Abstract
Cuprizone is a neurotoxin with copper-chelating ability used in animal model of multiple sclerosis in which oxidative stress has been documented as one of the cascade in the pathogenesis. Moringa oleifera is a phytomedicinal plant with antioxidant and neuroprotective properties. This study aimed at evaluating the ameliorative capability of M. oleifera in cuprizone-induced behavioral and histopathological alterations in the prefrontal cortex and hippocampus of Wistar rats. Four groups of rats were treated with normal saline, cuprizone, M. oleifera and a combination of M. oleifera and cuprizone, for five weeks. The rats were subjected to Morris water maze and Y-maze to assess long and short-term memory respectively. The animals were sacrificed, and brain tissues were removed for histochemical and enzyme lysate immunosorbent assay for catalase, superoxide dismutase, and nitric oxide. Cuprizone significantly induced oxidative and nitrosative stress coupled with memory decline and cortico-hippocampal neuronal deficits; however, administration of M. oleifera significantly reversed the neuropathological deficits induced by cuprizone.
Multiple sclerosis (MS) is an autoimmune disease characterized by neuronal demyelination, astrogliosis, and microglial activation [1]. It is generally believed that MS is an inflammatory disease controlled by T cell-induced autoimmune reaction against the myelin sheet which principally affects the white matter. However, evidences have emerged that regions of the grey matter are also significantly affected [2]. As part of brain regions affected by the condition, the frontal cortex and hippocampus have been observed to be tremendously affected, thereby impairing cognitive and memory function [3]. The presence of demyelination in the hippocampal formation of individuals with MS has also being reported, and the extent of this lesion strongly relates with progression of cognitive and memory dysfunctions seen in such patients [3]. Although MS was first described in the 18th century, the development of an efficacious therapy has always being a challenge. This is perhaps because the exact etiopathological mechanism underlying MS has remained elusive. Several animal models of MS have been developed in the last couple of decades in an attempt to critically understand the pathogenesis of the disease and ultimately develop an effective therapy. The most commonly used animal model of MS is the cuprizone (CPZ) model, a toxin-induced model of MS [4]. This is as a result of the superb copper-chelating ability of CPZ. Due to the crucial role that copper (Cu) plays in many cellular processes, its concentration is strictly regulated. Copper dyshomeostasis can result in neurodegeneration [5]. A possible mechanism of action for CPZ is via increased intracellular Cu by entrapment or depleted Cu levels from chelation [6]. Characteristic histomorphological and pathological manifestation of the CPZ-induced demyelination typically mimics type III MS lesions [1]. A 0.1% CPZ diet has very negligible effects on demyelination, whereas CPZ diet greater than 0.5% results in high mortality rate, and these dose-dependent effects are also strain-dependent [7]. The commonly used dose is between 0.2%–0.4%, but this is dependent on animal strain, age and anticipated outcome.
Oxidative stress has been implicated in the pathophysiology of CPZ model of MS [8910]. Oxidative stress is the imbalance in intracellular biochemical redox activity, ultimately impairing cellular integrity and function. The central nervous system is especially sensitive to oxidative stress because of the relatively high oxygen utilization, high polyunsaturated fat and low antioxidant level [11].
Substantial attention has been drawn to the development of efficacious therapies from phytomedicinal sources in the treatment of clinical conditions. Nigeria, India, China, and the United States are in the frontline of research channeled towards drug development from medicinal plants [12]. One of such plants that have received a lot of attention in the past two decades is Moringa oleifera. M. oleifera has been reported to have antibiotic, antitrypanosomal, hypotensive, antispasmodic, antiulcer, anti-inflammatory, hypocholesterolemic, hypoglycemic and neuroprotective activities [131415161718]. Phytochemicals present in the leaves of M. oleifera include tannins, steroids, triterpenoids, flavonoids, saponins, anthraquinones, alkaloids, and reducing sugars [19]. The essential oil of M. oleifera has been found to contain flavonoids quercetin and luteolin [20]. Quercetin contains phenolic hydroxyl groups with antioxidant action, and strongly inhibits the production of both reactive oxygen and nitrogen species [2122]. Luteolin present in M. oleifera extract has a strong antioxidant activity and confers a defensive capability on DNA [23]. Luteolin also has free radical scavenging and anti-inflammatory potentials [24]. The neuroprotective effect of M. oleifera extracts was also investigated in animal models of neurodegeneration [18]. In addition, M. oleifera leaf extract improves memory impairment and provides significant antioxidants to counteract oxidative stress in rats [25].
