Abstract
Introduction
This study examined the effect of the application of low intensity pulsed ultrasound on bone healing after an injection of adipose tissue-derived stem cells (ADSCs) during the implantation of a titanium implant in the tibia of diabetes-induced rats.
Materials and Methods
Twelve Sprague-Dawely rats were used. After inducing diabetes, the ADSCs were injected into the hole for the implant. Customized screw type implants, 2.0 mm in diameter and 3.5 ㎜ in length, were implanted in both the tibia of the diabetes-induced rats. After implantation, LIPUS was applied with parameters of 3 MHz, 40 mW/cm2, and 10 minutes for 7 days to the left tibiae (experimental group) of the diabetes-induced rats. The right tibiae in each rat were used in the control group. At 1, 2 and 4 week rats were sacrificed, and the bone tissues of both tibia were harvested. The bone tissues of the three rats in each week were used for bone-to-implant contact (BIC) and bone area (BA) analyses and the bone tissues of one rat were used to make sagittal serial sections.
Results
In histomorphometric analyses, the BIC in the experimental and control group were respectively, 39.00±18.17% and 42.87±9.27% at 1 week, 43.74±6.83% and 32.27±6.00% at 2 weeks, and 32.62±11.02% and 47.10±9.77% at 4 weeks. The BA in experimental and control group were respectively, 37.28±3.68% and 31.90±2.84% at 1 week, 20.62±2.47% and 15.64±2.69% at 2 weeks, and 11.37±4.54% and 17.69±8.77% at 4 weeks. In immunohistochemistry analyses, Osteoprotegerin expression was strong at 1 and 2 weeks in the experimental group than the control group. Receptor activator of nuclear factor kB ligand expression showed similar staining at each week in the experimental and control group.
Conclusion
These results suggest that the application of low intensity pulsed ultrasound after an injection of adipose tissue-derived stem cells during the implantation of titanium implants in the tibia of diabetes-induced rats provided some positive effect on bone regeneration at the early stage after implantation. On the other hand, this method is unable to increase the level of osseointegration and bone regeneration of the implant in an uncontrolled diabetic patient.
REFERENCES
1. Misch CE. Contemporary implant dentistry. 3rd ed.Seoul: Daehan Narae Publishing;2009.
2. Min HK. Clinical characteristics of diabetes in Korea. J Korean Diabets Assoc. 1992; 16:163–74.
3. Shin CS, Kim HK, Kim WB, Park KS, Kim SY, Cho BY, et al. Incidence of diabetes mellitus in Yonchon county, Korea. J Korean Diabets Assoc. 1996; 20:264–72.
4. He H, Liu R, Desta T, Leone C, Gerstenfeld LC, Graves DT. Diabetes causes decreased osteoclastogenesis, reduced bone formation and enhanced apoptosis of osteoblastic cells in bacteria stimulated bone loss. Endocrinology. 2004; 145:447–52.


5. Santana RB, Xu L, Chase HB, Amar S, Graves DT, Trackman PC. A role for advanced glycation end products in diminished bone healing in type 1 diabetes. Diabetes. 2003; 52:1502–10.


6. Weiss RE, Gorn AH, Nimni ME. Abnormalities in the biosynthesis of cartilage and bone proteoglycans in experimental diabetes. Diabetes. 1981; 30:670–7.


7. Nevins ML, Karimbux NY, Weber HP, Giannobile WV, Fiorellini JP. Wound healing around endosseous implants in experimental diabetes. Int J Oral Maxillofac Implants. 1998; 13:620–9.


8. McCracken M, Lemons JE, Rahemtulla F, Prince CW, Feldman D. Bone response to titanium alloy implants placed in diabetic rats. Int J Oral Maxillofac Implants. 2000; 15:345–54.
9. Duarte LR. The stimulation of bone growth by ultrasound. Arch Orthop Trauma Surg. 1983; 101:153–9.


10. Yang KH, Parvizi J, Wang SJ, Lewallen DG, Kinnick RR, Greenleaf JF, et al. Exposure to low intensity ultrasound increases aggrecan gene expression in a rat femur fracture model. J Orthop Res. 1996; 14:802–9.
11. Eberson CP, Hogan KA, Moore DC, Ehrlich MG. Effect of low-intensity ultrasound stimulation on consolidation of the regenerate zone in a rat model of distraction osteogenesis. J Pediatr Orthop. 2003; 23:46–51.


