Abstract
Anesthetics are used extensively in surgeries and related procedures to prevent pain. However, there is some concern regarding neuronal degeneration and cognitive deficits arising from regular anesthetic exposure. Recent studies have indicated that brain-derived neurotrophic factor (BDNF) and cyclic AMP response element-binding protein (CREB) are involved in learning and memory processes. Genistein, a plant-derived isoflavone, has been shown to exhibit neuroprotective effects. The present study was performed to examine the protective effect of genistein against isoflurane-induced neurotoxicity in rats. Neonatal rats were exposed to isoflurane (0.75%, 6 hours) on postnatal day 7 (P7). Separate groups of rat pups were orally administered genistein at doses of 20, 40, or 80 mg/kg body weight from P3 to P15 and then exposed to isoflurane anesthesia on P7. Neuronal apoptosis was detected by TUNEL assay and FluoroJade B staining following isoflurane exposure. Genistein significantly reduced apoptosis in the hippocampus, reduced the expression of proapoptotic factors (Bad, Bax, and cleaved caspase-3), and increased the expression of Bcl-2 and Bcl-xL. RT-PCR analysis revealed enhanced BDNF and TrkB mRNA levels. Genistein effectively upregulated cAMP levels and phosphorylation of CREB and TrkB, leading to activation of cAMP/CREB-BDNF-TrkB signaling. PI3K/Akt signaling was also significantly activated. Genistein administration improved general behavior and enhanced learning and memory in the rats. These observations suggest that genistein exerts neuroprotective effects by suppressing isoflurane-induced neuronal apoptosis and by activating cAMP/CREB-BDNF-TrkB-PI3/Akt signaling.
Inhalation anesthetics, such as isoflurane, are commonly used in clinical practice. However, accumulating experimental evidence suggests that early exposure to volatile anesthetics can cause neurodegeneration in the developing animal brain [12345]. Furthermore, anesthetic exposure has been reported to show correlations with persistent learning and behavioral deficits [67]. Various mechanisms have even been proposed for anestheticinduced neuroapoptosis and memory deficits [8910]. A better understanding of the mechanisms would aid the development of strategies that could prevent or reduce anesthetic-induced apoptosis and memory impairments.
Cyclic AMP response element-binding protein (CREB) is widely involved in learning and memory [11] and in long-term potentiation (LTP) [12]. CREB plays critical roles in hippocampal neurogenesis, neuronal survival and differentiation, and neuroprotection [131415]. Inhibition of phosphodiesterase-4 (PDE4) leads to increased cAMP levels, resulting in phosphorylation of CREB [1617]. Phosphorylation/activation of CREB (pCREB) was found to be critical for long-term memory consolidation [1819]. Further studies showed that anesthetic isoflurane and sevoflurane downregulate cAMP/CREB signaling [920].
Brain-derived neurotrophic factor (BDNF) is an important neuroprotective factor that plays significant roles in neuronal development, synaptogenesis, learning, and memory [2122]. BDNF exerts its functions through activation of specific cell surface receptors, TrkB and p75 neurotrophin receptor [23]. Activation of TrkB was shown to be essential for the survivalpromoting functions of BDNF [24]. Furthermore, BDNF activates the phosphoinositide 3-kinase (PI3K)/protein kinase B (Akt) signaling pathway in neurons [25]. The PI3K/Akt pathway is expressed widely in the CNS, and it mediates cell survival, proliferation, and differentiation. It is also involved in learning and memory formation [2627]. Initiation of the BDNF/TrkB/PI3-K/Akt signal pathway in the hippocampus is crucial for working memory formation [28]. Impaired BDNF-TrkB signaling has recently been proposed in anesthetic-induced neurotoxicity [29].
Genistein, 5,7-dihydroxy-3-(4-hydroxyphenyl)-4H-1-benzopyran-4-one, is an isoflavone widely present in leguminous plants [30]. Genistein was found to exert a wide spectrum of biological activities, including antioxidant [31], anti-inflammatory [32], hepatoprotective [33], neuroprotective [34], and antimetastatic effects [35]. The present study was performed to determine whether genistein can reduce neuronal apoptosis, modulate cAMP/CREB–BDNF/TrkB/PI3K signaling, and improve memory and learning in rats exposed to isoflurane.
Genistein and isoflurane (0.75%) were obtained from Sigma-Aldrich (St. Louis, MO, USA). For expression analysis, antibodies against CREB, p-CREB, cleaved caspase-3, Bcl-2, Bad, Bcl-xL, Bax, β-actin, phosphatase and tensin homolog (PTEN), and mammalian target of rapamycin complex 1 (mTORc1) were purchased from Cell Signaling Technology (Beverly, MA, USA). Akt, p-Akt, GSK-3β, p- GSK-3β, BDNF, TrkB, and p-TrkB were obtained from Santa Cruz Biotechnology (Santa Cruz, CA, USA). PDE4 and Ca2+/calmodulin-dependent kinase IV (CaMKIV) were from Abcam. All other chemicals and reagents used in the present study were purchased from Sigma-Aldrich unless otherwise noted.