Much light has been shed on the demyelinating role of CPZ in animal models of demyelination with a knowledge gap in deficits in neuronal function in the cortico-hippocampal morphology and memory decline associated with CPZ use. The aim of this study was to evaluate the anti-oxidant effects of M. oleifera during experimentally-induced demyelination by CPZ in the frontal cortex and hippocampus of Wistar rats.
Twenty-four adult Wistar rats of average weight 120±3.2 g were obtained from Bolaji Enterprise, Ilorin and kept in the Animal House of Faculty of Basic Medical Sciences, University of Ilorin following ethical considerations. The rats were maintained on normal rodent feeds procured from Ogo-Oluwa Livestock Enterprises, Ilorin. Feeds and water were provided liberally.
CPZ was purchased from Sigma-Aldrich, Hamburg, Germany. CPZ was prepared with the feeds to obtain 0.4% CPZ. M. oleifera plant was obtained and ethanolic extraction of the plant was carried out using column chromatography Fractionation method at the Department of Chemistry, University of Ilorin, Nigeria. The fraction MoF6 was used in the present study to evaluate the toxicity effects of M. oleifera leaves on adult female Wistar rats.
The rats were categorized into four groups. Group A (control) received 1 ml normal saline, group B (CPZ) received 0.4% CPZ diet, group C (M. oleifera) received 1.875 mg/ml M. oleifera, while group D (CPZ+M. oleifera) received a combination of CPZ diet (0.4%) and M. oleifera (1.875 mg/ml). The mode of administration was oral, for duration of 5 weeks.
At the end of administration of M. oleifera and CPZ, the rats were tested in the Morris water maze and Y-maze to assess spatial memory and working memory respectively.
This test was carried out to assess the spatial learning and memory of the rats. A pool of water measuring about 100 cm in diameter and 30 cm in depth was used. An escape platform about an inch deep from the surface of the water was placed in one of the quadrants outside of which was a visual cue. The animals were trained 24 hours prior to the actual test. During the training, each rat was placed in each of the other three quadrants for a maximum period of 60 seconds to find the escape platform at intervals of 15 minutes between quadrants until the escape latency period reduced to less than 15 seconds. During the test, the pool was colored and the animals were placed in each of the three quadrants different from the escape platform quadrant at an interval of 15 minutes between quadrants. The time taken to find the escape platform was recorded as the escape latency period.
This test was used to examine the working memory of the rats. The animals were placed in a Y-maze whose arms measured 75 cm in length and 15 cm in breadth with an angle of 120° between the arms. The animals were allowed to explore the maze for a duration of 5 minutes. The manner of arm entries was recorded. A correct alternation is scored when the animal successfully explored each of the three arms of the maze per triad of exploration (e.g., ABC, CAB, or BCA). Once two arms were explored per triad of exploration (e.g., ABA, CAC, BAB), it was considered an incorrect alternation. The percentage correct alternation of each rat was estimated as a ratio of the correct alternation to the total alternation multiplied by 100.
After the conduct of the behavioral assessment, the rats were subjected to cervical dislocation, and the brain tissues were immediately excised and dissected into two hemispheres. All the right hemispheres were fixed in 4% paraformaldehyde for histochemical processing, while the left hemispheres were rinsed three times in 0.25 M sucrose for five minutes and stored in 30% sucrose at 4℃. Coronal sections of the prefrontal cortex (PFC) and hippocampus were obtained stereotaxically. Subsequently, these two parts of the brain were processed to obtain paraffin wax embedded block for Cresyl fast violet demonstration. Tissues stored in 30% sucrose were dissected on ice to obtain PFC and hippocampus. The homogenate of these tissues was obtained and then centrifuged at 12,000 rpm to obtain the supernatant containing tissue lysates. The supernatants were obtained and stored at very low temperatures. Superoxide dismutase (SOD), catalase (CAT), and nitric oxide (NO) activities in the tissue lysates were assayed using appropriate enzyme lysate immunosorbent assay kits.
Photomicrographs of slides obtained from histochemical
processing of tissues were captured using Olympus (Tokyo, Japan) binocular microscope which was connected to a 5.0 megapixel Amscope camera (Amscope Inc., Irvine, CA, USA). Data obtained from enzyme analysis was subjected to statistical analysis using the graph pad prism software (version 5, GraphPad Inc., La Jolla, CA, USA). The results of SOD, CAT, and NO were plotted as bar graphs with error bars (mean and standard error of mean). One-way ANOVA with Tukey's multiple comparison test was done. Significant value was set at P<0.05, P<0.01, and P<0.005.
