) for 24–48 hours showed decreased cell migration toward the wound area in a concentration dependent manner. The counting number of PC3 cell migration toward the wound area was significant reduced, relative to the untreated controls. On the invasion assay (Fig. 4B), the invading cells showed a spindle fibroblast-like morphology. Treatment of the cells with RBC3G (10 and 50 µM) 24 hours before plating resulted in inhibition of the invasion capability of the cells through matrix gel-coated Transwell plates. The counting number of invaded cells showed significantly reduction of the treated cells compared to the control.
The results from this study identified cyanidine-3-glucoside in the Thai black rice bran (LP variety) as an anti-cancer agent that inhibited prostate cancer transformation and delay the progression of prostate cancer, including cell proliferation, transformation, migration, and invasion. This chemical isolated compound derived from successive purifying fractions of the Thai black rice bran, RBC3G, is primary presented in high amount as a natural aglycone form of anthocyanins that never been reported for constituents in this plant. The bioactive activities, specific compound properties and its mechanism of action that exerted on invasive prostate cancer PC3 cells is the anti-cancer progression. Previously, several studies had reported cyanindin-3-glucoside from fruits affecting carcinogenesis through many pathways. For example, it inhibits proliferation of human cancer cell lines by suppression of MAPK activity [33]. It is a potent antioxidant, particularity against OH- and O2 radicals [5, 33]. Cyanindin-3-glucoside induces apoptosis of cancer cells via caspase-3 cleavage, DNA fragmentation via a Bcl-2/Bax pathway [17, 34], and induces cell cycle arrest via a cyclin dependent pathway [35]. These pathways are primarily concentrated on induced apoptotic cell death, which remarked as criteria for a cancer therapeutic agent. However, the resulting cell death effect of several compounds is mostly from the high concentration of the compound under examined in vitro. To be an effective anti-cancer agent in vivo, it would require a high amount of the compound from dietary fruits or vegetables which consumable is limited and it could result in unpredictable side effects or toxicity. The biochemical and cell biological explorations in this study suggest that with appropriate concentration, the natural cyanindin-3-glucoside could inhibit the PC3 cancer cell behaviors or its EMT characteristics via reversal of the EMT process. Thus, cyanindin-3-glucoside contained in rice bran or other dietary fruits and vegetable appear to contribute to cancer prevention than anti-cancer therapeutic. Or, it could be potentiated to further development as a drug or supplement that act as a weak anti-cancer agent.
The evident presented in this study clearly demonstrated that RBC3G decreased the percent PC3 cell survival in a concentration-dependent manner, reduced the expression of β-catenin and p-FAK, and induced cytoskeletal remodeling within the cells, leading to a delaying of cancer transformation process. The results were correlated well with the former observations showing that during this process, β-catenin, mostly distributed on the cell surface and in the perinuclear region, was dislodged from F-actin association and translocated from the cell adhesion junction, by the aid of cytoplasmic microtubules, into the nucleus [30, 36]. RBC3G inhibited both β-catenin expression and its chemical signaling cascade, thus inhibiting its dissociation and translocation from the cell periphery and playing significant roles in the signaling of cell differentiation through the process of EMT. Consequently, the data suggest that RBC3G reduces p-FAK expression in the PC3 cells and decreases its distribution at the cell periphery, which would reduce cell-matrix interaction. The immuno-labeled α-tubulin in the transformed PC3 cells reflected microtubule formation, indicating the high invasive potential of the cell. RBC3G reduces microtubule formation and appears to reduce the cell motility capability. Therefore, RBC3G could prevent the cell from successive migration and invasion. The anti-metastasis mechanism of RBC3G relative to PC3 cells defined through signaling inhibition of molecules essential to epithelial-mesenchymal transition.