12. Yang RS, Lin WL, Chen YZ, Tang CH, Huang TH, Lu BY, et al. Regulation by ultrasound treatment on the integrin expression and differentiation of osteoblasts. Bone. 2005; 36:276–83.


13. Leung KS, Cheung WH, Zhang C, Lee KM, Lo HK. Low intensity pulsed ultrasound stimulates osteogenic activity of human periosteal cells. Clin Orthop Relat Res. 2004; 418:253–9.


14. Chen YJ, Wang CJ, Yang KD, Chang PR, Huang HC, Huang YT, et al. Pertussis toxin-sensitive Galphai protein and ERK-dependent pathways mediate ultrasound promotion of osteogenic transcription in human osteoblasts. FEBS Lett. 2003; 554:154–8.
15. Tanzer M, Harvey E, Kay A, Morton P, Bobyn JD. Effect of noninvasive low intensity ultrasound on bone growth into porous-coated implants. J Orthop Res. 1996; 14:901–6.


16. Tanzer M, Kantor S, Bobyn JD. Enhancement of bone growth into porous intramedullary implants using noninvasive low intensity ultrasound. J Orthop Res. 2001; 19:195–9.


17. Dalla-Bona DA, Tanaka E, Inubushi T, Oka H, Ohta A, Okada H, et al. Cementoblast response to low- and high-intensity ultrasound. Arch Oral Biol. 2008; 53:318–23.


18. Ikai H, Tamura T, Watanabe T, Itou M, Sugaya A, Iwabuchi S, et al. Low-intensity pulsed ultrasound accelerates periodontal wound healing after flap surgery. J Periodontal Res. 2008; 43:212–6.


19. Schortinghuis J, Bronckers AL, Stegenga B, Raghoebar GM, de Bont LG. Ultrasound to stimulate early bone formation in a distraction gap: a double blind randomised clinical pilot trial in the edentulous mandible. Arch Oral Biol. 2005; 50:411–20.


20. Cowan CM, Shi YY, Aalami OO, Chou YF, Mari C, Thomas R, et al. Adipose derived adult stromal cells heal critical-size mouse calvarial defects. Nat Biotechnol. 2004; 22:560–7.
21. Dudas JR, Marra KG, Cooper GM, Penascino VM, Mooney MP, Jiang S, et al. The osteogenic potential of adipose-derived stem cells for the repair of rabbit calvarial defects. Ann Plast Surg. 2006; 56:543–8.


22. Yoon E, Dhar S, Chun DE, Gharibjanian NA, Evans GR. In vivo osteogenic potential of human adipose-derived stem cells/poly lactide-co-glycolic acid constructs for bone regeneration in a rat critical-sized calvarial defect model. Tissue Eng. 2007; 13:619–27.
23. Pittenger MF, Mackay AM, Beck SC, Jaiswal RK, Douglas R, Mosca JD, et al. Multilineage potential of adult human mesenchymal stem cells. Science. 1999; 284:143–7.


24. Hwang DS, Kim UK, Chang MC, Kim YD, Shin SH, Chung IK. Osteogenic differentiation of stem cells in a gelatin-hydroxyapatite nanocomposite. Tissue Eng Regen Med. 2009; 6:1107–13.
25. Kim MG, Jung IK, Shin SH, Kim CH, Kim BJ, Kim JH, et al. Effect of adipose-derived stem cells on bone healing on titanium implant in tibia of diabetes mellitus induced rats. J Korean Assoc Oral Maxillofac Surg. 2010; 36:392–401.


26. Marvel S, Okrasinski S, Bernacki SH, Loboa E, Dayton PA. The development and validation of a LIPUS system with preliminary observations of ultrasonic effects on human adult stem cells. IEEE Trans Ultrason Ferroelectr Freg Control. 2010; 57:1977–84.


27. Hui CF, Chan CW, Qin L, Li G, Lee KM, Hu YY, et al. Osteogenic enhancement of low intensity pulsed ultrasound on bone marrow mesenchymal stem cell. Bone. 2008; 43:78.


