Abstract
The purposes of this study were therefore to characterize the degeneration and regeneration of nerves to the calf muscles after selective neurectomy, both macroscopically and microscopically, and to determine the incidence of such regeneration in a rabbit model. Seventy four New Zealand white rabbits were used. Selective neurectomy to the triceps surae muscles was performed, and the muscles were subsequently harvested and weighed 1-4 months postneurectomy. The gastrocnemius muscles were stained with Sihler's solution to enable the macroscopic observation of any nerve regeneration that may have occurred subsequent to neurectomy. The change in triceps surae muscle weight was measured along the time course of the experiment. After neurectomy, nerve degeneration was followed by regeneration in all cases. The weight of the triceps surae muscle decreased dramatically between completion of the neurectomy and 1 month postneurectomy, but increased thereafter. The nerve branches were weakly stained with Sihler's solution until 2 months postneurectomy, and then strongly stained after 3 months. The number of myelinated axons was decreased at 2 month after neurectomy compared to nonneurectomized controls, but then gradually increased thereafter. Although there are currently no reports on the incidence of recovery after calf reduction, it may be a very common occurrence in the clinical field based on our findings. The findings of this study provide fundamental anatomical and surgical information to aid planning and practice in calf-reduction surgery.
The triceps surae, or calf muscle (TSM), comprises the gastrocnemius muscle (GCM) and the soleus muscle (SM). Activity of the TSM causes plantar flexion and helps in the maintenance of a standing posture. Genetic or acquired factors may thicken the GCM or make its contour uneven. Calf reduction may be desired in these cases for esthetic reasons, especially among young women. Calf reduction involves reducing the volume of the GCM, which does not cause problems in daily life, since plantar flexion and the standing posture are mainly the responsibility of the SM portion of the TSM [1].
Many methods are currently used for calf reduction. In the early days of calf-reduction surgery, subtotal resection of the GCM was performed to reduce the hypertrophic calf [234]. However, methods involving the direct removal of muscle volume have some disadvantages, including an incision scar, prolonged recovery period, high risk of damaging blood vessels, and contour irregularities [234]. Botulinum toxin A was introduced more recently as a method of reducing the volumes of both GCM and the masseter muscle. This is a somewhat effective method of reducing muscle mass, but carries with it the limitation that its effects last only about 6 months [56]. Nevertheless, it has been used consistently to produce a slender leg because of its convenience and lack of side effects.
Selective neurectomy of the tibial nerve to the GCM is currently receiving considerable attention in the field of calf reduction. The main advantage of this procedure is that it has a long-lasting effect and only minimal side effects, such as scarring. In some studies it was predicted that the muscle atrophy induced by neurectomy declines and reaches a steady state by 4 months after neurectomy [789]. However, it is highly likely that in some cases the GCM will become reinnervated by the tibial nerve and the muscle will start to regrow; peripheral nerve regeneration after injury is a well-known phenomenon in the clinical setting. However, there are currently no reports on the incidence of muscle recovery after calf reduction.
The aims of the present study were to characterize the processes of degeneration and possible subsequent regeneration of the nerves to GCM and SM after selective neurectomy in the rabbit, both macroscopically and microscopically, and to determine the incidence of regeneration after selective neurectomy.
In total, 74 New Zealand white rabbits were used for this study. Six rabbits were assigned to a control group. The mean weight of the rabbits was 2.3 kg (range, 1.9-2.6 kg). Care of the rabbits was managed according to the guidelines established by the Konkuk University Ethical Committee for Laboratory Animals. The rabbits were acclimatized to the laboratory rooms for 1 week before the surgical procedure. After the neurectomy, the rabbits were kept in animal cages according to their experimental group schedule. The TSMs were harvested from the completion of neurectomy and at particular time points from 1 to 4 months thereafter.