This study and its experimental design were approved by the animal experimentation ethics committee of the hospital, and all techniques were performed in compliance with the guidelines issued for the care and use of laboratory animals by the NIH and National Animal Welfare Law of China. Pregnant Sprague–Dawley rats were housed in individual sterile plastic cages under standard animal house conditions (12-h day/night cycle, 23℃±2℃) at 55~65% humidity levels. The rats were given free access to standard pellet food and water. Animals were monitored carefully for the birth of pups, and the day on which pups were born was designated as postnatal day 0 (P0). The pups were housed in sterile cages and carefully maintained under the same conditions as described above.
Separate groups of rat pups were treated orally with genistein at 20, 40, or 80 mg/kg body weight every day from P3 to P15 along with the standard diet. On P7, the pups were exposed to 0.75% isoflurane (6 h) in 30% oxygen or air [~0.3 minimum alveolar concentration (MAC)] as described by Orliaguet et al.[36] in a temperature-controlled chamber [5]. Rats in the control group were not exposed to isoflurane and were not given genistein. P7 was chosen for anesthetic exposure based on previous studies suggesting that rats are most sensitive to anesthesia-induced neuronal damage during this period [1]. For analysis of neuroapoptosis, cAMP levels, and gene expression, the rat pups were sacrificed 1 h after anesthetic exposure. The animals were perfused transcardially with ice-cold saline and 4% paraformaldehyde in 0.1 M phosphate buffer.
The influence of genistein on isoflurane-induced neuronal apoptosis was determined by TUNEL assay as described by Li et al. [5]. The brain tissues were cut into sections at a thickness of 5 µm, and apoptosis was assessed using the Dead End™ fluorometric TUNEL system kit (Promega, Madison, WI, USA) according to the manufacturer's instructions. The numbers of TUNEL-positive cells in the hippocampal CA1, CA3, and dentate gyrus sections were determined and analyzed using NIS-Elements BR image processing and analysis software (Nikon Corporation, Tokyo, Japan). The concentration of TUNEL-positive cells in each region is presented as the number of TUNEL-positive cells/mm2.
Fluoro-Jade B (FJB) staining was performed to determine neurodegeneration. Hippocampal sections (30 µm thick) were fixed on slides coated with gelatin and dried at room temperature overnight. Slides were rehydrated and then incubated in potassium permanganate (0.06%) for 15 min, rinsed with distilled H2O, and stained with FJB. The sections were further incubated with 0.1% acetic acid for 30 min and observed under a microscope (DM IRB; Leica, Wetzlar, Germany).
Cyclic AMP (cAMP) levels in the hippocampal tissues were determined using a cAMP complete ELISA kit in accordance with the manufacturer's protocol (Enzo Life Sciences, Farmingdale, NY, USA). The cAMP levels were expressed as pmol/mg.
RT-PCR was performed to assess the influence of genistein on BDNF and TrkB gene expression in the hippocampal tissues of isoflurane anesthesia-treated rat pups. Total RNA was isolated from the hippocampi using Trizol (Invitrogen, Carlsbad, CA, USA), and the RNA concentration was determined using a Nanodrop spectrophotometer (ND 1000; Bio-Rad, Hercules, CA, USA). First-strand complementary DNA (cDNA) was synthesized using the Revert Aid First Strand cDNA Synthesis Kit (Fermentas, Glen Burnie, MD, USA). PCR was performed according to the manufacturer's protocol. The primer sequences for BDNF and TrkB were as follows: BDNF, Forward: 5'-CGAAGAGCTGCTGGATGAG-3', Reverse: 5'-ATGGGATTACACTTGGTCTCG-3'. TrkB, Forward: 5'-CCTCCACGGATGTTGCTGA-3', Reverse: 5'-GGCTGTTGGTGATACCGAAGTA-3'. GAPDH expression was assessed as an internal control using the following primer sequences: Forward: 5'-CCGTATCGGACGCCTGGTTA-3', Reverse: 5'-GGCTGTTGGTGATACCGAAGTA-3'. PCR products were separated on agarose gels (1%) and stained with 0.05% ethidium bromide. Band intensities were analyzed using a Bio Gel imagery apparatus (Bio Rad).
