Abstract
Purpose
The purpose of this study was to compare the phototoxic effects of blue light exposure on periodontal pathogens in both planktonic and biofilm cultures.
Methods
Strains of Aggregatibacter actinomycetemcomitans, Fusobacterium nucleatum, and Porphyromonas gingivalis, in planktonic or biofilm states, were exposed to visible light at wavelengths of 400.520 nm. A quartz-tungsten-halogen lamp at a power density of 500 mW/cm2 was used for the light source. Each sample was exposed to 15, 30, 60, 90, or 120 seconds of each bacterial strain in the planktonic or biofilm state. Confocal scanning laser microscopy (CSLM) was used to observe the distribution of live/dead bacterial cells in biofilms. After light exposure, the bacterial killing rates were calculated from colony forming unit (CFU) counts.
Results
CLSM images that were obtained from biofilms showed a mixture of dead and live bacterial cells extending to a depth of 30-45 µm. Obvious differences in the live-to-dead bacterial cell ratio were found in P. gingivalis biofilm according to light exposure time. In the planktonic state, almost all bacteria were killed with 60 seconds of light exposure to F. nucleatum (99.1%) and with 15 seconds to P. gingivalis (100%). In the biofilm state, however, only the CFU of P. gingivalis demonstrated a decreasing tendency with increasing light exposure time, and there was a lower efficacy of phototoxicity to P. gingivalis as biofilm than in the planktonic state.
Conclusions
Blue light exposure using a dental halogen curing unit is effective in reducing periodontal pathogens in the planktonic state. It is recommended that an adjunctive exogenous photosensitizer be used and that pathogens be exposed to visible light for clinical antimicrobial periodontal therapy.
Periodontal disease is very common in adults. Periodontal disease results from inflammation of the periodontal tissue around the teeth. Various periodontopathic bacteria cause these periodontal infections [1]. These periodontopathic bacteria exist in the gingival sulcus and periodontal pockets in the biofilm state. Biofilms that colonize the teeth and periodontal pockets are among the most complex biofilms that exist in the ecosystem [2]. The most frequently used method for periodontal disease treatment is mechanical debridement of biofilm. Nevertheless, it has been demonstrated that mechanical removal of biofilm cannot completely remove all periodontal pathogens from the tooth surface. The anatomical complexity of the roots (furcation areas and root concavities) [3,4] and bacteria invading the periodontal supporting tissue [5-7] are among the reasons for this phenomenon. Accordingly, systemic or local antibiotics have been used to overcome this problem. However, biofilm exhibits several antibiotic-resistance mechanisms [8-10]. The difficulty in maintaining therapeutic antimicrobial concentrations in the oral cavity and disruption of the oral microflora are also problems associated with the use of antibiotics [11]. For these reasons, alternative methods of antimicrobial treatment for periodontal disease are being investigated.
Photodynamic therapy (PDT) is one of these alternative methods. PDT involves three indispensable components. These are visible light, a nontoxic photosensitizer, and oxygen [12]. Photosensitizers absorb the visible light that matches the wavelength of their peak absorption. A photochemical mechanism caused by photosensitizers results in bacterial death [6,13-15]. In most situations, an additional exogenous photosensitizer to the target bacteria is required for photosensitization. However, some bacteria do not require exogenous photosensitizers. Black-pigmented bacteria (BPB), such as porphyrins, indicate the use of an endogenous photosensitizer [16-18]. Although classified as anaerobes, the BPB species found in the oral cavity can tolerate low concentrations of oxygen, comparable to those levels in untreated human periodontal pockets [19,20]. These small amounts of oxygen render periodontal diseases susceptible to PDT.
Previous studies have shown that low-energy argon laser irradiation has phototoxic effects on Porphyromonas and Prevotella species [21,22]. Additionally, visible light has inactivated Porphyromonas gingivalis and Fusobacterium nucleatum without an exogenous photosensitizer [23,24]. However, most previous studies of the phototoxic effects of visible light have evaluated phototoxicity in the planktonic state of bacteria [22-25]. While a few studies have shown phototoxic effects of blue light on periodontal pathogens in the planktonic state, little attention has been paid to biofilm conditions.
Quartz-tungsten-halogen lamps (hereafter, "halogen lamps") irradiate blue light (400-520 nm wavelength) and are often used to cure composite resin materials. The purpose of this study was to compare the phototoxic effects on periodontal pathogens (Aggregatibacter actinomycetemcomitans, F. nucleatum, and P. gingivalis) in both planktonic and biofilm cultures exposed to blue light.