In the present study, animals were subjected to Morris water maze and Y-maze test to assay for spatial memory and working memory respectively (Fig. 1). The Y-maze test is built on the natural tendency of rats to explore a maze by systematically entering one arm to another without returning to an arm it recently explored. This is also used to assay for short term memory. There was no significant difference in the total number of arm entries in the Y maze (P>0.05) across the experimental groups, suggesting that there was no difference in the exploratory drive of the experimental animals (Table 1). However, CPZ induced a significant reduction in the working memory of the experimental animal as it was observed that the CPZ treated animal presented with significantly reduced percentage correct alternation. This finding suggests that CPZ adversely affects short-term memory potentiation. M. oleifera was able to restore the working memory of the experimental animal as we observed an increase in the percentage correct alternation of animals that were treated with both CPZ and M. oleifera. Correspondingly, in the Morris water maze, which was used to assess long-term memory, CPZ induced a significant increase in the escape latency period of animals treated with CPZ only. M. oleifera was able to restore long-term memory of the animals that were co-treated with both CPZ and M. oleifera. This finding suggests that M. oleifera through a series of chemical events is able to counteract and restore the depletion in both short-term and long-term memory induced by CPZ.
In the present study we assayed for SOD, CAT, and NO activities in the PFC and hippocampus of experimental animals (Fig. 2). SOD and CAT are the first line of defense against reactive oxygen species (ROS). CPZ caused a significant reduction in SOD activity in both PFC and hippocampus. This suggests that cuprizone induced excessive generation of superoxide which cannot be mopped up by the intrinsically produced SOD. This deficit in SOD activity could result in superfluous reactive oxygen species. Similarly, CAT activity was also significantly reduced in the PFC and hippocampus of CPZ-treated rats suggesting the role that CPZ plays in reducing oxidative stress. CPZ also induced excessive production of reactive nitrogen species as we observed a significant increase in nitric oxide activities in both the PFC and hippocampus. M. oleifera was able to significantly normalize the activities of SOD and CAT in the PFC and hippocampus of experimental animals. This was deduced from the significantly elevated levels of SOD and CAT in the PFC and hippocampus of animals treated with both CPZ and M. oleifera relative to those treated with CPZ only. In like manner, M. oleifera was able to deplete the excessively generated ROS by the actions of CPZ. These findings suggest that M. oleifera prevents oxidative and nitrosative stress induced by CPZ.
The cresyl fast violet stain was used to study neuronal morphology based on the affinity of neuronal cells for this histochemical stain (Fig. 3). The histochemical demonstration in the present study revealed the specific mode and mechanisms that substantiates the behavioral and biochemical findings. Animals in the control group and M. oleifera group did not show any pathological alteration in the morphological appearance of the pyramidal cells of the hippocampus and prefrontal cortex. These cells were aptly stained and properly situated within their neuropils. In the two aforementioned groups, the arrangement of the pyramidal neurons of the cornus ammonis (CA) of the dentate gyrus was generally normal and structurally well defined. The apical and basal dendrites were well expressed and interconnected within the neuropils of both groups. There was proper delineation and characteristically normal cytoarchitectural assortment of the pyramidal cells within the external pyramidal layer of the PFC. CPZ-treated rats showed several degenerative changes within the PFC and hippocampal sections. In this group, the hippocampal and cortical sections presented with histoarchitectural distortion across neuropil. There was observed extensive central chromatolytic and pyknotic changes in the pyramidal cells of the CA region and external pyramidal layer of the hippocampus and PFC respectively. Rats that were treated with both CPZ and M. oleifera showed marked improvement in the morphological and histoarchitectural appearance compared to rats that received CPZ only, although we observed a mild degenerative changes characterized by transient chromatolysis of a few pyramidal cells, cellular assortment across the hippocampus and PFC were better delineated and assorted compared to CPZ group. It was also observed that M. oleifera treatment showed antiproteolytic potentials by preventing Nissl bodies degradation induced by CPZ as revealed by the increased staining intensity of the pyramidal neurons in the cortex of rats exposed to CPZ and M. oleifera. In summary, histochemical demonstration of corticohippocampal sections revealed that M. oleifera is able to sustain neuronal integrity against CPZ assault in the PFC and hippocampus of Wistar rats.
The ameliorative effects of orally administered M. oleifera on CPZ-induced oxidative and nitrosative stress and memory decline have been demonstrated in this study. From our findings, CPZ adequately induced oxidative and nitrosative stress in the PFC and hippocampus of experimental animals which manifested in the histomorphology of these important brain regions and translated to the neurobehavioral outcome of these animals. M. oleifera intervention restored the perturbed biochemical redox imbalance, neuronal integrity within the frontal cortex and hippocampus and ultimately long-term and short-term memory of experimental animals.