To a better understanding of the detailed mechanism(s) by which RBC3G inhibits EMT, we studied the expression and distribution of Smad since Smad signaling pathway appears to be activating in prostate cancer PC3 cell. Both immunohistochemical and western blot analysis demonstrated that in addition to β-catenin reduction, the inhibition of EMT by RBC3G was associated with a reduction in Smad and Snail transcriptional factors. Smad signaling regulated by the growth factor receptor such as TGF-β, which activates the phosphorylation and formation of Smad complex and then moves into the nucleus to regulate the transcription of many genes [30, 31, 37]. We found that PC3 cell expressed high level of both Smad and Snail. The untreated PC3 cell displayed intense distribution of immunostaining for Smad2/3, mainly aggregated within the nucleus. RBC3G significantly reduced the Smad2/3 expression and diminished its nuclei localization, aa well as decreased the number of Smad positive cells. The cells also expressed high level of Snail, and Snail1/2 complex was found over the nucleus and in the cytoplasm. RBC3G treatment decreased the cytoplasmic distribution of Snail1/2, but did not reduced Snail1/2 concentrated in the nucleus. These data suggest that RBC3G inhibits translocation of β-catenin and Smad transcriptional factors into the nucleus, thus inhibiting EMT. Meanwhile, RBC3G treated PC3 cells reduced the Snail transcription and expression that was high during EMT development and cancer transformation leading to inhibition of E-cadherin [30]. Relative to Snail expression by RBC3G, the current data indicate an alteration of expression level and distribution of E-cadherin and vimentin (tumor marker proteins) in the treated cells. Obviously, RBC3G significantly reduced vimentin expression (0.53–0.45 folds) and reduced vimentin immuno-intensity in the cells. As defined by Western blot analysis, RBC3G also increased the expression level of E-cadherin in the treated PC3 cells. The results suggest that RBC3G could effectively delay the cancer transformation process or it could potentially reverse prostate cancer cells conversion from mesenchymal to epithelial cell type. This consequence mechanism on cell differentiation never been explained before, but it associates with the previous study demonstrating that C3G potentiate diffentiation of PC3 and DU145 cells by increased the level of P75NGFR tumor suppressor leading the cells to normal-like cell phenotype Sorrenti et al. [17].
The effectiveness of RBC3G on delaying cancer progression exhibited by the significant reduction of MMP-9 enzyme levels, which correlated well with the reduction of NF-κB, a transcription factor associated with cell migration activation in PC3 cells. These inhibitory effects were resulted from the initial reduction of NF-κB expression and its signaling activity that subsequently suppressed MMP-9 production and decreased matrix degradation activity. RBC3G had anti-metastatic and anti-invasion effects on PC3 cell biological activity in the inhibition assays. This result agrees with previous studies that reported on other plants derived anthocyanins [1, 7, 33, 38-40]. Precisely, analysis for cellular responses suggests that the major phytochemical constituent in the RBC3G, possesses anti-cancer properties at a very early stage of malignancy in this invasive prostate cancer cell line.
In conclusion, this study indicates that at least in vitro, RBC3G inhibits EMT during prostate cancer transformation through Smad signaling pathway mediating a proper Snail and E-cadherin expression for controlling the cell behaviors. RBC3G also exerts an inhibitory effect on prostate cancer cells by decreasing NF-kB mediated MMP-9 expression and activation, thus, reducing the cells aggressive potential. Therefore, RBC3G could be a promising candidate compound for prostate cancer prevention and/or being a supplement to drug(s) during anti-cancer intervention.
Acknowledgements
We thank the Center of Nanoimaging, Faculty of Science, Mahidol University for providing accessible and advice to confocal laser scanning microscope usage.
Notes
References
1. Matchett MD, MacKinnon SL, Sweeney MI, Gottschall-Pass KT, Hurta RA. 2006; Inhibition of matrix metalloproteinase activity in DU145 human prostate cancer cells by flavonoids from lowbush blueberry (Vaccinium angustifolium): possible roles for protein kinase C and mitogen-activated protein-kinase-mediated events. J Nutr Biochem. 17:117–25. DOI: 10.1016/j.jnutbio.2005.05.014. PMID: 16111875.
2. Lu JN, Lee WS, Kim MJ, Yun JW, Jung JH, Yi SM, Jeong JH, Kim HJ, Choi YH, Kim GS, Ryu CH, Shin SC. 2014; The inhibitory effect of anthocyanins on Akt on invasion and epithelial-mesenchymal transition is not associated with the anti-EGFR effect of the anthocyanins. Int J Oncol. 44:1756–66. DOI: 10.3892/ijo.2014.2315. PMID: 24585214.