28. Angle SR, Sena K, Sumner DR, Virdi AS. Osteogenic differentiation of rat bone marrow stromal cells by various intensities of low-intensity pulsed ultrasound. Ultrasonics. 2011; 51:281–8.


29. Jeong SY, Shin SH, Kim UK, Park BS, Chung IK. The study of bone mineral density in the mandible of streptozotocin-induced diabetic rats. J Korean Assoc Oral Maxillofac Surg. 2002; 28:95–102.
30. Wu JR, Du GH. Temperature elevation generated by a focused Gaussian beam of ultrasound. Ultrasound Med Biol. 1990; 16:489–98.


31. Chang WH, Sun JS, Chang SP, Lin JC. Study of thermal effects of ultrasound stimulation on fracture healing. Bioelectromagnetics. 2002; 23:256–63.


32. Dyson M. Non-thermal cellular effects of ultrasound. Br J Cancer Suppl. 1982; 45:165–71.
33. Dinno MA, Dyson M, Young SR, Mortimer AJ, Hart J, Crum LA. The significance of membrane changes in the safe and effective use of therapeutic and diagnostic ultrasound. Phys Med Biol. 1989; 34:1543–52.


34. Rawool NM, Goldberg BB, Forsberg F, Winder AA, Hume E. Power Doppler assessment of vascular changes during fracture treatment with low-intensity ultrasound. J Ultrasound Med. 2003; 22:145–53.


35. Claes L, Willie B. The enhancement of bone regeneration by ultrasound. Prog Biophys Mol Biol. 2007; 93:384–98.


36. Rubin C, Bolander M, Ryaby JP, Hadjiargyrou M. The use of low-intensity ultrasound to accelerate the healing of fractures. J Bone Joint Surg Am. 2001; 83-A:259–70.


37. Sena K, Leven RM, Mazhar K, Sumner DR, Virdi AS. Early gene response to low-intensity pulsed ultrasound in rat osteoblastic cells. Ultrasound Med Biol. 2005; 31:703–8.


38. Hadjiargyrou M, McLeod K, Ryaby JP, Rubin C. Enhancement of fracture healing by low intensity ultrasound. Clin Orthop Relat Res. 1998; (355 Suppl):S216–29.


39. Tsai CL, Chang WH, Liu TK. Preliminary studies of duration and intensity of ultrasonic treatments on fracture repair. Chin J Physiol. 1992; 35:21–6.
40. Tanzer M, Harvey E, Kay A, Morton P, Bobyn JD. Effect of noninvasive low intensity ultrasound on bone growth into porous-coated implants. J Orthop Res. 1996; 14:901–6.


41. Wang FS, Kuo YR, Wang CJ, Yang KD, Chang PR, Huang YT, et al. Nitric oxide mediates ultrasound-induced hypoxia-inducible factor-1alpha activation and vascular endothelial growth factor-A expression in human osteoblasts. Bone. 2004; 35:114–23.
42. Nolte PA, van der Krans A, Patka P, Janssen IM, Ryaby JP, Albers GH. Low-intensity pulsed ultrasound in the treatment of nonunions. J Trauma. 2001; 51:693–702.


43. Korstjens CM, Nolte PA, Burger EH, Albers GH, Semeins CM, Aartman IH, et al. Stimulation of bone cell differentiation by low-intensity ultrasound-a histomorphometric in vitro study. J Orthop Res. 2004; 22:495–500.


44. Zuk PA, Zhu M, Ashjian P, De Ugarte DA, Huang JI, Mizuno H, et al. Human adipose tissue is a source of multipotent stem cells. Mol Biol Cell. 2002; 13:4279–95.


45. Lin Y, Liu L, Li Z, Qiao J, Wu L, Tang W, et al. Pluripotency potential of human adipose-derived stem cells marked with exogenous green fluorescent protein. Mol Cell Biochem. 2006; 291:1–10.


46. Hofmann S, Hagenmu¨ller H, Koch AM, Mu¨ller R, Vunjak-Novakovic G, Kaplan DL, et al. Control of in vitro tissue-engineered bone-like structures using human mesenchymal stem cells and porous silk scaffolds. Biomaterials. 2007; 28:1152–62.