Evidences in recent times have suggested that oxidative stress plays a crucial role in the pathogenesis of MS [126]. Our study revealed that CPZ induced oxidative stress as we noted a reduction in the activities of SOD and CAT in the PFC and hippocampus of Wistar rats treated with CPZ. This points out that there is a superfluous generation of ROS (superoxide and hydrogen peroxide) by the actions of CPZ that cannot be catered for by the intrinsic antioxidant system in the aforementioned brain regions. SOD and CAT are important enzymes critical in the antioxidant system. SOD is responsible for breaking down highly reactive superoxide into a less reactive hydrogen peroxide. Hydrogen peroxide is further disintegrated into water molecule and oxygen by the action of CAT. Exacerbating the level of superoxide and hydrogen peroxide will eventually downplay the activities of SOD and CAT, respectively, thereby resulting into oxidative stress. Oxidative stress leads to mitochondrial dysfunction which is one of the prominent features in MS lesions [27]. It has been postulated that CPZ is a neurotoxin that triggers cell death in neuronal and non-neuronal cells especially oligodendrocytes. CPZ-induced oxidative stress in the present study was coupled with nitrosative stress as it was noted that animals maintained on CPZ diets for 5 weeks had exacerbated levels of NO. NO is a crucial cellular signaling molecule that has been reported to have a bidirectional role with favorable and cytotoxic action subject to its level in the body [28]. Increased NO levels single-handedly or in combination with ROS produced deleterious effects such as apoptosis associated neurodegenerative processes. Most neurodegenerative disorders are marked by the presence of intracellular accumulation of protein aggregates which are nitrotyrosine-positive [29]. Cumulatively, CPZ induced oxidative and nitrosative stress in the present study, consistent with several other findings in literature [273031].
We further demonstrated that M. oleifera significantly restored the perturbation of the cellular redox balance in the frontal cortex and hippocampus. M. oleifera treatment normalized the level of SOD, CAT, and NO by two proposed mechanisms. Firstly, M. oleifera induced a cascade of chemical reaction that helped in boosting the intrinsically produced superoxide and CAT thereby strengthening the antioxidant system to cater for the exacerbated ROS and nitrogen species. Secondly, it acts as an antioxidant in its own capacity, thereby helping the intrinsic antioxidant system to mop up the excessively generated reactive species. Phytochemicals present in M. oleifera that give it its antioxidant potential include quercetin and luteoline [1520].
Our findings from the neurobehavioral test in the present study revealed that CPZ treatment significantly depleted both long and short-term memory. As a measure of long-term memory, animals were subjected to Morris water maze which is a stress induced behavioral test. Animals maintained on CPZ diets for 5 weeks had an increased escape latency period which is a marker for long term memory. Likewise, in the spontaneous alternation test which was used to assess short-term memory, these animals presented with decreased percentage correct alternation. This observed depletion in the memory of experimental animals suggests the possible negative effect CPZ has on the cortico-hippocampal region responsible for memory formation and long-term potentiation. Previous studies of MS patients have linked hippocampal demyelination to progressive cognitive and memory dysfunction [30]. M. oleifera treatment after 5 weeks of CPZ diet restored the long and short-term memory in experimental animals, and this is consistent with the report that the leaf extract of M. oleifera improves memory impairment in Wistar rats [25].
Histochemical findings in this study support previous reports that CPZ extensively damages hippocampal neurons [3233]. Nissl stain findings in the present study revealed that CPZ compromised the integrity of neuronal cells within the PFC and hippocampus of experimental animals. Neurons in these two brain regions showed early signs of pyknosis, characterized by extreme central chromatolysis, intercellular fragmentation, axonal degeneration (following demyelination), and cytoplasmic condensation. Axons regulate a number of intricate operations within the nervous system that not only control signal processing in brain circuits but also neuronal synaptic efficiency [34]. This kind of compromise is consistent with the neurobehavioral outcome of these animals in the present study. CPZ compromises cellular morphology through oxidative damage to the cells by raising levels of neurotoxin such as superoxide which are capable of damaging the nuclear component of the cell. M. oleifera restored cellular integrity within the hippocampus and frontal cortex of experimental animals by preventing cell death and fast-tracking re-myelination. M. oleifera has been reported to have neuroprotective [1518], and the observed therapeutic potential in the current work appears to be by enhancing the antioxidant defense system.