3. Somintara S, Leardkamolkarn V, Suttiarporn P, Mahatheeranont S. 2016; Anti-tumor and immune enhancing activities of rice bran gramisterol on acute myelogenous leukemia. PLoS One. 11:e0146869. DOI: 10.1371/journal.pone.0146869. PMID: 26752299. PMCID: PMC4709086.


4. Srisuwan S, Arkaravichien T, Mahatheeranont S, Puangsombat P, Seekhaw P, Noenplab ANL, Sattayasai J. 2015; Effects of aqueous extract of unpolished dark purple glutinous rice, Var Luem Pua, on ROS in SK-N-SH cells and scopolamine-induced memory deficit in mice. Trop J Pharm Res. 14:1635–41.


5. Pitija K, Nakornriab M, Sriseadka T, Vanavichit A, Wongpornchai S. 2013; Anthocyanin content and antioxidant capacity in bran extracts of some Thai black rice varieties. Int J Food Sci Tech. 48:300–8. DOI: 10.1111/j.1365-2621.2012.03187.x.


6. Wang LS, Stoner GD. 2008; Anthocyanins and their role in cancer prevention. Cancer Lett. 269:281–90. DOI: 10.1016/j.canlet.2008.05.020. PMID: 18571839. PMCID: PMC2582525.


7. Chen PN, Kuo WH, Chiang CL, Chiou HL, Hsieh YS, Chu SC. 2006; Black rice anthocyanins inhibit cancer cells invasion via repressions of MMPs and u-PA expression. Chem Biol Interact. 163:218–29. DOI: 10.1016/j.cbi.2006.08.003. PMID: 16970933.


8. Miller KD, Siegel RL, Lin CC, Mariotto AB, Kramer JL, Rowland JH, Stein KD, Alteri R, Jemal A. 2016; Cancer treatment and survivorship statistics, 2016. CA Cancer J Clin. 66:271–89. DOI: 10.3322/caac.21349. PMID: 27253694.


9. Lin HC, Lin JY. 2016; Immune cell-conditioned media suppress prostate cancer PC-3 cell growth correlating with decreased proinflammatory/anti-inflammatory cytokine ratios in the media using 5 selected crude polysaccharides. Integr Cancer Ther. 15:NP13–25. DOI: 10.1177/1534735415627923. PMID: 27130724. PMCID: PMC5739154.


10. Seidi K, Jahanban-Esfahlan R, Abasi M, Abbasi MM. 2016; Anti tumoral properties of Punica granatum (Pomegranate) seed extract in different human cancer cells. Asian Pac J Cancer Prev. 17:1119–22. DOI: 10.7314/APJCP.2016.17.3.1119. PMID: 27039735.


11. Modaeinama S, Abasi M, Abbasi MM, Jahanban-Esfahlan R. 2015; Anti tumoral properties of Punica granatum (Pomegranate) peel extract on different human cancer cells. Asian Pac J Cancer Prev. 16:5697–701. DOI: 10.7314/APJCP.2015.16.14.5697. PMID: 26320438.


12. Peng CC, Peng CH, Chen KC, Hsieh CL, Peng RY. 2011; The aqueous soluble polyphenolic fraction of psidium guajava leaves exhibits potent anti-angiogenesis and anti-migration actions on DU145 cells. Evid Based Complement Alternat Med. 2011:219069. DOI: 10.1093/ecam/neq005. PMID: 21799674. PMCID: PMC3135903.
13. Chen KC, Peng CC, Chiu WT, Cheng YT, Huang GT, Hsieh CL, Peng RY. 2010; Action mechanism and signal pathways of Psidium guajava L. aqueous extract in killing prostate cancer LNCaP cells. Nutr Cancer. 62:260–70. DOI: 10.1080/01635580903407130. PMID: 20099201.
14. Albrecht M, Jiang W, Kumi-Diaka J, Lansky EP, Gommersall LM, Patel A, Mansel RE, Neeman I, Geldof AA, Campbell MJ. 2004; Pomegranate extracts potently suppress proliferation, xenograft growth, and invasion of human prostate cancer cells. J Med Food. 7:274–83. DOI: 10.1089/jmf.2004.7.274. PMID: 15383219.