A. actinomycetemcomitans ATCC 33384, F. nucleatum ATCC 23726, and P. gingivalis ATCC 33277, obtained from the Korean Collection for Oral Microbiology (Chosun University, Gwangju, Korea), were used. P. gingivalis and F. nucleatum were grown in trypticase soy broth (BD Diagnostics, Sparks, MD, USA), 5 µg/mL of hemin (Sigma Chemical Co., St. Louis, MO, USA), and 1 µg/mL of menadione (Sigma Chemical Co.) under anaerobic conditions (Bactron Anaerobic Chamber, Sheldon Manufacturing Inc., Cornelius, OR, USA) in an atmosphere of 90% N2, 5% CO2, and 5% H2. A. actinomycetemcomitans was grown in brain heart infusion (BHI) broth (BD Diagnostics) under anaerobic conditions in an atmosphere of 90% N2, 5% CO2, and 5% H2.
All of the strains were subcultured twice before exposure to light. The turbidity of the bacterial suspension was measured by spectrophotometry. A standard curve was established for adjusting the bacterial numbers. The bacterial concentration after incubation (24 hours for A. actinomycetemcomitans and F. nucleatum, 48 hours for P. gingivalis) was standardized by dilution with sterile broth to 1×107 cells/mL. Bacterial samples were prepared before exposure to light in two experimental set-ups as follows: 1) bacteria in suspension (planktonic state): 150 µL of suspension was placed 96-well microtitration plates; and 2) bacteria in biofilm (see the following section).
For biofilm development, a bacterial inoculum in BHI, containing approximately 107 cells/mL, was prepared. One hundred fifty microliters of inoculum (approximately 1.5×106 bacteria) was placed carefully into wells with trypticase soy broth (BD Diagnostics) containing 1 mg/mL of yeast extract (BD Diagnostics), 5 µg/mL of hemin (Sigma Chemical Co.), 1 µg/mL of menadione (Sigma Chemical Co.), 5% sheep blood (Hanil-KOMED, Seongnam, Korea), and 1.5% Bacto agar (BD Diagnostics). Then, the plates were incubated in an anaerobic state (90% N2, 5% H2, and 5% CO2) at 35℃ for 7 days. After initial incubation for 48 hours, the liquid medium was carefully aspirated from each well, and the biofilms were replenished with fresh trypticase soy broth containing 1 mg/mL of yeast extracts, 5 µg/mL of hemin, and 1 µg/mL of menadione. Fresh broth was added daily to each well very slowly, to avoid damage to the biofilm [2].
A confocal scanning fluorescence microscope with lens (FV300, Olympus Co., Tokyo, Japan) was used to observe the distribution of live and dead bacteria in the biofilms. The biofilms were grown on solid medium in 24-well plates (to accommodate the confocal microscope objectives), as described above. The bacterial inoculum in BHI, containing 109 cells/mL, was prepared for optimum biofilm development. The bacteria in the biofilm were observed using a LIVE/DEAD BacLight Bacterial Viability Kit (Molecular Probes Inc., Eugene, OR, USA), according to the manufacturer's instructions. Live bacteria in biofilm were observed using SYTO 9 stain, and dead bacteria were observed using propidium iodide stain. The biofilms were stained in the dark at room temperature for 15 minutes. An argon laser (476 nm) was used as the excitation source for the reagents, and the fluorescence emitted was collected by two separate emission filters at 500 nm (SYTO 9) and 635 nm (propidium iodide), respectively. Sections were collected at 5 µm intervals, and the collected images were analyzed by image-processing techniques to assess the distribution of live/dead bacteria within the biofilm matrices.
We applied a commercially available visible light source in the blue range of the spectrum: a halogen lamp (400-520 nm) (3M Curing Light XL3000, 3M ESPE, St. Paul, MN, USA). The light beam irradiated at a diameter of 8 mm. The power output of the light was 500 mW/cm2. The distance between the light source tip and the sample surface was 10 mm. Each bacterial sample was exposed to 15, 30, 60, 90, or 120 seconds of light.
After light exposure of the bacteria in the planktonic state, the samples were diluted serially with sterile broth. Then, 100 µL aliquots were applied to the agar plates. After light exposure, we collected adherent bacteria from the blood agar in each well. The bacteria were gently scraped from the blood agar plates using a sterile bacteriological loop. The removed bacterial biofilms were dispersed in broth. Then, serial dilutions were prepared, and 100 µL aliquots were spread over the surfaces of the agar plates. A. actinomycetemcomitans was applied to blood agar plates (pancreatic digest of casein 14.5 g/L, papaic digest of soybean meal 5.0 g/L, sodium chloride 5.0 g/L, agar 14.0 g/L, growth factor 1.5 g/L, sheep blood 50 ml/L) (Hanil-KOMED), and F. nucleatum and P. gingivalis were applied to brucella blood agar plates (enzymatic digest of casein 10.0 g/L, enzymatic digest of animal tissue 10.0 g/L, sodium chloride 5.0 g/L, agar 15.0 g/L, vitamin K1 0.01 g/L, yeast extract 2.0 g/L, dextrose 1.0 g/L, sodium bisulfide 0.1 g/L, sheep blood 50 ml/L, hemin 5 mg/L) (Hanil-KOMED). Survival of these bacteria was determined by counting the colony-forming units (CFUs) after incubation. All of the bacteria were cultured under anaerobic conditions at 37℃ until bacterial colonies were visible (3-7 days). CFUs were calculated in each well, and the percentages of surviving bacteria were calculated in relation to the nonexposed samples (control group) under similar experimental conditions. All of the experiments were repeated at least twice.