This study identified CPZ as a neurotoxin capable of inducing both oxidative and nitrosative stress. It also revealed the therapeutic potential of M. oleifera against CPZ-induced behavioral and neuropathological deficits in the PFC and hippocampus of Wistar rat.
References
1. Lucchinetti C, Bruck W, Parisi J, Scheithauer B, Rodriguez M, Lassmann H. Heterogeneity of multiple sclerosis lesions: implications for the pathogenesis of demyelination. Ann Neurol. 2000; 47:707–717. PMID: 10852536.
2. Geurts JJ, Barkhof F. Grey matter pathology in multiple sclerosis. Lancet Neurol. 2008; 7:841–851. PMID: 18703006.
3. Norkute A, Hieble A, Braun A, Johann S, Clarner T, Baumgartner W, Beyer C, Kipp M. Cuprizone treatment induces demyelination and astrocytosis in the mouse hippocampus. J Neurosci Res. 2009; 87:1343–1355. PMID: 19021291.
4. Praet J, Guglielmetti C, Berneman Z, Van der Linden A, Ponsaerts P. Cellular and molecular neuropathology of the cuprizone mouse model: clinical relevance for multiple sclerosis. Neurosci Biobehav Rev. 2014; 47:485–505. PMID: 25445182.
5. Manto M. Abnormal copper homeostasis: mechanisms and roles in neurodegeneration. Toxics. 2014; 2:327–345.
6. Skripuletz T, Bussmann JH, Gudi V, Koutsoudaki PN, Pul R, Moharregh-Khiabani D, Lindner M, Stangel M. Cerebellar cortical demyelination in the murine cuprizone model. Brain Pathol. 2010; 20:301–312. PMID: 19371354.
7. Skripuletz T, Lindner M, Kotsiari A, Garde N, Fokuhl J, Linsmeier F, Trebst C, Stangel M. Cortical demyelination is prominent in the murine cuprizone model and is strain-dependent. Am J Pathol. 2008; 172:1053–1061. PMID: 18349131.
8. Draheim T, Liessem A, Scheld M, Wilms F, Weissflog M, Denecke B, Kensler TW, Zendedel A, Beyer C, Kipp M, Wruck CJ, Fragoulis A, Clarner T. Activation of the astrocytic Nrf2/ARE system ameliorates the formation of demyelinating lesions in a multiple sclerosis animal model. Glia. 2016; 64:2219–2230. PMID: 27641725.
9. Suneetha A, Raja Rajeswari K. Role of dimethyl fumarate in oxidative stress of multiple sclerosis: a review. J Chromatogr B Analyt Technol Biomed Life Sci. 2016; 1019:15–20.
10. Sajjadian M, Kashani IR, Pasbakhsh P, Hassani M, Omidi A, Takzare N, Clarner T, Beyer C, Zendedel A. Protective effects of cannabidiol on cuprizone-induced demyelination in C57BL/6 mice. J Contemp Med Sci. 2017; 3:278–283.
11. Carvalho AN, Lim JL, Nijland PG, Witte ME, Van Horssen J. Glutathione in multiple sclerosis: more than just an antioxidant? Mult Scler. 2014; 20:1425–1431. PMID: 24842957.
12. World Health Organization. Traditional medicine strategy 2002-2005. Geneva: World Health Organization;2002.
13. Eilert U, Wolters B, Nahrstedt A. The antibiotic principle of seeds of Moringa oleifera and Moringa stenopetala. Planta Med. 1981; 42:55–61. PMID: 7255568.
14. Ezeamuzie IC, Ambakederemo AW, Shode FO, Ekwebelem SC. Antiinflammatory effects of Moringa oleifera root extract. Int J Pharmacogn. 1996; 34:207–212.
15. Gbadamosi IT, Omotoso GO, Olajide OJ, Dada-Habeeb SO, Arogundade TT, Lambe E, Obasi KK. Moringa protects against nicotine-induced morphological and oxidative damage in the frontal cortex of Wistar rats. Anatomy. 2016; 10:170–176.
16. Sreelatha S, Jeyachitra A, Padma PR. Antiproliferation and induction of apoptosis by Moringa oleifera leaf extract on human cancer cells. Food Chem Toxicol. 2011; 49:1270–1275. PMID: 21385597.
17. Ferreira RS, Napoleão TH, Santos AF, Sá RA, Carneiro-da-Cunha MG, Morais MM, Silva-Lucca RA, Oliva ML, Coelho LC, Paiva PM. Coagulant and antibacterial activities of the watersoluble seed lectin from Moringa oleifera. Lett Appl Microbiol. 2011; 53:186–192. PMID: 21605145.