15. Salehi B, Fokou PVT, Yamthe LRT, Tali BT, Adetunji CO, Rahavian A, Mudau FN, Martorell M, Setzer WN, Rodrigues CF, Martins N, Cho WC, Sharifi-Rad J. 2019; Phytochemicals in prostate cancer: from bioactive molecules to upcoming therapeutic agents. Nutrients. 11:1483. DOI: 10.3390/nu11071483. PMID: 31261861. PMCID: PMC6683070.


16. Zhang ZH, Xie DD, Xu S, Xia MZ, Zhang ZQ, Geng H, Chen L, Wang DM, Wei W, Yu DX, Xu DX. 2017; Total glucosides of paeony inhibits lipopolysaccharide-induced proliferation, migration and invasion in androgen insensitive prostate cancer cells. PLoS One. 12:e0182584. DOI: 10.1371/journal.pone.0182584. PMID: 28783760. PMCID: PMC5544245.


17. Sorrenti V, Vanella L, Acquaviva R, Cardile V, Giofrè S, Di Giacomo C. 2015; Cyanidin induces apoptosis and differentiation in prostate cancer cells. Int J Oncol. 47:1303–10. DOI: 10.3892/ijo.2015.3130. PMID: 26315029.


18. Roviello GN, Iannitti R, Roviello V, Palumbo R, Simonyan H, Vicidomini C. 2017; Synthesis and biological evaluation of a novel Amadori compound. Amino Acids. 49:327–35. DOI: 10.1007/s00726-016-2363-4. PMID: 27864693.


19. Roviello GN, Iannitti R, Palumbo R, Simonyan H, Vicidomini C, Roviello V. 2017; Lac-L-TTA, a novel lactose-based amino acid-sugar conjugate for anti-metastatic applications. Amino Acids. 49:1347–53. DOI: 10.1007/s00726-017-2433-2. PMID: 28478584.


20. Carella A, Roviello V, Iannitti R, Palumbo R, La Manna S, Marasco D, Trifuoggi M, Diana R, Roviello GN. 2019; Evaluating the biological properties of synthetic 4-nitrophenyl functionalized benzofuran derivatives with telomeric DNA binding and antiproliferative activities. Int J Biol Macromol. 121:77–88. DOI: 10.1016/j.ijbiomac.2018.09.153. PMID: 30261256.


21. Voulgari A, Pintzas A. 2009; Epithelial-mesenchymal transition in cancer metastasis: mechanisms, markers and strategies to overcome drug resistance in the clinic. Biochim Biophys Acta. 1796:75–90. DOI: 10.1016/j.bbcan.2009.03.002. PMID: 19306912.


22. Kim DH, Xing T, Yang Z, Dudek R, Lu Q, Chen YH. 2017; Epithelial mesenchymal transition in embryonic development, tissue repair and cancer: a comprehensive overview. J Clin Med. 7:1. DOI: 10.3390/jcm7010001. PMID: 29271928. PMCID: PMC5791009.


23. Lamouille S, Xu J, Derynck R. 2014; Molecular mechanisms of epithelial-mesenchymal transition. Nat Rev Mol Cell Biol. 15:178–96. DOI: 10.1038/nrm3758. PMID: 24556840. PMCID: PMC4240281.


24. Vuoriluoto K, Haugen H, Kiviluoto S, Mpindi JP, Nevo J, Gjerdrum C, Tiron C, Lorens JB, Ivaska J. 2011; Vimentin regulates EMT induction by Slug and oncogenic H-Ras and migration by governing Axl expression in breast cancer. Oncogene. 30:1436–48. DOI: 10.1038/onc.2010.509. PMID: 21057535.