47. Li X, Yao J, Wu L, Jing W, Tang W, Lin Y, et al. Osteogenic induction of adipose-derived stromal cells: not a requirement for bone formation in vivo. Artif Organs. 2010; 34:46–54.


48. Jeon O, Rhie JW, Kwon IK, Kim JH, Kim BS, Lee SH. In vivo bone formation following transplantation of human adipose-derived stromal cells that are not differentiated osteogenically. Tissue Eng Part A. 2008; 14:1285–94.


49. Kruyt MC, Dhert WJ, Oner FC, van Blitterswijk CA, Verbout AJ, de Bruijn JD. Analysis of ectopic and orthotopic bone formation in cell-based tissue-engineered constructs in goats. Biomaterials. 2007; 28:1798–805.


50. Boyce BF, Xing L. Functions of RANKL/RANK/OPG in bone modeling and remodeling. Arch Biochem Biophys. 2008; 473:139–46.


51. Pe′rez-Saya′ns M, Somoza-Martin JM, Barros-Angueira F, Rey JM, Garcl′a-Garcl′a A. RANK/RANKL/OPG role in distraction osteogenesis. Oral Surg Oral Med Oral Pathol Oral Radiol Endod. 2010; 109:679–86.
Fig. 2.
Non-decalcified section: histological aspects observed within the limits of the thread.(Villanueva stain, ×100)
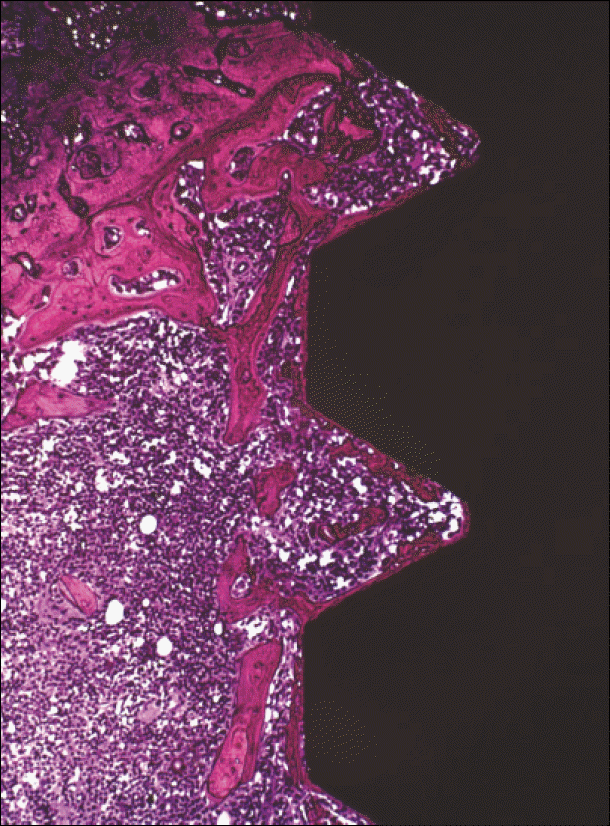
Fig. 5.
Microphotographs.(H-E stain, ×100) A. 1 week after implantation in experimental rat, B. 1 week after implantation in control rat, C. 2 weeks after