Abstract
Alpha-synuclein (α-syn) is a presynaptic protein that is richly expressed in the central and peripheral nervous systems of mammals, and it is related to the pathogenesis of Parkinson's disease and other neurodegenerative disorders. In the present study, we compared the distribution of the immunoreactivity of α-syn and its related gliosis in the spinal cord of young adult (2-3 years) and aged (10-12 years) beagle dogs. We discovered that α-syn immunoreactivity was present in many neurons in the thoracic level of the aged spinal cord, however, its protein level was not distinct inform that of the adult spinal cord. In addition, ionized calcium-binding adapter molecule-1 (a marker for microglia) immunoreactivity, and not glial fibrillary acidic protein (a marker for astrocytes) immunoreactivity, was somewhat increased in the aged group compared to the adult group. These results indicate that α-syn immunoreactivity was not dramatically changed in the dog spinal cord during aging.
Alpha-synuclein (α-syn) is a soluble acidic protein of 140 amino acids widely presented in presynaptic sites in the central nervous system (CNS) of mammals, particularly in the neocortex, hippocampus, and striatum [1,2]. α-Syn normally participates in the homeostasis of synaptic vesicles [3], related to synaptic transmission, neuronal plasticity and lipid transport [4-6].
α-Syn is also related to neurodegenerative diseases, as an aggregation of α-syn constitutes the main component of Lewy bodies (LB) which are considered the neuropathological hallmark of Parkinson's disease, Alzheimer's disease, and dementia with Lewy bodies (DLB) [7,8]. α-Syn related pathology is found not only in the brain, but also in the spinal cord, such as multiple system atrophy (MSA), amyotrophic lateral sclerosis (ALS) [9,10].
The accumulation of misfolded α-syn in neuronal cell bodies, axons and synapses leads to glial and neuronal dysfunction and death in the various neurodegenerative diseases, and these disorders are classified as synucleinopathies [7,11,12].
During normal aging, the disease susceptibility is increased [13], because the spinal cord undergoes various neurochemical and structural changes [14-16]. There are some reports on the distribution of α-syn and its expression pattern in neurodegenerative diseases in various species, however, no study has yet demonstrated the age-related changes of α-syn in the dog spinal cord. Hence, in the present study, we compared the immunoreactivity of α-syn in the spinal cord between the adult and aged beagle dog.
Clinically and neurologically normal male beagle dogs were used at 2-3 years (young adult dogs, n=12) and 10-12 years (aged dogs, n=12) of age, with normal values in blood count, chemistry, gas analysis and serum electrolytes. The animal protocol used in this study was reviewed and approved based on ethical procedures and scientific care by the Kangwon National University-Institutional Animal Care and Use Committee (KIACUC-12-0010).
For histochemical analysis, young adult and aged dogs (n=7 in each group) were anesthetized with zoletil50 (8 mg/kg) and xylazine (2 mg/kg) mixture and perfused transcardially with 0.1 M phosphate-buffered saline (PBS, pH 7.4) followed by 4% paraformaldehyde in 0.1 M phosphate buffer (PB, pH 7.4). The cervical (C6-C8), thoracic (T9-T10) and lumbar (L5-L6) spinal cord were removed and posfixed in the same fixative for 12 h. The spinal cord tissues were cryoprotected by infiltration with 30% sucrose overnight. Thereafter the frozen tissues were serially sectioned on a cryostat (Leica, Wetzlar, Germany) into 30 µm in thickness, and the sections were then collected into six-well plates containing PBS.