The rabbits were placed under general anesthesia by intramuscular injection of ketamine (50 mg/kg) and xylazine (10 mg/kg). The rabbits' skin was shaved from the thigh to the ankle, and an incision was made to expose the tibial nerve at the popliteal fossa. The branches of tibial nerve to the GCM and SM were cut about 5 mm from the branching point to its point of entry into the muscles. After the surgery, the skin incision was sutured to allow recovery. The rabbits were kept in animal cages as per the experimental group schedule. The TSMs were harvested from 1 to 4 months postoperatively, weighed, and then stained with Sihler's solution to allow the observation of nerve regeneration.
The GCMs were fixed in 10% formalin for 1 month and then macerated with 3% potassium hydroxide solution containing 0.04 ml of 3% hydrogen peroxide per 100 ml for 4 weeks. The maceration solution was changed every other day. The macerated muscles were decalcified in Sihler's solution I (one part glacial acetic acid, one part glycerin, and six parts 1% aqueous chloral hydrate) for about 3 weeks. The decalcification solution was changed once per week. The staining step for Sihler's solution II (one part Ehrlich's hematoxylin, one part glycerin, and six parts 1% aqueous chloral hydrate) lasted 2 weeks, and the destaining step (in Sihler's solution I) lasted for 6 hours. The solution was changed when it became purple. At completion of the staining process, the muscle tissue was transparent and the nerve tissue was stained purple. The stained GCMs were neutralized in 0.05% lithium carbonate solution for 1 hour and cleared in glycerin at increasing concentrations (40%, 60%, 80%, and 100%; for 1 week in each concentration). After clearing, the muscles were stored in pure glycerin in the dark.
The SMs of the control and experimental groups were used for histological study. Each SM was fixed with 10% formalin. After dehydration and paraffin infiltration, the paraffin-embedded specimens were sectioned at a thickness of 5 µm. The sections were stained with Masson's trichrome to enable the histological observation of nerve degeneration and regeneration. The stained sections were photographed using a 2048×1536-pixel digital CCD camera (DP70, Olympus, Tokyo, Japan).
Since the absolute weight of the TSM may be dependent upon the individual rabbit's body weight, the TSM index was also calculated (i.e., TSM weight/body weight). One-way ANOVA was used to compare the mean body and TSM weight changes of the rabbits and the TSM index after neurectomy. The data analysis was conducted using standard computer software (SPSS for Windows version 19.0, SPSS Inc., Chicago, IL, USA). The level of statistical significance was set at P<0.05.
The average weights of the TSM in the control and experimental groups are listed in Table 1. Since there was no statistically significant difference between the results of both sides (P>0.05), the results of the right and left sides were pooled. The weight of the TSM decreased at 1 month postneurectomy, but gradually increased thereafter up to 4 months postneurectomy. The TSM index was also lowest at 1 month postneurectomy, and gradually increased thereafter. However, unlike the weight of the TSM which was higher than control at 4 months postneurectomy, TSM index was not higher than control at 4 months (Fig. 1). There were statistically significant differences in TSM weight change between the various time periods postneurectomy in the experimental groups (P<0.05).
All nerve branches to the GCM were distinctly stained. Unlike the case in humans, the GCM of the rabbits has three heads (Fig. 2): medial, lateral, and third. The medial and lateral heads each have an independent nerve branch, while the branch to the third head arises as a bifurcation from the branch to the lateral head. Each main branch divides into small branches, which in turn divides into even smaller branches. The ramified branches were distributed perpendicularly at all levels. Finally, the fine branches innervated the GCM and were observed in Sihler's staining.
The amount of connective tissue was greater in all of the experimental groups than in the controls, with this trend increasing over the course of the experimental period. The branches of the tibial nerve to the GCM were more weakly stained in the neurectomy sides than in the normal sides (Fig. 3).