25. Tran NL, Nagle RB, Cress AE, Heimark RL. 1999; N-Cadherin expression in human prostate carcinoma cell lines. An epithelial-mesenchymal transformation mediating adhesion withStromal cells. Am J Pathol. 155:787–98. DOI: 10.1016/S0002-9440(10)65177-2. PMID: 10487836. PMCID: PMC1866912.
26. Valenta T, Hausmann G, Basler K. 2012; The many faces and functions of β-catenin. EMBO J. 31:2714–36. DOI: 10.1038/emboj.2012.150. PMID: 22617422. PMCID: PMC3380220.


27. Zhang Q, Bai X, Chen W, Ma T, Hu Q, Liang C, Xie S, Chen C, Hu L, Xu S, Liang T. 2013; Wnt/β-catenin signaling enhances hypoxia-induced epithelial-mesenchymal transition in hepatocellular carcinoma via crosstalk with hif-1α signaling. Carcinogenesis. 34:962–73. DOI: 10.1093/carcin/bgt027. PMID: 23358852.


28. Lim J, Thiery JP. 2012; Epithelial-mesenchymal transitions: insights from development. Development. 139:3471–86. DOI: 10.1242/dev.071209. PMID: 22949611.


29. Burk U, Schubert J, Wellner U, Schmalhofer O, Vincan E, Spaderna S, Brabletz T. 2008; A reciprocal repression between ZEB1 and members of the miR-200 family promotes EMT and invasion in cancer cells. EMBO Rep. 9:582–9. DOI: 10.1038/embor.2008.74. PMID: 18483486. PMCID: PMC2396950.


30. Clevers H. 2006; Wnt/beta-catenin signaling in development and disease. Cell. 127:469–80. DOI: 10.1016/j.cell.2006.10.018. PMID: 17081971.
31. Tu B, Peng ZX, Fan QM, Du L, Yan W, Tang TT. 2014; Osteosarcoma cells promote the production of pro-tumor cytokines in mesenchymal stem cells by inhibiting their osteogenic differentiation through the TGF-β/Smad2/3 pathway. Exp Cell Res. 320:164–73. DOI: 10.1016/j.yexcr.2013.10.013. PMID: 24183998.


32. Wang Y, Shi J, Chai K, Ying X, Zhou BP. 2013; The role of snail in EMT and tumorigenesis. Curr Cancer Drug Targets. 13:963–72. DOI: 10.2174/15680096113136660102. PMID: 24168186. PMCID: PMC4004763.


33. Ding M, Feng R, Wang SY, Bowman L, Lu Y, Qian Y, Castranova V, Jiang BH, Shi X. 2006; Cyanidin-3-glucoside, a natural product derived from blackberry, exhibits chemopreventive and chemotherapeutic activity. J Biol Chem. 281:17359–68. DOI: 10.1074/jbc.M600861200. PMID: 16618699.


34. Cho E, Chung EY, Jang HY, Hong OY, Chae HS, Jeong YJ, Kim SY, Kim BS, Yoo DJ, Kim JS, Park KH. 2017; Anti-cancer effect of cyanidin-3-glucoside from mulberry via caspase-3 cleavage and DNA fragmentation in vitro and in vivo. Anticancer Agents Med Chem. 17:1519–25. DOI: 10.2174/1871520617666170327152026. PMID: 28356020.


35. Chen PN, Chu SC, Chiou HL, Chiang CL, Yang SF, Hsieh YS. 2005; Cyanidin 3-glucoside and peonidin 3-glucoside inhibit tumor cell growth and induce apoptosis in vitro and suppress tumor growth in vivo. Nutr Cancer. 53:232–43. DOI: 10.1207/s15327914nc5302_12. PMID: 16573384.
36. Lin BW, Gong CC, Song HF, Cui YY. 2017; Effects of anthocyanins on the prevention and treatment of cancer. Br J Pharmacol. 174:1226–43. DOI: 10.1111/bph.13627. PMID: 27646173. PMCID: PMC5429338.


37. Yun CY, Choi H, You YJ, Yang JY, Baek JA, Cho ES. 2016; Requirement of Smad4-mediated signaling in odontoblast differentiation and dentin matrix formation. Anat Cell Biol. 49:199–205. DOI: 10.5115/acb.2016.49.3.199. PMID: 27722013. PMCID: PMC5052229.