To obtain the accurate data for immunohistochemistry, the free-floating sections were carefully processed under the same conditions. Immunohistochemistry for α-syn, glial fibrillary acidic protein (GFAP, a marker for astrocytes) and ionized calcium-binding adapter molecule-1 (Iba-1, a marker for microglia) was performed under the same conditions in dogs of different ages in order to examine whether the degree of immunohistochemical staining was accurate. The sections were sequentially treated with 0.3% H2O2 and 10% normal goat serum. They were then incubated with diluted sheep anti-α-syn (diluted 1:1000, Abcam, Cambridge, MA), mouse anti-GFAP (diluted 1:800, Chemicon, Temecula, CA) and rabbit anti-Iba-1 (diluted 1:800, Wako, Richmond, VA), respectively, and subsequently exposed to biotinylated rabbit anti-sheep, goat anti-rabbit or horse anti-mouse IgG and streptavidin peroxidase complex (1:200, Vector, Burlingame, CA). They were then visualized by staining with 3,3'-diaminobenzidine tetrahydrochloride (Sigma, St. Louis, MO) in 0.1 M Tris-HCl buffer (pH 7.2) and mounted on gelatin-coated slides. The sections were mounted in Canada balsam (Kanto) following dehydration. In order to establish the specificity of the immunostaining, a negative control test was carried out with pre-immune serum instead of primary antibody. The negative control test resulted in the absence of immunoreactivity in all structures.
To confirm change in α-syn, GFAP and Iba-1 levels in the cervical, thoracic and lumbar spinal cord between the adult and aged dog, the animals (n=5 in each group) were sacrificed and used for the western blot analysis. In brief, the tissues were homogenized in 50 mM PBS (pH 7.4) containing EGTA (pH 8.0), 0.2% NP-40, 10 mM EDTA (pH 8.0), 15 mM sodium pyrophosphate, 100 mM β-glycerophosphate, 50 mM NaF, 150 mM NaCl, 2 mM sodium orthovanadate, 1 mM PMSF, and 1 mM DTT. After centrifugation, the protein level in the supernatants was determined using a Micro BCA protein assay kit (Pierce Chemical, Rockford, IL, USA). Aliquots containing 20 µg of total protein were boiled in a loading buffer containing 150 mM Tris (pH 6.8), 3 mM DTT, 6% SDS, 0.3% bromophenol blue, and 30% glycerol. The aliquots were then loaded onto a polyacrylamide gel. After electrophoresis, the gels were transferred to nitrocellulose transfer membranes (Pall Crop, East Hills, NY, USA). To reduce background staining, the membranes were incubated with 5% non-fat dry milk in PBS containing 0.1% Tween 20, followed by incubation with sheep anti-α-syn (diluted 1:1000, Abcam, Cambridge, MA), rabbit anti-Iba-1 (diluted 1:1000, Wako, Richmond, VA), and mouse anti-GFAP (diluted 1:1000, Chemicon, Temecula, CA), peroxidase-conjugated donkey anti-sheep, rabbit or mouse IgG (Sigma, St. Louis, MO, USA) and ECL kit (Pierce Chemical). The result of western blot analysis was scanned, and densitometric analysis for the quantification of the bands was done using Scion Image software (Scion Corp., Frederick, MD), which was used to count relative optical density (ROD): A ratio of the ROD was calibrated as %, with the adult-group designated as 100%.
Every statistical analysis was carried out using GraphPad Prism 4.0 (GraphPad Software, USA). We used Kruskal-Wallis one way analysis of variance (ANOVA) on ranking test statistical significance in the number of cell, ROD and dimensions of gray matter and white matter. Data are expressed as the mean±SEM. The data were evaluated by student's t-test. Statistical significance was considered at P<0.05.
In the adult dog, α-syn immunoreactivity was easily detected in neuropil of all the laminae of the cervical, thoracic and lumbar spinal cord, and immunoreactivity was somewhat different according to the laminae; α-syn-immunoreactive profiles were mainly found in laminae I-III, and medial part of laminae X and laminae IX of motor nuclei (Figure 1A, 2A and 3A). In addition, in the thoracic level, α-syn immunoreactivity was also detected in intermediolateral nucleus in lamina VII (Figure 2A and 2b). α-Syn immunoreactivity was not detected in cell bodies and dendrites of neurons in the cervical and lumbar spinal cord (Figure 2 and 3).