The main branches of the tibial nerve were thin, and the fine branches were not observed 1 month postneurectomy. At 2 months postneurectomy the main branches were still faintly observed, and the fine branches were still not visible. At 3 months postneurectomy the main branches were thicker than at 1 or 2 months, but the fine branches were still not visible. At 4 months postneurectomy the main branches were thick and the fine branches were faintly visible. The main branches of the tibial nerve were thinner and fainter than controls, and the fine terminal branches were not visible at all. At 1 month postneurectomy the main branches were more distinct than at 2 months, at 3 months postneurectomy the main branches had started to thicken, while the fine terminal branches did not appear at all until 4 months postneurectomy on Sihler's staining.
All samples were observed at the mid-level of the SM belly. The nerve bundles of the control group were filled with axons surrounded by myelin sheaths. The number of myelinated axons decreased after selective neurectomy, and the axons were replaced by connective tissue. The diameter of the nerve bundle was reduced in the experimental groups (Fig. 4).
The number of myelinated axons was markedly reduced at 1 month postneurectomy and further reduced at 2 months postneurectomy. In both cases the space left by the missing axons had been replaced by connective tissues. The diameter of the nerve bundle was smaller at 3 months postneurectomy than at 1 and 2 months, but the number of axons had gradually increased. At 4 months postneurectomy the nerve bundle was still small, but the number of axons was similar to that found in the controls (Fig. 4).
In summary, although the diameter of the nerve bundle was smaller at experimental group than in the control, the number of axons decreased until 2 months postneurectomy but gradually increased thereafter.
The amount of fat tissue between the muscle fibers in the TSM was not observed immediately after neurectomy, but it was observed to increase macroscopically and microscopically after 3 months postneurectomy (Fig. 5).
It is well known that neurectomy can lead to muscle atrophy, which has prompted plastic surgeons to implement selective neurectomy for esthetic purposes [78910]. Many studies have investigated this surgical protocol.
In the present study, the TSM index significantly had decreased by 1 month postneurectomy and then gradually increased thereafter. This change in muscle pattern is similar to observations made in other studies after nerve block [1112131415]. It has been reported that after neurectomy of the tibial nerve in the rat, the weight of the GCM at 8 weeks had decreased dramatically, and that this reduction in muscle mass appeared to be irreversible, although there was still a small amount of muscle left [10]. However, with the exception of a few samples, the weights of the TSMs at 4 months postneurectomy in the present study had increased from the 1-month level.
Analyses of intramuscular nerves using microdissection or computer-assisted modeling of nerve branching patterns have some limitations in that they cannot provide integral and precise pictures [1617]. Sihler's staining method is a whole-mount protocol that renders soft tissues and muscles transparent, while staining the nerves. This staining procedure has thus been used to observe the distribution of intramuscular nerves in various muscles without damaging the smaller nerve branches [1718192021]. The main advantage of Sihler's staining is that the nerve branches and networks in the muscle can be confirmed by the naked eye. Sihler's staining method was used in the present study to observe the intramuscular nerve branches to the GCM after neurectomy, allowing the nerve regeneration after neurectomy to be observed over several months without any specialized viewing equipment.
The nerves in the experimental groups were stained more faintly than in the control groups, since nerve degeneration and regeneration were in progress in those groups. The mean diameter was smaller and there were fewer regenerated myelinated axons than in intact myelinated axons [14]. Sihler's staining at 3 and 4 months postneurectomy demonstrated that the number of main branches of tibial nerves was similar to that observed in the control group, although the branches were thinner and tapered off following neurectomy. It can therefore be predicted that the regenerated nerves comprise small-diameter myelinated axons.