implantation in experimental rat, D. 2 weeks after implantation in control rat, E. 4 weeks after implantation in experimental rat, F. 4 weeks after implantation in control rat.
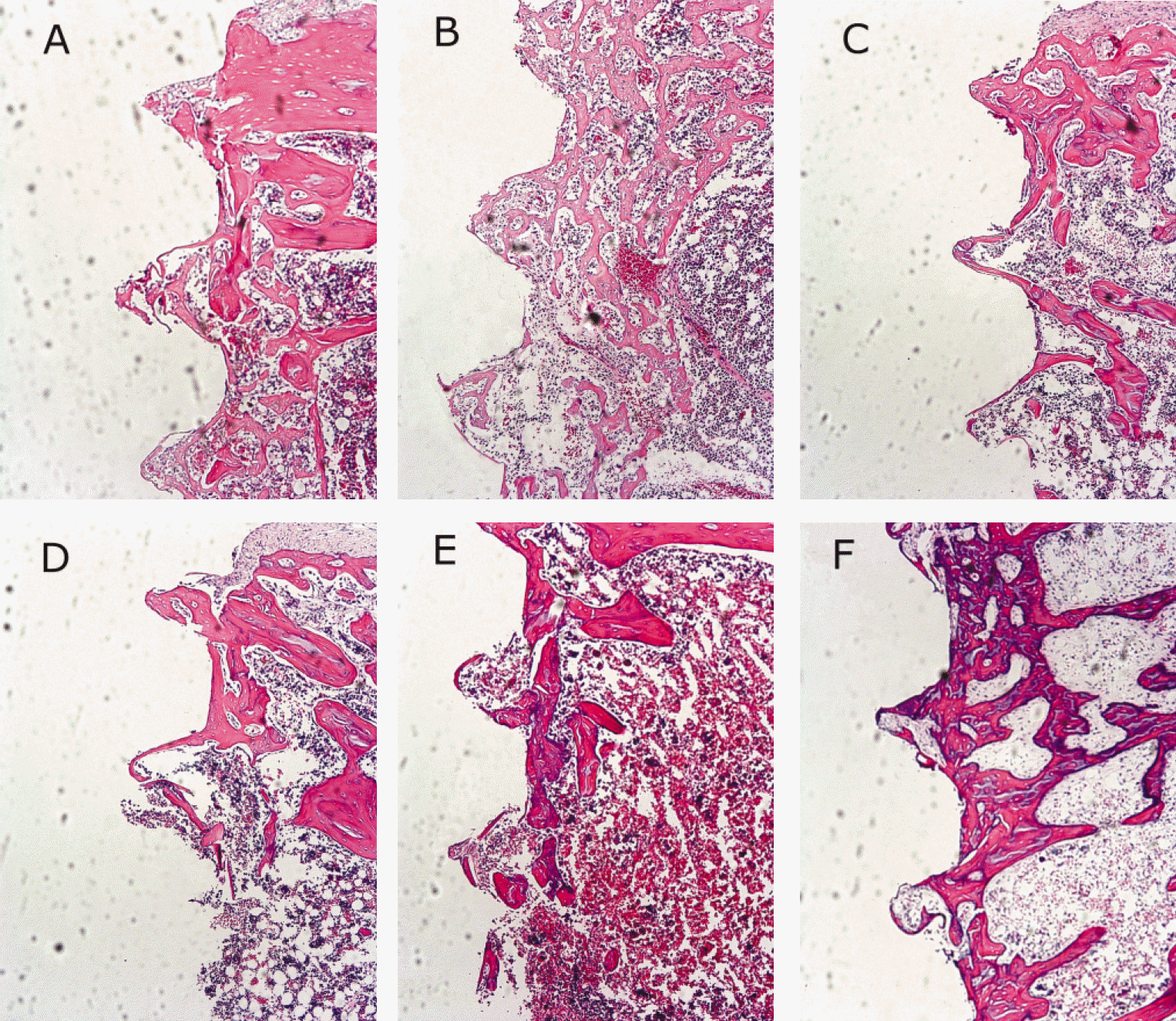
Fig. 6.
Microphotographs. (Masson's trichrome stain, ×100) A. 1 week after implantation in experimental rat, B. 1 week after implantation in control rat, C. 2 weeks after implantation in experimental rat, D. 2 weeks after implantation in control rat, E. 4 weeks after implantation in experimental rat, F. 4 weeks after implantation in control rat.
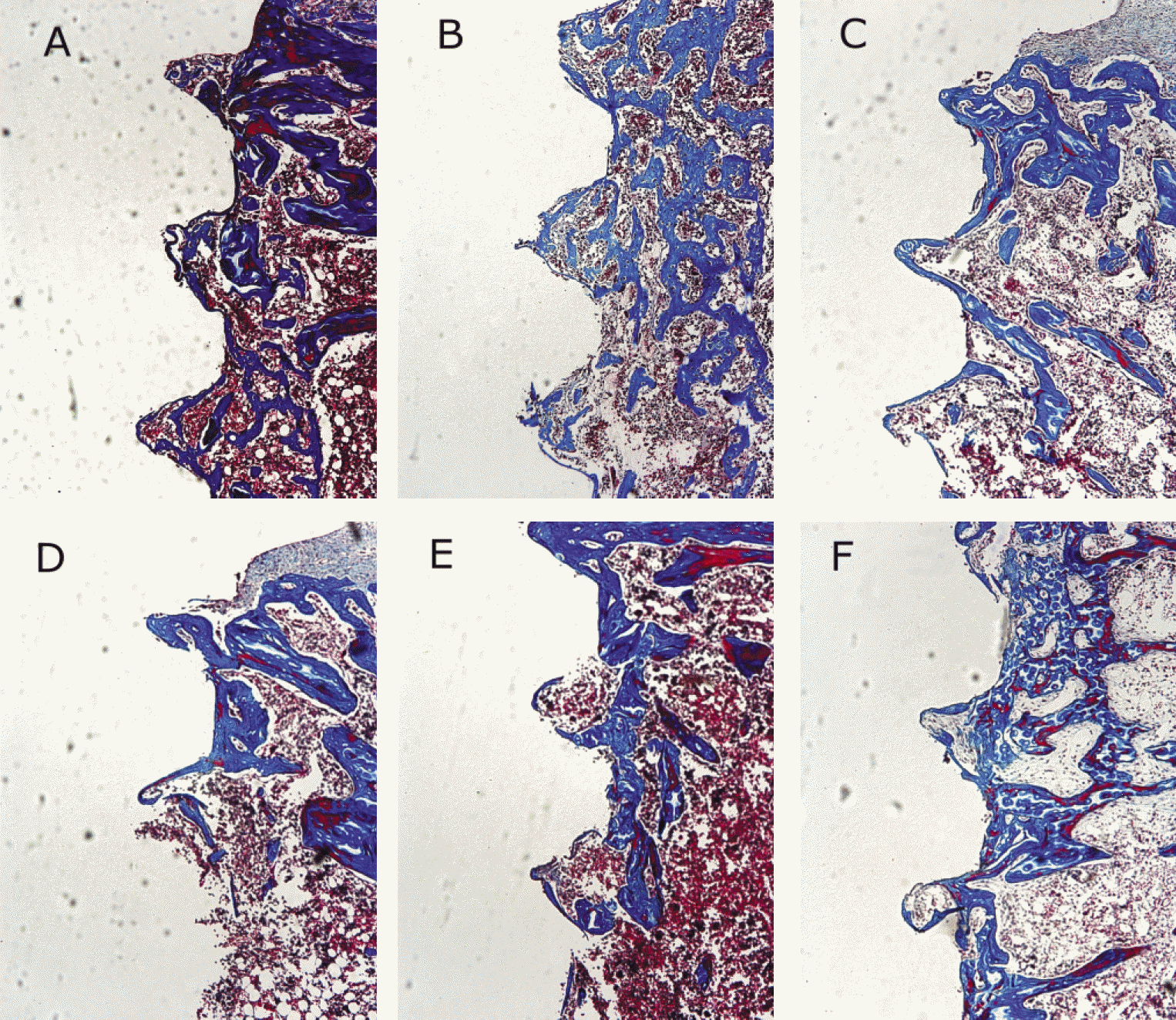
Fig. 7.