In the aged group, the distribution pattern of α-syn-immunoreactive structures in the cervical and lumbar spinal cord was similar to that in the adult dog (Figure 1B and 3B), however, in the thoracic level, α-syn immunoreactivity was easily detected in the cytoplasm of many neurons, especially in laminae I-III, intermediolateral nucleus (autonomic nucleus) and motor nuclei (Figure 2B and 2f). Although immunoreactivity of α-syn was newly expressed in many neurons in the thoracic spinal cord (Figure 2B), the general pattern of α-syn immunoreactivity was not significantly changed in the cervical and lumbar spinal cord in the aged dogs compared to that in the adult group (Figure 1B and 3B).
α-Syn protein levels in the aged spinal cord were not found to be significantly changed in the cervical, thoracic and lumbar spinal cord compared to those in the adult group (Figure 4), although α-syn immunoreactivity was newly expressed in motor neurons in the aged thoracic spinal cord.
In the present study, we investigated α-syn immunoreactivity and its protein levels in the spinal cord of adult and aged beagle dogs, in order to compare the distribution and changes of α-syn.
In the adult dog, α-syn immunoreaction was distributed in neuropil throughout the gray matter of the cervical and lumbar spinal cord, and higher α-syn-immunoreactivity was detected in the laminae I-III, and medial part of laminae X and laminae IX of motor nuclei, as well as in intermediolateral nucleus (autonomic nucleus) in laminae VII in the thoracic spinal cord. This result is supported by previous studies that reported α-syn as being highly expressed in laminae I, II and X in the rat spinal cord [17], as well as in laminae IX in the C57BL/6J mouse [18,19].
In the aged dog, in the cervical and lumbar spinal cord, the expression pattern of α-syn was similar to that in the adult dog, and α-syn immunoreactivity was not significantly changed compared to that in the adult group. In addition, α-syn protein level in the aged spinal cord was not significantly changed compared to that in the adult group. Here, we report for the first time that α-syn immunoreactivity was not apparently changed in the cervical and lumbar spinal cord of the aged dog compared to that of the adult.
Interestingly, in the aged thoracic spinal cord, α-syn immunoreactivity was easily detected in the cytoplasm of many neurons, however, the expression pattern of α-syn in the aged spinal cord had a non-aggregated profile (cloud-like neuropil staining). This result is supported by a previous study that reported a grain-like structure of α-syn in neuropil without classic LB found in autonomic nuclei of the thoracic spinal cord in the aged human (44% of 304 subjects) [20]. However, it was reported that α-syn immunoreactivity was detected in the intermediolateral nucleus, with focal Lewy body (LB) and Lewy neuritis (LN) accumulation in the thoracic spinal cord of elderly subjects, which is classified as incidental Lewy body disease (ILBD) or α-syn pathology. Furthermore, it was closely associated with the involvement of a similar pathology in more upward situated regions of the central nervous system, such as the medulla oblongata, pons and hippocampus [20-22]. Therefore, it is likely that non-aggregated α-syn immunoreactivity in the aged dog spinal cord is distinctively different from pathological features such as aggregation and inclusion of α-syn, and it might be related to the non pathological profiles of α-syn during normal aging.
Microglia are one of glial cells and they play an important role in active immune defense in the CNS [23], and astrocytes, which are also one of glial cells, exert several functions such as neurotransmission and neuronal functions in the CNS [24]. In the present study, Iba-1 immunoreactivity, not GFAP immunoreactivity, was somewhat increased in the aged group compared to that in the adult group. Activated microglia secrete inflammatory mediators including reactive oxygen intermediates, nitric oxide, excitatory amino acids [25]. It was reported that gliosis can cause inflammatory responses in synucleinopathies [26-28]. In the present study, there was no significant activation of glia in the aged spinal cord, indicating that it might be related to no distinct changes of non-aggregated α-syn expression in the aged spinal cord.
In brief, α-syn immunoreactivity was not markedly changed in neuropil of the aged dog spinal cord, though Iba-1 immunoreactivity was slightly increased compared to those in the adult.
Figures and Tables
Figure 1
α-Syn immunohistochemistry in the cervical spinal cord of the adult (A, a, b and c) and aged (B, d, e and f) dogs. There are no significant differences between the adult and aged dog. Bar=500 µm (A-B), 100 µm (a-f).