After neurectomy the nerve bundle enclosed within the perineurium had a smaller number of myelinated axons and was filled with connective tissue. Histological analysis revealed that connective tissues took up much of the space left by degenerated axons until 2 months postneurectomy, and the proportion of space taken up by myelinated axons began to increase and that taken up by connective tissues conversely decreased after 3 months. Although the diameter of the nerve bundle was still smaller, the inner structure of the nerve bundle at 4 months postneurectomy was similar to that in the control. Since there was more connective tissue than myelinated axons until 2 months postneurectomy, the nerve branches were barely stained, but thereafter began to stain strongly with Sihler's solution, as regeneration progressed.
de Castro Rodrigues et al. [12] demonstrated that the number of fat cells increased in the rat SM and extensor digitorum longus muscle after denervation. In the present study, fat tissue began to appear after 3 months and was observed between the muscle fibers under light-microscope observation (Fig. 5). The GCMs were stained more darkly after 3 or 4 months than after 1 or 2 months (Fig. 3). It was more difficult to macerate and destain the GCM in Sihler's solution I at 3 or 4 months. It was speculated that the fat tissues that appeared in the GCM at those time points may have affected the maceration and destaining steps. However, this increase in fat tissues may not have affected the increase in the weight of TSMs postneurectomy, since fat has smaller specific gravity than muscle.
In conclusion, the weight of the TSM began to increase after 1 month postneurectomy. The nerve branches were weakly stained with Sihler's solution until 2 months postneurectomy, and then strongly stained after 3 months. The number of myelinated axons decreased following neurectomy, but gradually increased thereafter in all of the experimental animals. These results demonstrate the occurrence of nerve regeneration after neurectomy. It can therefore be assumed that nerve regeneration will frequently occur following calf reductions performed in the field of plastic surgery; this not been reported previously in the literature. Further studies are needed to establish methods for blocking nerve regeneration to the GCM following neurectomy.
Figures and Tables
Fig. 1
Graph showing the changes in triceps surae muscle (TSM) weight after selective neurectomy. The TSM and TSM index (TSM weight relative to body weight [BW]) decreased dramatically between completion of neurectomy and 1 month postneurectomy, and started to increase thereafter.
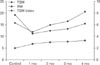
Fig. 2
Photographs of the left gastrocnemius muscle of the control group. Branches of the tibial nerve were observed with the aid of Sihler's staining. The nerve innervation of the gastrocnemius lateral and medial heads is clearly evident in the enlarged photographs.
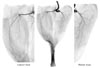
Fig. 3
Photographs of the nerve regeneration process in the experimental groups which had one-side neurectomy. The nerve branches were thinner and the nerve innervation of the muscle could not be seen after neurectomy (Sihler's stain).
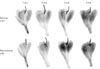
Fig. 4
(A-E) Histological changes of the nerve branch after neurectomy. The number of myelinated axons decreased, with the spaces left being filled with connective tissue in the experimental groups. Scale bars=100 µm (A-E).
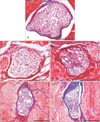
Fig. 5
(A-D) Fat cell invasion after neurectomy. The increase of fat tissues was shown in harvested triceps surae muscle (TSM) Sihler's stain and histological pictures of the 3 months group. Scale bar=500 µm (A-D).
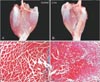
Acknowledgements
This research was supported by Basic Science Research Program through the National Research Foundation of Korea (NRF) funded by the Ministry of Education, Science and Technology (2012-0003497).
References
1. Standring S. Gray's anatomy: the anatomical basis of clinical practice. 40th ed. London: Churchill Livingstone/Elsevier;2008.
2. Kim IG, Hwang SH, Lew JM, Lee HY. Endoscope-assisted calf reduction in Orientals. Plast Reconstr Surg. 2000; 106:713–718.
3. Lee JT, Wang CH, Cheng LF, Lin CM, Huang CC, Chien SH. Subtotal resection of gastrocnemius muscles for hypertrophic muscular calves in Asians. Plast Reconstr Surg. 2006; 118:1472–1483.
4. Park YJ, Jo YW, Bang SI, Kim HJ, Lim SY, Mun GH, Hyon WS, Oh KS. Radiofrequency volume reduction of gastrocnemius muscle hypertrophy for cosmetic purposes. Aesthetic Plast Surg. 2007; 31:53–61.