The statistical analysis was performed using the IBM SPSS ver. 19.0 (IBM Co., Armonk, NY, USA). To assess the effects of the light exposure time on CFU changes in the same bacterial biofilm, the Kruskal-Wallis test was applied. The level of significance was P<0.05. Correlations between CFU and light exposure time in the planktonic and biofilm states of the bacterial strains were evaluated. Spearman's rank correlation coefficients (Spearman's rho) were used.
We observed live and dead bacterial cell mixture in CSLM images (X-Y). The bacterial cell mixture was observed extending to a depth of 30-45 µm (Fig. 1). No fluorescence signal was observed in the biofilm below 45 µm. An obvious difference in the live/dead bacteria ratio was found in the P. gingivalis biofilms according to light exposure time (0 second vs. 120 seconds).
The CFU in each experimental group was tabulated for each light exposure time. The mean and standard deviation values of the CFU obtained are shown in Figs. 2 and 3. In the bacterial strains in a planktonic state, the CFU values were significantly different according to light exposure time in F. nucleatum and P. gingivalis (Fig. 2). In the biofilm state, the CFU values were significantly different according to light exposure time in P. gingivalis (Fig. 3).
Table 1 demonstrates correlations between the CFU and light exposure time in the planktonic and biofilm states of the bacterial strains. The bacterial strains in the planktonic state showed a significant negative correlation between the CFU and light exposure time in A. actinomycetemcomitans and F. nucleatum. P. gingivalis showed a negative correlation, but was not statistically significant. The bacterial strains in the biofilm state failed to demonstrate a significant correlation between the CFU and light exposure time in A. actinomycetemcomitans and F. nucleatum. Only P. gingivalis showed a significant negative correlation between the CFU and light exposure time.
The present study compared the phototoxicity of blue light to A. actinomycetemcomitans, F. nucleatum, and P. gingivalis in the planktonic or biofilm state. BPB, such as P. gingivalis, use external heme as an iron source for their growth [26]. Additionally, BPB accumulate a cell-surface black pigment that consists mainly of iron protoporphyrin IX (PpIX) [27]. This endogenous porphyrin can act as a photosensitizer. Porphyrins exhibit an intense peak at approximately 405 nm on the ultraviolet/visible spectrum. This specific light spectrum causes excitation of PpIX. Transferred energy from the PpIX triplet state to molecular oxygen produces excited-state singlet oxygen. This excited-state singlet oxygen can oxidize and destroy lipids, proteins, and nucleic acids [28].
Noncoherent blue light sources, such as halogen lamps, are commonly used in dentistry for the photopolymerization of composites. Moreover, halogen light sources are attractive in that they are easy to operate, compact, and lightweight and have a lower cost compared with lasers. The light source used in the present study emitted 400 to 520 nm, similar to the region of absorption of porphyrin.
Applying blue light from a halogen lamp, we demonstrated a phototoxic effect on periodontal pathogens. In the planktonic state, a decreasing tendency in CFU counts was found according to increases in exposure time in all of the periodontal pathogens. Almost all of the bacteria were killed with 60 seconds of light exposure to F. nucleatum (99.1%) and with 15 seconds to P. gingivalis (100%) (Fig. 2). Light exposure of A. actinomycetemcomitans failed to demonstrate statistical significance regarding CFUs according to light exposure time. In the biofilm state, however, a decreasing tendency in CFU counts was found according to increases in exposure time in P. gingivalis only. A. actinomycetemcomitans and F. nucleatum not only failed to show statistical significance among CFUs according to light exposure time, but they also failed to show correlations between CFUs and exposure time (Fig. 3).
There are several explanations for the phototoxic effects of visible light on bacteria in the planktonic state. Visible light (408-750 nm) has been found to be mutagenic and to cause metabolic and membrane damage to bacteria such as Escherichia coli [29,30]. Feuerstein et al. [24] suggested that increases in temperature could damage bacteria after exposure to blue light.
In the present study, the results in the planktonic state seem to be similar to the results of several previous studies. Henry et al. [22] evaluated the phototoxicity of argon laser irradiation on Porphyromonas and Prevotella species in liquid media. BPB, including the bacteria in this study, demonstrated phototoxicity under oxygen conditions. Feuerstein et al. [24] investigated the phototoxicity to P. gingivalis and F. nucleatum of blue light from various photocuring light sources (quartz-tungsten-halogen lamp, light-emitting diode, plasma-arc) at wavelengths of 400-500 nm. They suggested that visible light sources without exogenous photosensitizers have a phototoxic effect, mainly on gram-negative periodontal pathogens. Bacterial samples were also exposed to a near-infrared diode laser (wavelength 830 nm) for comparison. However, the near-infrared diode laser did not affect any of the bacteria tested.