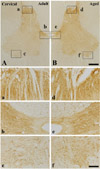
Figure 2
α-Syn immunohistochemistry in the thoracic spinal cord of the adult (A, a, b and c) and aged (B, d, e and f) dogs. α-Syn immunoreactivity was detected in the cytoplasm of spinal motor neuron in the aged dog. Bar=500 µm (A-B), 100 µm (a-f).
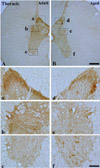
Acknowledgments
The authors would like to thank Mr. Seung Uk Lee for their technical help in this study. This work was supported by and by the Technology Innovation Program funded by the Ministry of Knowledge Economy (MKE, Korea), and by 2010 Research Grant from Kangwon National University.
References
1. Abeliovich A, Schmitz Y, Fariñas I, Choi-Lundberg D, Ho WH, Castillo PE, Shinsky N, Verdugo JM, Armanini M, Ryan A, Hynes M, Phillips H, Sulzer D, Rosenthal A. Mice lacking alpha-synuclein display functional deficits in the nigrostriatal dopamine system. Neuron. 2000. 25(1):239–252.
2. Totterdell S, Meredith GE. Localization of alpha-synuclein to identified fibers and synapses in the normal mouse brain. Neuroscience. 2005. 135(3):907–913.
3. Li JY, Henning Jensen P, Dahlström A. Differential localization of alpha-, beta- and gamma-synucleins in the rat CNS. Neuroscience. 2002. 113(2):463–478.
4. Irizarry MC, Kim TW, McNamara M, Tanzi RE, George JM, Clayton DF, Hyman BT. Characterization of the precursor protein of the non-A beta component of senile plaques (NACP) in the human central nervous system. J Neuropathol Exp Neurol. 1996. 55(8):889–895.
5. Kahle PJ, Neumann M, Ozmen L, Muller V, Jacobsen H, Schindzielorz A, Okochi M, Leimer U, van Der Putten H, Probst A, Kremmer E, Kretzschmar HA, Haass C. Subcellular localization of wild-type and Parkinson's disease-associated mutant alpha - synuclein in human and transgenic mouse brain. J Neurosci. 2000. 20(17):6365–6373.
6. Trojanowski JQ, Lee VM. Aggregation of neurofilament and alpha-synuclein proteins in Lewy bodies: implications for the pathogenesis of Parkinson disease and Lewy body dementia. Arch Neurol. 1998. 55(2):151–152.
7. Eller M, Williams DR. α-Synuclein in Parkinson disease and other neurodegenerative disorders. Clin Chem Lab Med. 2011. 49(3):403–408.
8. Rampello L, Cerasa S, Alvano A, Buttà V, Raffaele R, Vecchio I, Cavallaro T, Cimino E, Incognito T, Nicoletti F. Dementia with Lewy bodies: a review. Arch Gerontol Geriatr. 2004. 39(1):1–14.
9. Seilhean D, Takahashi J, El Hachimi KH, Fujigasaki H, Lebre AS, Biancalana V, Dürr A, Salachas F, Hogenhuis J, de Thé H, Hauw JJ, Meininger V, Brice A, Duyckaerts C. Amyotrophic lateral sclerosis with neuronal intranuclear protein inclusions. Acta Neuropathol. 2004. 108(1):81–87.
10. Wenning GK, Jellinger KA. The role of alpha-synuclein in the pathogenesis of multiple system atrophy. Acta Neuropathol. 2005. 109(2):129–140.
11. Takeda A, Hashimoto M, Mallory M, Sundsumo M, Hansen L, Sisk A, Masliah E. Abnormal distribution of the non-Abeta component of Alzheimer's disease amyloid precursor/alpha-synuclein in Lewy body disease as revealed by proteinase K and formic acid pretreatment. Lab Invest. 1998. 78(9):1169–1177.
12. Wakabayashi K, Yoshimoto M, Tsuji S, Takahashi H. Alpha-synuclein immunoreactivity in glial cytoplasmic inclusions in multiple system atrophy. Neurosci Lett. 1998. 249(2-3):180–182.