18. Libro R, Giacoppo S, Soundara Rajan T, Bramanti P, Mazzon E. Natural phytochemicals in the treatment and prevention of dementia: an overview. Molecules. 2016; 21:518. PMID: 27110749.
19. Kasolo JN, Bimenya GS, Ojok L, Ochieng J, Ogwal-okeng JW. Phytochemicals and uses of Moringa oleifera leaves in Ugandan rural communities. J Med Plants Res. 2010; 4:753–757.
20. Pari L, Karamać M, Kosińska A, Rybarczyk A, Amarowicz R. Antioxidant activity of the crude extracts of drumstick tree (Moringa oleifera Lam.) and sweet broomweed (Scoparia dulcis L.) leaves. Pol J Food Nutr Sci. 2007; 57:203–208.
21. Pace-Asciak CR, Hahn S, Diamandis EP, Soleas G, Goldberg DM. The red wine phenolics trans-resveratrol and quercetin block human platelet aggregation and eicosanoid synthesis: implications for protection against coronary heart disease. Clin Chim Acta. 1995; 235:207–219. PMID: 7554275.
22. Kawada N, Seki S, Inoue M, Kuroki T. Effect of antioxidants, resveratrol, quercetin, and N-acetylcysteine, on the functions of cultured rat hepatic stellate cells and Kupffer cells. Hepatology. 1998; 27:1265–1274. PMID: 9581680.
23. Romanová D, Vachálková A, Cipák L, Ovesná Z, Rauko P. Study of antioxidant effect of apigenin, luteolin and quercetin by DNA protective method. Neoplasma. 2001; 48:104–107. PMID: 11478688.
24. El-Hawary SS, El-Sofany RH, Abdel-Monem AR, Ashour RS, Sleem AA. Polyphenolics content and biological activity of Plectranthus amboinicus (Lour.) spreng growing in Egypt (Lamiaceae). Pharmacogn J. 2012; 2:45–54.
25. Ganguly R, Hazra R, Ray K, Guha D. Effect of Moringa oleifera in experimental model of Alzheimer's disease: role of antioxidants. Ann Neurosci. 2005; 12:33–36.
26. Lassmann H. What drives disease in multiple sclerosis: inflammation or neurodegeneration? Clin Exp Neuroimmunol. 2010; 1:2–11.
27. Lassmann H. Pathology and disease mechanisms in different stages of multiple sclerosis. J Neurol Sci. 2013; 333:1–4. PMID: 23735777.
28. Keynes RG, Garthwaite J. Nitric oxide and its role in ischaemic brain injury. Curr Mol Med. 2004; 4:179–191. PMID: 15032712.
29. Duda JE, Giasson BI, Chen Q, Gur TL, Hurtig HI, Stern MB, Gollomp SM, Ischiropoulos H, Lee VM, Trojanowski JQ. Widespread nitration of pathological inclusions in neurodegenerative synucleinopathies. Am J Pathol. 2000; 157:1439–1445. PMID: 11073803.
30. Acs P, Kipp M, Norkute A, Johann S, Clarner T, Braun A, Berente Z, Komoly S, Beyer C. 17beta-estradiol and progesterone prevent cuprizone provoked demyelination of corpus callosum in male mice. Glia. 2009; 57:807–814. PMID: 19031445.
31. Kashani IR, Rajabi Z, Akbari M, Hassanzadeh G, Mohseni A, Eramsadati MK, Rafiee K, Beyer C, Kipp M, Zendedel A. Protective effects of melatonin against mitochondrial injury in a mouse model of multiple sclerosis. Exp Brain Res. 2014; 232:2835–2846. PMID: 24798398.
32. Abe H, Saito F, Tanaka T, Mizukami S, Hasegawa-Baba Y, Imatanaka N, Akahori Y, Yoshida T, Shibutani M. Developmental cuprizone exposure impairs oligodendrocyte lineages differentially in cortical and white matter tissues and suppresses glutamatergic neurogenesis signals and synaptic plasticity in the hippocampal dentate gyrus of rats. Toxicol Appl Pharmacol. 2016; 290:10–20. PMID: 26577399.
33. Nathoo N, Yong VW, Dunn JF. Understanding disease processes in multiple sclerosis through magnetic resonance imaging studies in animal models. Neuroimage Clin. 2014; 4:743–756. PMID: 24936425.