38. Chen PN, Chu SC, Chiou HL, Kuo WH, Chiang CL, Hsieh YS. 2006; Mulberry anthocyanins, cyanidin 3-rutinoside and cyanidin 3-glucoside, exhibited an inhibitory effect on the migration and invasion of a human lung cancer cell line. Cancer Lett. 235:248–59. DOI: 10.1016/j.canlet.2005.04.033. PMID: 15975709.


39. Rugină D, Sconţa Z, Leopold L, Pintea A, Bunea A, Socaciu C. 2012; Antioxidant activities of chokeberry extracts and the cytotoxic action of their anthocyanin fraction on HeLa human cervical tumor cells. J Med Food. 15:700–6. DOI: 10.1089/jmf.2011.0246. PMID: 22846076. PMCID: PMC3407391.


40. Xu M, Bower KA, Wang S, Frank JA, Chen G, Ding M, Wang S, Shi X, Ke Z, Luo J. 2010; Cyanidin-3-glucoside inhibits ethanol-induced invasion of breast cancer cells overexpressing ErbB2. Mol Cancer. 9:285. DOI: 10.1186/1476-4598-9-285. PMID: 21034468. PMCID: PMC2984473.


Fig. 1
(A) Scheme of extraction, fractionation and purification of LP rice bran to yield the cyanidin-3-O-glucoside. (B) MTT assay showing the effect of rice bran methanol fractions (LBM1, LBM2, and LBM3), sub-fractions (LBM2.1, LBM2.2, and LBM2.3), semi-purified fractions (LBM2.2.1, LBM2.2.2) and the purified RBC3G on viability of PC3 cells in culture. The assay was performed after 24 and 48 hours treatments. LC-MS/MS, liquid chromatography/tandem mass spectrometry; LP, Luem Pua; RBC3G, rice bran, cyanidin 3-glucoside. Data was expressed as mean±standard error of the mean. *P<0.05, compared to control groups.