In this study, the lower efficacy of light exposure in the biofilm state might have been a reflection of the absence of light exposure effects, as mentioned above. However, light exposure seems to be effective in P. gingivalis because of the existence of endogenous porphyrin. Blue light exposure could contribute to the activation of the endogenous porphyrin of P. gingivalis and to the generation of the reactive oxygen species responsible for cell death. Nevertheless, the rate of killing of P. gingivalis at all exposure times seems to be lower in the biofilm state, compared to the planktonic state (Figs. 2 and 3). It is likely that the phototoxic effects on periodontal pathogens would be greater in the planktonic state than in the biofilm state.
Fontana et al. [2] investigated the effects of the exposure of various bacteria from human dental plaque to PDT in vitro under planktonic or biofilm conditions. They reported a reduced susceptibility of biofilms to PDT when using methylene blue and a diode laser. Several investigators have reported similar results demonstrating reduced susceptibility of biofilms to PDT [31,32]. They proposed a possible cause to this phenomenon: incomplete permeation of the photosensitizer into the biofilm bacteria. However, we found the reduced susceptibility of biofilms to blue light exposure despite the absence of an exogenous photosensitizer in this study. We developed single-bacterial biofilms according to the biofilm forming method of Fontana et al. [2]. We were able to identify a tendency toward a decreasing proportion of dead cells with an increasing depth of the biofilm (Fig. 1). This might have resulted from a lower layer of the biofilm not being fully enough exposed to visible light to cause bacterial death because of turbidity of the biofilm.
Two possible explanations for the lower efficacy of phototoxicity on P. gingivalis in biofilm compared to the planktonic state are quorum interaction between bacterial cells [33] and imbalance of oxygen distribution in the biofilm [34].
Most bacterial species communicate with specific signal molecules. This mechanism is known as quorum sensing [35]. Dahl et al. [33] proposed that the gram-negative cell wall can also act as a kind of signal molecule. This cell wall can accentuate the rate of cell killing. Therefore, it is conceivable that the difference in the sensitivity of the bacterial cell to killing in the two conditions (planktonic and biofilm) is attributable to variances in the level of these reaction products.
Werner et al. [34] reported the heterogeneity of the anabolic pattern inside Pseudomonas aeruginosa biofilms. Microelectrode probing has shown that the bottom of a biofilm is anaerobic and that little bacterial growth and metabolic activity occurs there. On the other hand, the conditions at the surface of the biofilm are aerobic and allow more growth and metabolic activity. These physiological conditions may partly explain the resistance of biofilm-growing bacteria to antimicrobial therapy including PDT and phototherapy [36]. Feuerstein et al. [23] suggested that the mechanism of blue visible light phototoxicity to P. gingivalis and F. nucleatum is oxygen dependent, which might result in the formation of mainly hydroxyl radicals. A phototoxic effect did not occur under anaerobic conditions. Oxygen seems to be the inevitable factor for phototoxicity [12].
The result of this study showed that blue light exposure is available to reduce periodontal pathogens in the planktonic state. However, periodontal pathogens in the intraoral environment exist in a biofilm state. Blue light exposure to periodontal periodontal pathogens in the biofilm state was less effective than in the planktonic state. Little phototoxic effect was shown even in the biofilm states of A. actinomycetemcomitans and F. nucleatum. Therefore, it is recommended that an adjunctive exogenous photosensitizer (e.g., methylene blue, toluidine blue O, erythrosine) be used when visible light exposure is used for antimicrobial periodontal therapy. Further clinical study is required to evaluate the effect of blue light exposure in vivo.
Figures and Tables
Figure 1
Confocal images (horizontal X-Y sections). Live bacteria were stained fluorescent green using SYTO 9 stain, while dead bacteria were stained fluorescent red using propidium iodide. The values on the left represent the distance from the biofilm surface. (A) Aggregatibacter actinomycetemcomitans, (B) Fusobacterium nucleatum, and (C) Porphyromonas gingivalis.
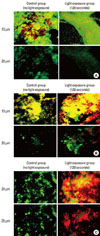
Figure 2
Mean colony forming unit (CFU) values of each bacterial strain in the planktonic state according to light exposure time.
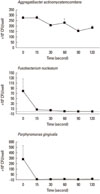
References
1. Darveau RP, Tanner A, Page RC. The microbial challenge in periodontitis. Periodontol 2000. 1997. 14:12–32.