Osteoprotegerin antibody reactions. (×100) A. 1 week after implantation in experimental rat, B. 1 week after implantation in control rat, C. 2 weeks after implantation in experimental rat, D. 2 weeks after implantation in control rat, E. 4 weeks after implantation in experimental rat, F. 4 weeks after implantation in control rat.
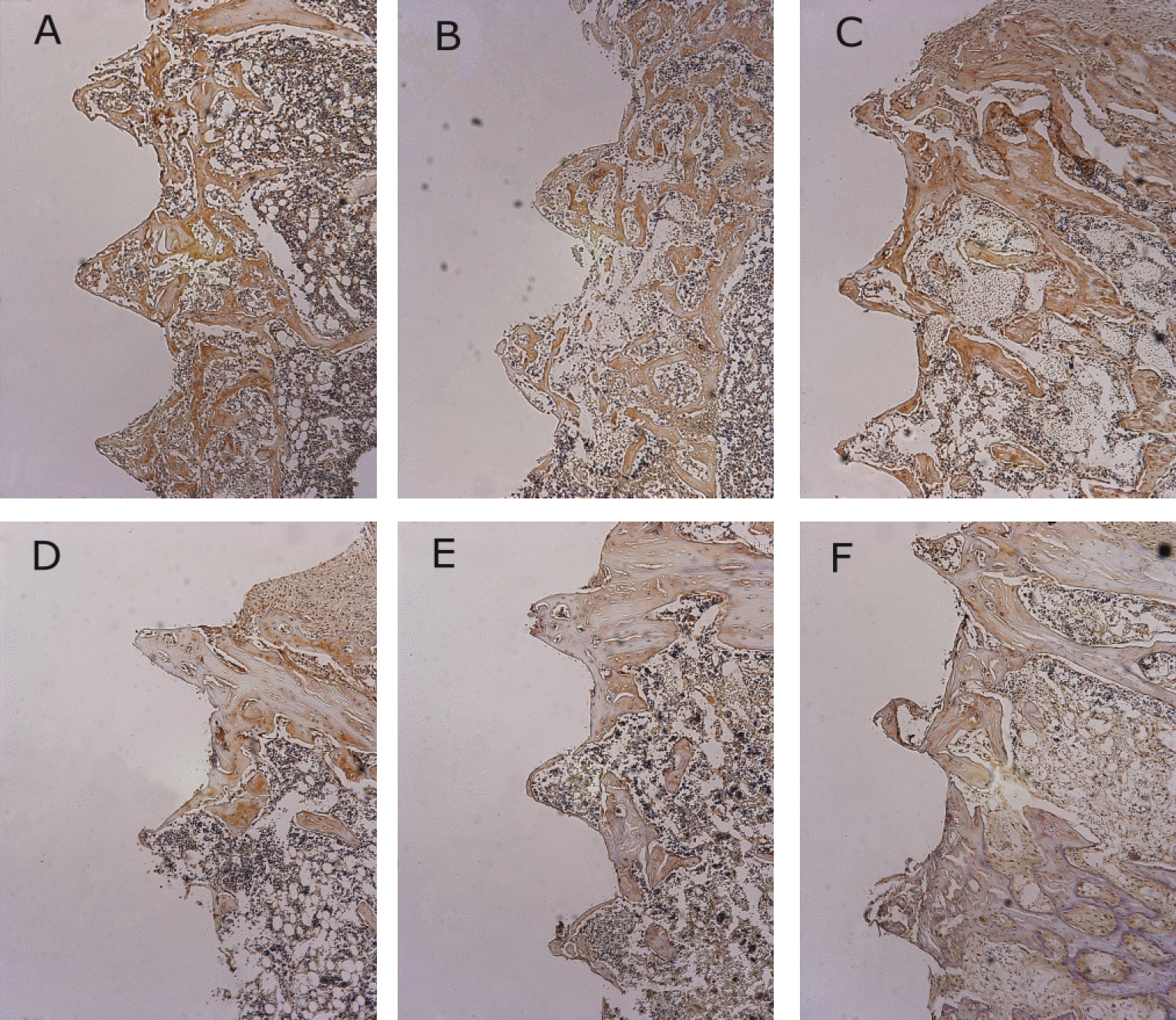
Fig. 8.
Receptor activator of nuclear factor kB ligand antibody reactions.(×100) A. 1 week after implantation in experimental rat, B. 1 week after implantation in control rat, C. 2 weeks after implantation in experimental rat, D. 2 weeks after implantation in control rat, E. 4 weeks after implantation in experimental rat, F. 4 weeks after implantation in control rat.