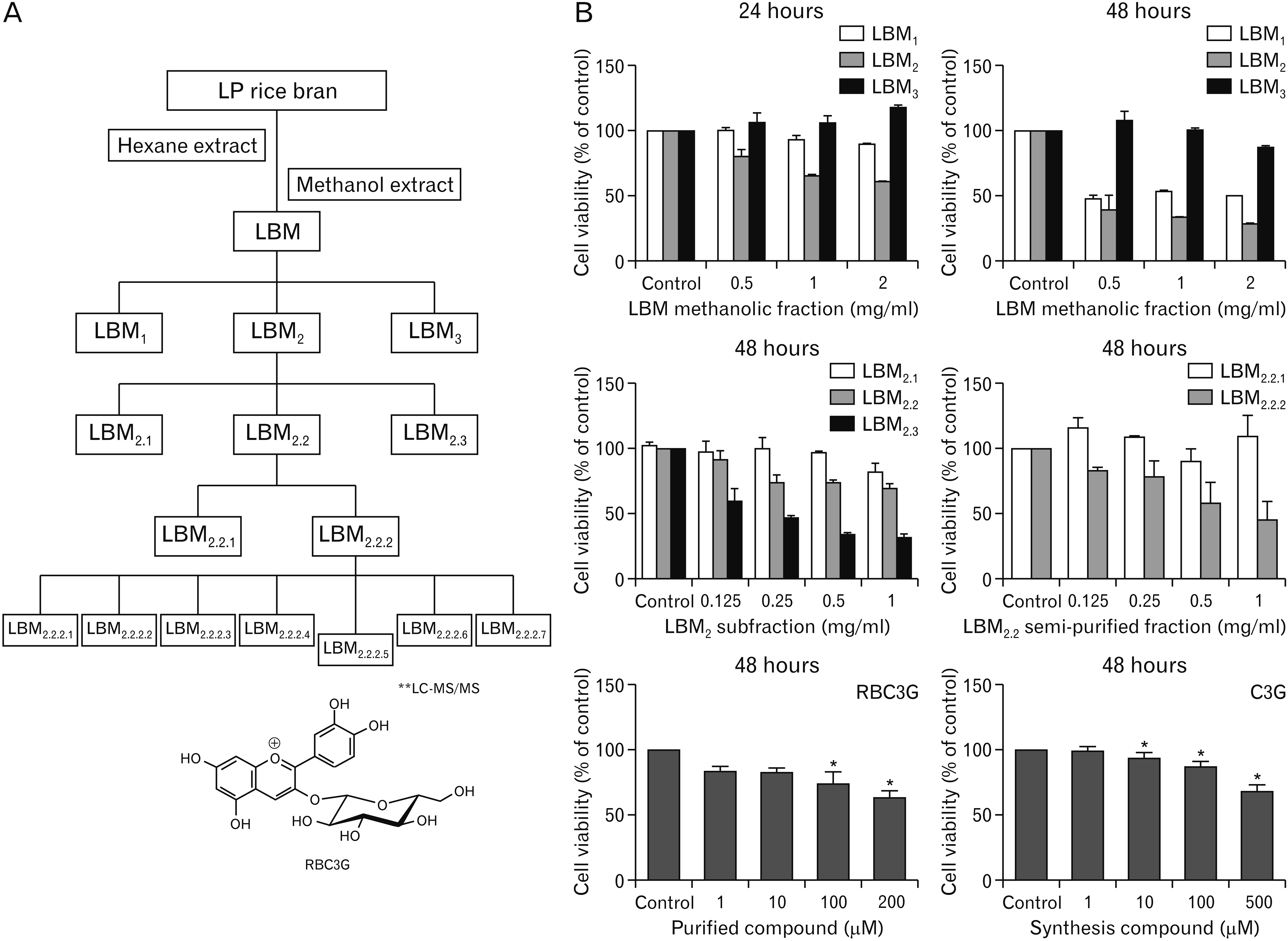
Fig. 2
(A–F) Confocal laser scanning micrographs of untreated PC3 cells (left row) and RBC3G treated PC3 cells (middle and right rows), cultured on FN-coated plate, showing the distribution patterns of proteins associated with EMT characteristics, cytoskeletal organization, and their signaling by double immuno-stained with antibodies to (A) β-catenin, probed with TRITC (red) and alpha-tubulin, probed with FITC (green). (B) β-catenin, probed with TRITC (red) and F-actin, probed with FITC (green). (C) pFAK-Alexa555 (red) and F-actin-Alexa488 (green). (D) Vimentin-conjugated Alexa555 (red). (E) Smad2/3-conjugated Alexa555 (red) and F-actin, probed with FITC (green). (F) Snail1/2-conjugated Alexa555 (red) and F-actin-Alexa488 (green). Nuclei were counter-stained with DAPI (blue), Scale bars=30 μm. (G–I) Western blot analysis of proteins associated with EMT characteristics and their signaling proteins involved in tumor transformation in the untreated and RBC3G treated PC3 cells, probed with antibodies to (G) β-catenin and pFAK, (H) E-cadherin and vimentin, (I) Smad2/3 and Snail1/2. The reactive bands density calculated in relative to β-actin. EMT, epithelial mesenchymal transition; FITC, fluorescein isothiocyanate; RBC3G, rice bran, cyanidin 3-glucoside; TRITC, tetramethylrhodamine isothiocyanate. The data presented as mean±standard error of the mean of triplicate independent experiments. *P<0.05 compared to control.