2. Fontana CR, Abernethy AD, Som S, Ruggiero K, Doucette S, Marcantonio RC, et al. The antibacterial effect of photodynamic therapy in dental plaque-derived biofilms. J Periodontal Res. 2009. 44:751–759.


3. Adriaens PA, Adriaens LM. Effects of nonsurgical periodontal therapy on hard and soft tissues. Periodontol 2000. 2004. 36:121–145.


4. Umeda M, Takeuchi Y, Noguchi K, Huang Y, Koshy G, Ishikawa I. Effects of nonsurgical periodontal therapy on the microbiota. Periodontol 2000. 2004. 36:98–120.


5. Amano A. Disruption of epithelial barrier and impairment of cellular function by Porphyromonas gingivalis. Front Biosci. 2007. 12:3965–3974.


6. Meyer DH, Sreenivasan PK, Fives-Taylor PM. Evidence for invasion of a human oral cell line by Actinobacillus actinomycetemcomitans. Infect Immun. 1991. 59:2719–2726.


7. Thiha K, Takeuchi Y, Umeda M, Huang Y, Ohnishi M, Ishikawa I. Identification of periodontopathic bacteria in gingival tissue of Japanese periodontitis patients. Oral Microbiol Immunol. 2007. 22:201–207.


8. Anderson GG, O'Toole GA. Innate and induced resistance mechanisms of bacterial biofilms. Curr Top Microbiol Immunol. 2008. 322:85–105.


9. del Pozo JL, Patel R. The challenge of treating biofilm-associated bacterial infections. Clin Pharmacol Ther. 2007. 82:204–209.


10. Fux CA, Costerton JW, Stewart PS, Stoodley P. Survival strategies of infectious biofilms. Trends Microbiol. 2005. 13:34–40.


11. Wilson M. Lethal photosensitisation of oral bacteria and its potential application in the photodynamic therapy of oral infections. Photochem Photobiol Sci. 2004. 3:412–418.


12. Takasaki AA, Aoki A, Mizutani K, Schwarz F, Sculean A, Wang CY, et al. Application of antimicrobial photodynamic therapy in periodontal and peri-implant diseases. Periodontol 2000. 2009. 51:109–140.