13. Kokoszka JE, Coskun P, Esposito LA, Wallace DC. Increased mitochondrial oxidative stress in the Sod2 (+/-) mouse results in the age-related decline of mitochondrial function culminating in increased apoptosis. Proc Natl Acad Sci U S A. 2001. 98(5):2278–2283.
14. Ahn JH, Choi JH, Kim JS, Lee HJ, Lee CH, Yoo KY, Hwang IK, Lee YL, Shin HC, Won MH. Comparison of immunoreactivities in 4-HNE and superoxide dismutases in the cervical and the lumbar spinal cord between adult and aged dogs. Exp Gerontol. 2011. 46(8):703–708.
15. Monti B, Contestabile A. Selective alteration of DNA fragmentation and caspase activity in the spinal cord of aged rats and effect of dietary restriction. Brain Res. 2003. 992(1):137–141.
16. Sotiriou E, Vassilatis DK, Vila M, Stefanis L. Selective noradrenergic vulnerability in α-synuclein transgenic mice. Neurobiol Aging. 2010. 31(12):2103–2114.
17. Vivacqua G, Yin JJ, Casini A, Li X, Li YH, D'Este L, Chan P, Renda TG, Yu S. Immunolocalization of alpha-synuclein in the rat spinal cord by two novel monoclonal antibodies. Neuroscience. 2009. 158(4):1478–1487.
18. Chung YH, Joo KM, Kim MJ, Cha CI. Immunohistochemical study on the distribution of alpha-synuclein in the central nervous system of transgenic mice expressing a human Cu/Zn superoxide dismutase mutation. Neurosci Lett. 2003. 342(3):151–154.
19. Vivacqua G, Casini A, Vaccaro R, Fornai F, Yu S, D'Este L. Different sub-cellular localization of alpha-synuclein in the C57BL\6J mouse's central nervous system by two novel monoclonal antibodies. J Chem Neuroanat. 2011. 41(2):97–110.
20. Oinas M, Paetau A, Myllykangas L, Notkola IL, Kalimo H, Polvikoski T. alpha-Synuclein pathology in the spinal cord autonomic nuclei associates with alpha-synuclein pathology in the brain: a population-based Vantaa 85+ study. Acta Neuropathol. 2010. 119(6):715–722.
21. Bloch A, Probst A, Bissig H, Adams H, Tolnay M. Alpha-synuclein pathology of the spinal and peripheral autonomic nervous system in neurologically unimpaired elderly subjects. Neuropathol Appl Neurobiol. 2006. 32(3):284–295.
22. Klos KJ, Ahlskog JE, Josephs KA, Apaydin H, Parisi JE, Boeve BF, DeLucia MW, Dickson DW. Alpha-synuclein pathology in the spinal cords of neurologically asymptomatic aged individuals. Neurology. 2006. 66(7):1100–1102.
23. Boje KM, Arora PK. Microglial-produced nitric oxide and reactive nitrogen oxides mediate neuronal cell death. Brain Res. 1992. 587(2):250–256.
24. Horner PJ, Palmer TD. New roles for astrocytes: the nightlife of an 'astrocyte'. La vida loca! Trends Neurosci. 2003. 26(11):597–603.
25. Vaca K, Wendt E. Divergent effects of astroglial and microglial secretions on neuron growth and survival. Exp Neurol. 1992. 118(1):62–72.
26. Croisier E, Graeber MB. Glial degeneration and reactive gliosis in alpha-synucleinopathies: the emerging concept of primary gliodegeneration. Acta Neuropathol. 2006. 112(5):517–530.
27. Klegeris A, Giasson BI, Zhang H, Maguire J, Pelech S, McGeer PL. Alpha-synuclein and its disease-causing mutants induce ICAM-1 and IL-6 in human astrocytes and astrocytoma cells. FASEB J. 2006. 20(12):2000–2008.
28. Lee HJ, Suk JE, Patrick C, Bae EJ, Cho JH, Rho S, Hwang D, Masliah E, Lee SJ. Direct transfer of alpha-synuclein from neuron to astroglia causes inflammatory responses in synucleinopathies. J Biol Chem. 2010. 285(12):9262–9272.