Fig. 3
(A) Western blot analysis of proteins associated with cell migration and invasion, MMP-9 and NF-κB, in PC3 cells after treatment with varied concentrations of RBC3G. The bands density of each protein calculated in relative to β-actin. The data presented as mean±standard error of the mean of triplicate independent experiments. (B) Zymography assay for MMP-9 enzyme released from PC3 cells during the treatment with RBC3G. The enzyme activity demonstrated from gelatinolytic band molecular weight (75 kDa). The bar graphs show relative bands intensity of the treated PC3 cells compared to untreated control. FN, fibronectin; MMP-9, matrix metalloproteinase-9; NF-κB, nuclear factor-kappa B; RBC3G, rice bran, cyanidin 3-glucoside. *P<0.05 compared to control. #P<0.05 compared to FN-coated plate control.
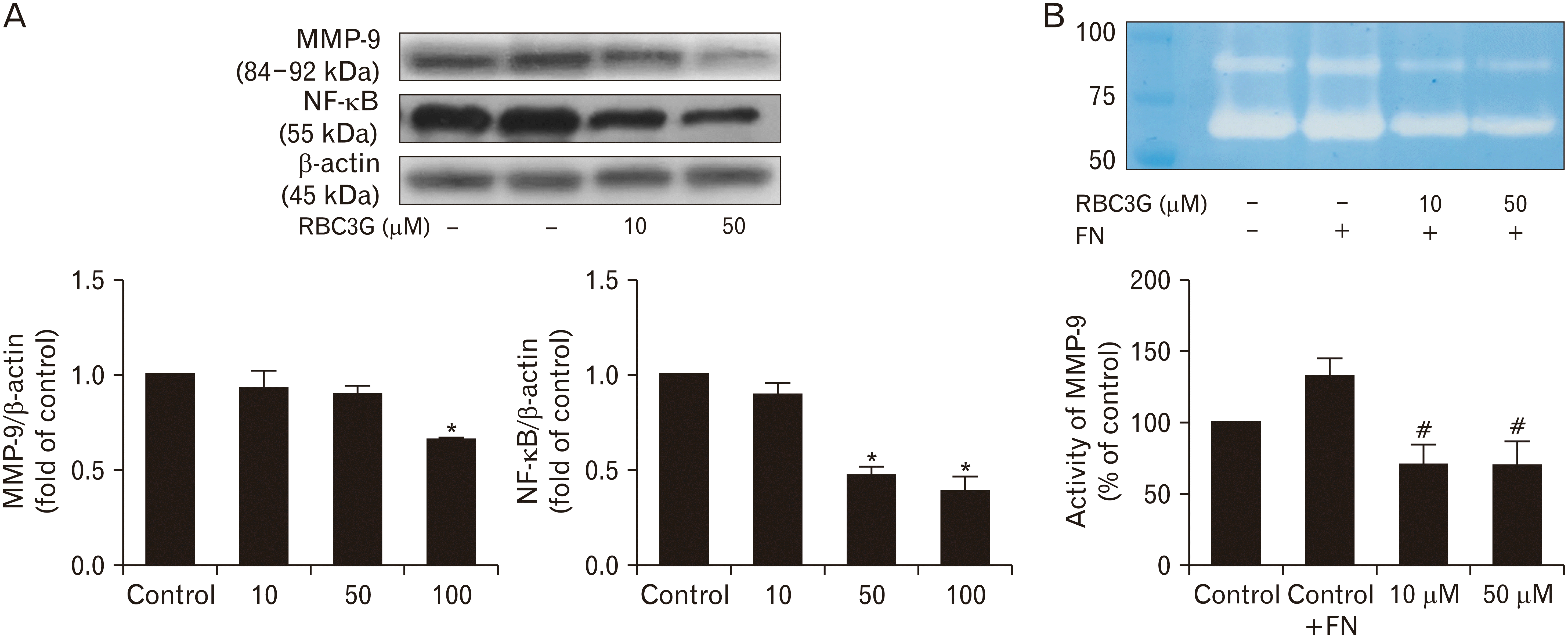
Fig. 4
(A) The phase contrast micrographs from scratch/wound healing assay shows the effect of RBC3G on migration of PC3 cells after 24 and 48 hours treatment. The bar graph shows the counting number of migrating cells into the scratched area in comparison with the controls (non-coated plate+PBS treatment and FN-coated plate+PBS treatment). (B) Cell invasion assay; through matrix gel-coated Transwell plate shows the inhibiting effect of RBC3G on PC3 cells. The bar graph shows the counting number of the invaded cells. FN, fibronectin; RBC3G, rice bran, cyanidin 3-glucoside. Data expressed as mean±standard error of the mean (n=3). ***P<0.001, compared to control groups. *P<0.05, compared to control groups.