5. Kim NH, Chung JH, Park RH, Park JB. The use of botulinum toxin type A in aesthetic mandibular contouring. Plast Reconstr Surg. 2005; 115:919–930.
6. Lee HJ, Lee DW, Park YH, Cha MK, Kim HS, Ha SJ. Botulinum toxin a for aesthetic contouring of enlarged medial gastrocnemius muscle. Dermatol Surg. 2004; 30:867–871.
7. Fong TH, Wong CH, Lin JY, Liao CK, Ho LY, Tsai FC. Correction of asymmetric calf hypertrophy with differential selective neurectomy. Aesthetic Plast Surg. 2010; 34:335–339.
8. Kim SC, Kang MH, Ock JJ. Calf-contouring surgery of gastrocnemius hypertrophy: selective neurectomy of the sural nerve. Aesthetic Plast Surg. 2008; 32:889–893.
9. Tsai FC, Mardini S, Fong TH, Kang JH, Chou CM. Selective neurectomy of the gastrocnemius and soleus muscles for calf hypertrophy: an anatomical study and 700 clinical cases. Plast Reconstr Surg. 2008; 122:178–187.
10. Tsai FC, Hsieh MS, Chou CM. Comparison between neurectomy and botulinum toxin A injection for denervated skeletal muscle. J Neurotrauma. 2010; 27:1509–1516.
11. Ahad MA, Fogerson PM, Rosen GD, Narayanaswami P, Rutkove SB. Electrical characteristics of rat skeletal muscle in immaturity, adulthood and after sciatic nerve injury, and their relation to muscle fiber size. Physiol Meas. 2009; 30:1415–1427.
12. de Castro Rodrigues A, Andreo JC, Rosa GM Jr, dos Santos NB, Moraes LH, Lauris JR. Fat cell invasion in long-term denervated skeletal muscle. Microsurgery. 2007; 27:664–667.
13. Gutmann E, Sanders FK. Recovery of fibre numbers and diameters in the regeneration of peripheral nerves. J Physiol. 1943; 101:489–518.
14. Irintchev A, Draguhn A, Wernig A. Reinnervation and recovery of mouse soleus muscle after long-term denervation. Neuroscience. 1990; 39:231–243.
15. Sung DH, Han TR, Park WH, Je Bang H, Kim JM, Chung SH, Woo EJ. Phenol block of peripheral nerve conduction: titrating for optimum effect. Arch Phys Med Rehabil. 2001; 82:671–676.
16. Hwang K, Kim YJ, Chung IH, Won HS, Tanaka S, Lee SI. Innervation of calf muscles in relation to calf reduction. Ann Plast Surg. 2003; 50:517–522.
17. Sheverdin VA, Hur MS, Won SY, Song WC, Hu KS, Koh KS, Kim HJ. Extra- and intramuscular nerves distributions of the triceps surae muscle as a basis for muscle resection and botulinum toxin injections. Surg Radiol Anat. 2009; 31:615–621.
18. Liu AT, Yu DZ, Chen G, Dang RS, Zhang YF, Zhang WJ, Liu BL, Jiang H. Profiling of innervations of mimetic muscles in fresh human cadavers using a modified Sihler's technique. Muscle Nerve. 2010; 42:88–94.
19. Mu L, Sanders I. Sihler's whole mount nerve staining technique: a review. Biotech Histochem. 2010; 85:19–42.
20. Won SY, Rha DW, Kim HS, Jung SH, Park ES, Hu KS, Kim HJ. Intramuscular nerve distribution pattern of the adductor longus and gracilis muscles demonstrated with Sihler staining: guidance for botulinum toxin injection. Muscle Nerve. 2012; 46:80–85.
21. Yu DZ, Liu AT, Zhang JL, Dang RS, Chen G, Liu BL, Han T, Yi J, Nagasao T, Jiang H. Two methods can simultaneously display both intramuscular nerves and blood vessels. Plast Reconstr Surg. 2012; 129:401–411.