34. Debanne D, Campanac E, Bialowas A, Carlier E, Alcaraz G. Axon physiology. Physiol Rev. 2011; 91:555–602. PMID: 21527732.
Fig. 1
Percentage correct alternation (A) and escape latency period (B) in the Y-maze and Morris water maze test respectively. CPZ, cuprizone; MOR, Moringa oleifera. There was a significant reduction in the percentage correct alternation of animals treated with CPZ relative to control and MOR (P<0.05 for both). There was no significant difference in the percentage correct alternation in control animals and MOR treated animals. Animals concomitantly treated with CPZ and MOR presented with a percentage correct alternation higher than that of the CPZ treated animals (P>0.05) and lower than MOR and control treated animals (P>0.05). The escape latency period of CPZ animals was significantly higher than the control, MOR and CPZ+MOR treated animals (P<0.01 for all three). There was no difference in the escape latency period of control, MOR, and CPZ+MOR (P>0.05). *P<0.05, **P<0.01.
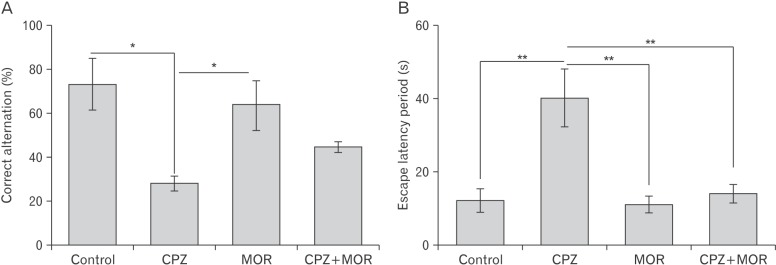
Fig. 2
(A–F) Catalase activity in the hippocampus (A) and prefrontal cortex (B), as well as nitric oxide activities in the hippocampus (C) and prefrontal cortex (D). CPZ, cuprizone; MOR, Moringa oleifera; PFC, prefrontal cortex; SOD, superoxide dismutase. There was a significant reduction in catalase activities in the hippocampus of CPZ treated animals relative to control (p<0.05), MOR (p<0.01), and CPZ+MOR (p<0.05). There is no significant difference in the hippocampal catalase level of control, MOR and CPZ+MOR (P>0.05). Similarly, catalase activities reduced in the prefrontal cortex of CPZ treated animals relative to the control and MOR groups (P<0.01). There was no significant difference in the cortical catalase activity of the control, MOR and CPZ+MOR (P>0.05). Nitric oxide activity increased in the hippocampus of CPZ treated animals relative to all other groups (P<0.01). There was no significant difference in the nitric oxide activities of control, MOR, and CPZ+MOR (P>0.05). Nitric oxide activity also increased in the prefrontal cortex of CPZ treated animals relative to control (P<0.01), MOR (P<0.005) and CPZ+MOR (P>0.05). There was a significant difference in the nitric oxide activities of, MOR group relative to control (P<0.01) and CPZ+MOR (P>0.005). *P<0.05, **P<0.01, ***P<0.005.
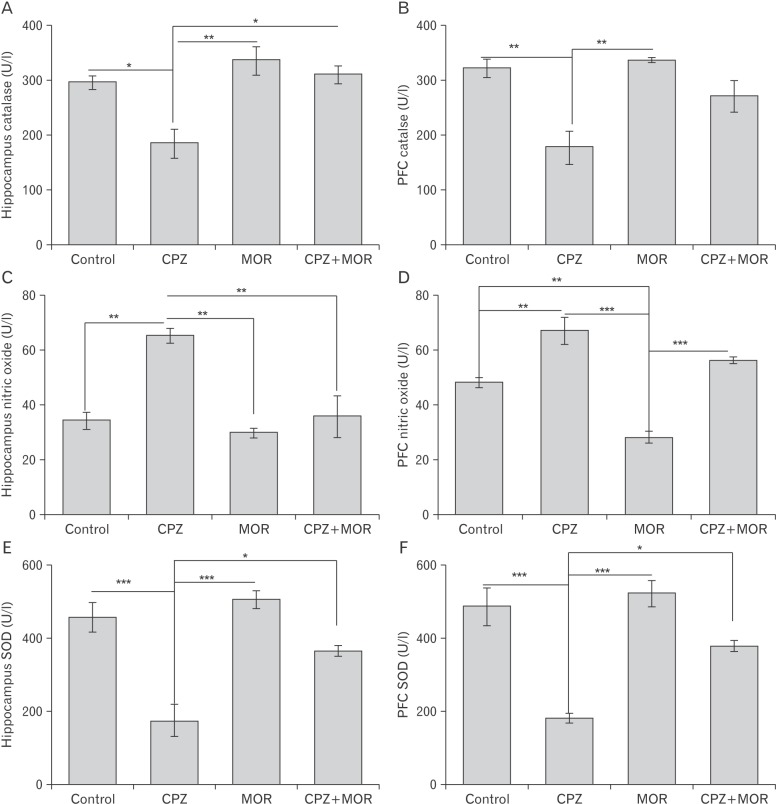
Fig. 3