13. Maisch T. Anti-microbial photodynamic therapy: useful in the future? Lasers Med Sci. 2007. 22:83–91.


14. Maisch T, Szeimies RM, Jori G, Abels C. Antibacterial photodynamic therapy in dermatology. Photochem Photobiol Sci. 2004. 3:907–917.


15. Sharman WM, Allen CM, van Lier JE. Photodynamic therapeutics: basic principles and clinical applications. Drug Discov Today. 1999. 4:507–517.


16. Duerden BI. Pigment production by Bacteroides species with reference to sub-classification. J Med Microbiol. 1975. 8:113–125.


17. Reid JS, Beeley JA, MacFarlane TW. A study of the pigment produced by Bacteroides melaninogenicus. J Dent Res. 1976. 55:1130.


18. Shah HN, Bonnett R, Mateen B, Williams RA. The porphyrin pigmentation of subspecies of Bacteroides melaninogenicus. Biochem J. 1979. 180:45–50.


20. Loesche WJ, Gusberti F, Mettraux G, Higgins T, Syed S. Relationship between oxygen tension and subgingival bacterial flora in untreated human periodontal pockets. Infect Immun. 1983. 42:659–667.


21. Henry CA, Dyer B, Wagner M, Judy M, Matthews JL. Phototoxicity of argon laser irradiation on biofilms of Porphyromonas and Prevotella species. J Photochem Photobiol B. 1996. 34:123–128.


22. Henry CA, Judy M, Dyer B, Wagner M, Matthews JL. Sensitivity of Porphyromonas and Prevotella species in liquid media to argon laser. Photochem Photobiol. 1995. 61:410–413.


23. Feuerstein O, Ginsburg I, Dayan E, Veler D, Weiss EI. Mechanism of visible light phototoxicity on Porphyromonas gingivalis and Fusobacterium nucleatum. Photochem Photobiol. 2005. 81:1186–1189.


24. Feuerstein O, Persman N, Weiss EI. Phototoxic effect of visible light on Porphyromonas gingivalis and Fusobacterium nucleatum: an in vitro study. Photochem Photobiol. 2004. 80:412–415.


25. Soukos NS, Som S, Abernethy AD, Ruggiero K, Dunham J, Lee C, et al. Phototargeting oral black-pigmented bacteria. Antimicrob Agents Chemother. 2005. 49:1391–1396.


26. Okamoto K, Nakayama K, Kadowaki T, Abe N, Ratnayake DB, Yamamoto K. Involvement of a lysine-specific cysteine proteinase in hemoglobin adsorption and heme accumulation by Porphyromonas gingivalis. J Biol Chem. 1998. 273:21225–21231.


27. Smalley JW, Silver J, Marsh PJ, Birss AJ. The periodontopathogen Porphyromonas gingivalis binds iron protoporphyrin IX in the mu-oxo dimeric form: an oxidative buffer and possible pathogenic mechanism. Biochem J. 1998. 331(Pt 3):681–685.


28. Redmond RW, Gamlin JN. A compilation of singlet oxygen yields from biologically relevant molecules. Photochem Photobiol. 1999. 70:391–475.


29. Gourmelon M, Cillard J, Pommepuy M. Visible light damage to Escherichia coli in seawater: oxidative stress hypothesis. J Appl Bacteriol. 1994. 77:105–112.


30. Webb RB, Malina MM. Mutagenesis in Escherichia coli by visible light. Science. 1967. 156:1104–1105.
31. Müller P, Guggenheim B, Schmidlin PR. Efficacy of gasiform ozone and photodynamic therapy on a multispecies oral biofilm in vitro. Eur J Oral Sci. 2007. 115:77–80.


32. Soukos NS, Socransky SS, Mulholland SE, Lee S, Doukas AG. Photomechanical drug delivery into bacterial biofilms. Pharm Res. 2000. 17:405–409.
33. Dahl TA, Midden WR, Hartman PE. Comparison of killing of gram-negative and gram-positive bacteria by pure singlet oxygen. J Bacteriol. 1989. 171:2188–2194.


34. Werner E, Roe F, Bugnicourt A, Franklin MJ, Heydorn A, Molin S, et al. Stratified growth in Pseudomonas aeruginosa biofilms. Appl Environ Microbiol. 2004. 70:6188–6196.