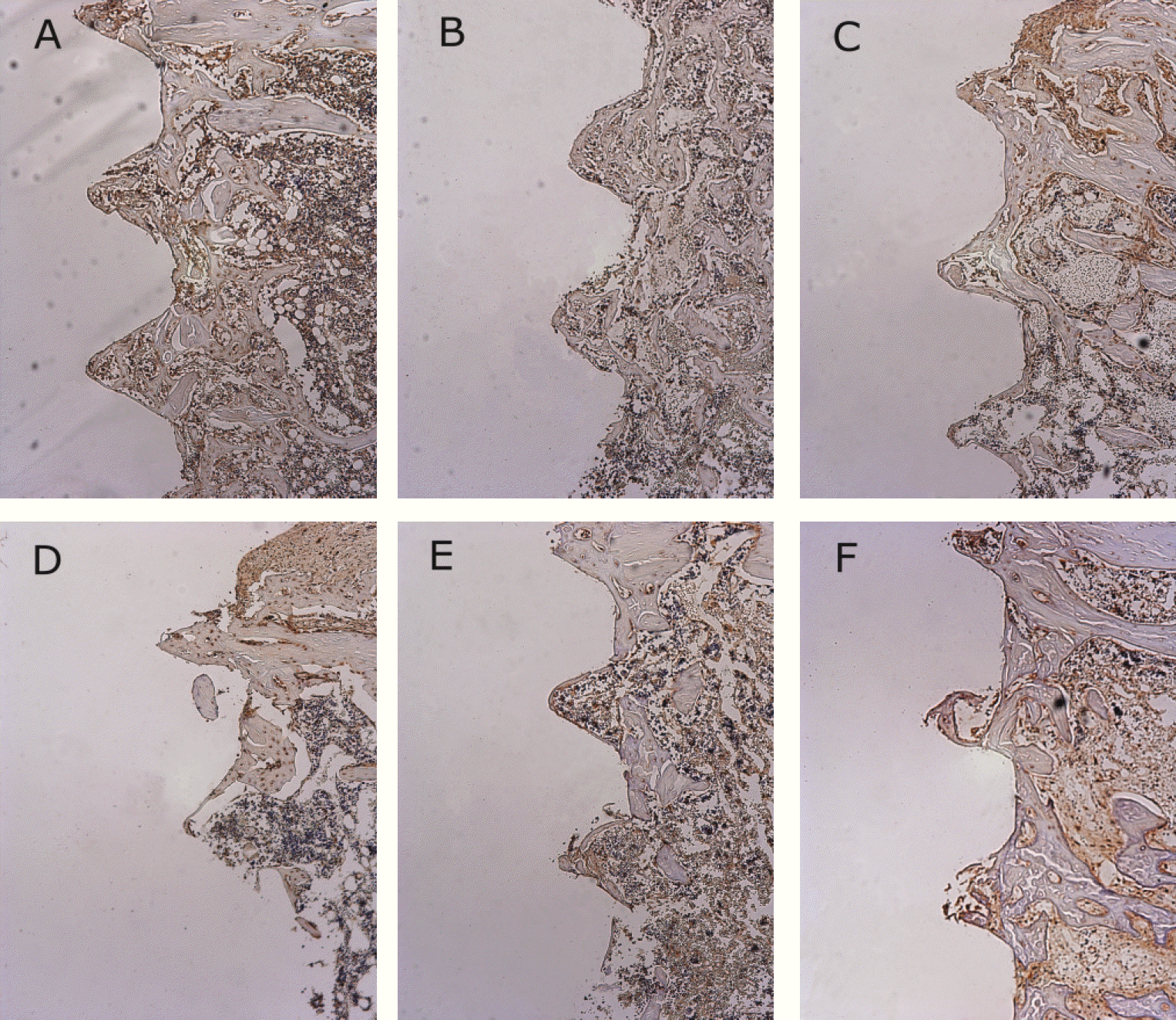
Table 1.
Mean bone-to-implant contact in experimental and control group
Weeks after implantation | Experimental (%) | Control (%) | P value |
---|---|---|---|
1 | 39.00±18.17 | 42.87±9.27 | .572 |
2 | 43.74±6.83 | 32.27±6.00 | .190 |
4 | 32.62±11.02 | 47.10±9.77 | .168 |
Table 2.
Mean bone area in experimental and controlgroup
Weeks after implantation | Experimental (%) | Control (%) | P value |
---|---|---|---|
1 | 37.28±3.68 | 31.90±2.84 | .080 |
2 | 20.62±2.47 | 15.64±2.69 | .139 |
4 | 11.37±4.54 | 17.69±8.77 | .405 |