Representative photomicrograph of the hippocampus and prefrontal cortex of experimental animals showing the pyramidal cells of the conus amonus three (CA3) region and external pyramidal layer respectively. CPZ, cuprizone; MOR, Moringa oleifera. The histomorphological presentation of the CA3 region of the hippocampus of control and MOR treated rats showed characteristically large pyramidal neurons with apical and basal dendrites projecting out of the large intensively stained soma. These pyramidal cells are laconically expressed and properly delineated in the CA3 region of Moringa treated control animals. The adjacent polymorphic layers on either side of the conus amonus (CA3) regions have cells expressed with brevity. There are no signs of pyknosis or apoptosis as it can be observed that each of the pyramidal cells stand out distinctly and not compactly with other pyramidal cells. The pyramidal cells in the CA3 region of the hippocampus of rats treated with CPZ shows pycnotic pyramidal cells organized in cluster and apoptotic CA3 cells appearing to be poorly stained as a sign of degenerative changes. The cellular assortment of the CA3 region of the hippocampus alongside the polymorphic layer adjoining it on either side appears distorted. Comparatively, the cellular assortment of pyramidal cells in the CA3 region of the rats that received a combined treatment of CPZ and MOR (group D) shows a better assortment and properly delineated cytoarchitectural manifestation when compared to that of the CPZ treated animals. Even though there appears to be a few chromatolytic cells cells, the histomorphological presentation of the CA3 region of the hippocampus of group D rats characteristically looks similar to those of the control and Moringa treated animals. There appeared to be distinctly stained pyramidal cells with apical and basal dendrites projecting out of the cell body. Similarly, histochemical demonstration of the prefrontal cortex (PFC) of control and MOR treated rats show intensively stained pyramidal cells with conspicuous apical and basal dendrites (arrows) and no sign of degeneration. CPZ-treated animal PFC slides present with chromatolytic and pyknotic cells (dotted circle) as well as reduced cellular density. Animals treated with both CPZ and MOR presented with mild signs of neuronal degeneration but the pyramidal neurons were intensively stained and better delineated than the CPZtreated animals (Cresyl fast violet stain, ×400).
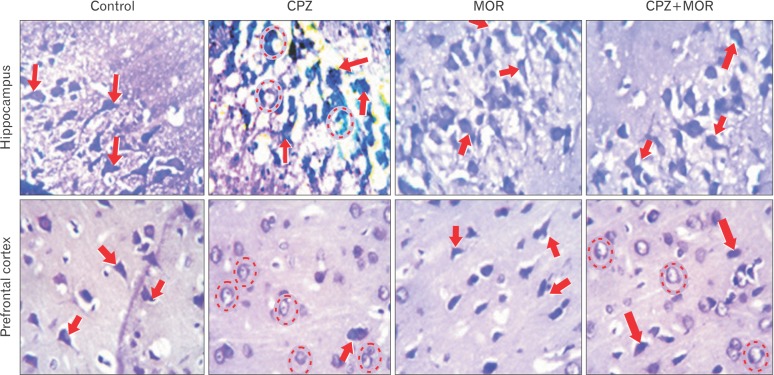
Table 1
Mean and standard error of mean of the total number of entries of experimental animals in the Y-maze test showing no statistical significant difference across the experimental groups
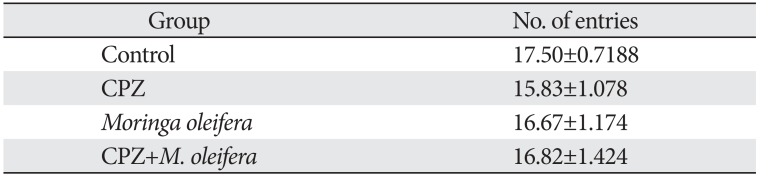
Group | No. of entries |
---|---|
Control | 17.50±0.7188 |
CPZ | 15.83±1.078 |
Moringa oleifera | 16.67±1.174 |
CPZ+M. oleifera | 16.82±1.424 |