The harvested hippocampal tissues were subjected to expression analysis by Western blotting as described previously [3738]. Briefly, the tissues were homogenized in lysis buffer (10 mM Tris-HCl, pH 7.4, 150 mM NaCl, 2 mM EDTA, 0.5% Nonidet P-40) with protease inhibitors (aprotinin, pepstatin A, and leupeptin) at mg/mL concentrations for protein extraction. The cell lysate was centrifuged (12,000 g for 10 min at 4℃), the supernatant was removed, and the total protein concentration was determined using a protein assay kit (Bio-Rad). Equal amounts of sample protein (50 µg) were electrophoresed in NuPAGE Novex Bis-Tris gradient gels (Invitrogen). The separated bands were then blotted onto nitrocellulose membranes and incubated with blocking solution (0.1% TBST and 5% non-fat milk) for 2 h, followed by incubation with primary antibodies overnight at 4℃. The membranes were washed three times in TBST and then incubated with horseradish peroxidase-conjugated secondary antibodies (Cell Signaling Technology) for 60 min. Following five to six washes in TBST, immunoreactive bands were visualized using an ECL detection kit (GE Healthcare, Fairfield, CT, USA) and analyzed using Image J software (NIH Image, Bethesda, MD, USA). Protein expression was normalized relative to the expression of β-actin as an internal standard.
P35 rats exposed to anesthesia on P7 were subjected to open field tests to evaluate their anxiety behavior and general locomotory activity. The rats were placed in a white plastic chamber (100×100×100 cm) for 5 min, and exploratory behavior in the novel environment was recorded using a video tracking system (XR-XZ301; Shanghai Soft maze Information Technology Co., Ltd., Shanghai, China).
Hippocampal-dependent and -independent responses were assessed by a fear conditioning test performed as described previously [3940]. P35 rats were subjected to fear stimuli. Each rat was placed in a test chamber in a dark room. The chamber had grid floors with stainless steel bars attached to a shock delivery system (Coulbourn, Whitehall, PA, USA). The animals were exposed to three tone–foot shock pairings (tone: 2000 Hz, 85 db, 30 s followed by foot shock: 1 mA, 2 s) at 1-min intervals. Animals were removed from the chamber 30 s after conditioning. After 24 h, the same animals were placed in the chamber, one animal at a time for a period of 8 min, in the absence of tone and electric shock. The behavior and freezing response of the animals were recorded. Two hours later, the animals were placed in a test chamber that varied from the first test chamber in context and smell (instead of ethanol, as used in the first chamber, 1% acetic acid was used to wipe the second chamber) in a relatively light room. Freezing responses were documented for 3 min without auditory conditioning stimulus. The auditory stimulus was turned on for 30 s in three cycles with a 60 s inter-cycle interval. The freezing behavior was recorded.
The Morris water maze test was used to assess learning ability and memory retention. For the Morris water maze test (Shanghai Jiliang Software Technology Co. Ltd., Shanghai, China), a circular pool was filled with warm water (25±1℃) approximately 1.5 cm above a transparent round platform 15 cm in diameter that was placed in any of the four quadrants of the pool. The platform was placed in the same position throughout the training period. P31 rats that were administered genistein and/or exposed to isoflurane on P7 were trained to explore the maze. The rats were trained in two sessions/day for 4 consecutive days. The animals were permitted to swim freely in the pool until they reached the platform. If the rats were unable to find the submerged platform within 60 s, they were directed to the platform and allowed to remain there for 30 s. The swimming path was observed using an automated video tracking system (ANY-maze video tracking system; Stoelting Co., Wood Dale, IL, USA). The time taken by the rats to reach the platform was recorded as the latency.
After 4 days of trial sessions, cued trials were conducted on P35 to evaluate non-cognitive impairments, such as visual impairments and/or any difficulties in swimming. The circular pool was surrounded with a black cloth to hide any visual cues. The rats were subjected to four trials/day. Each rat was positioned in a defined place within the pool during the trials and was allowed to swim and locate the submerged platform, which was attached to a rod fixed ~20 cm above water level. The rod served as the cue. The time taken to locate the cued platform was recorded as mentioned above.
For the place trials, the cloth surrounding the pool and the cue rod attached to the platform were removed. Rats were positioned at random points and allowed to locate the platform; the time taken to locate the platform was recorded.
Probe trials were conducted to assess memory retention. The test was performed 24 h after the place trials. The platform was placed in a different quadrant than where the submerged platform had been during the cued and place trials (target quadrant). The time spent by the rats in the target quadrant searching for the submerged platform was recorded.
The results are presented as means±standard deviation (SD) of six independent experiments. Data were analyzed for statistical significance by one-way analysis of variance (ANOVA) followed by Duncan's multiple range test as a post hoc analysis using SPSS (version 22.0; SPSS, Chicago, IL, USA). In all analyses, p<0.05 was taken to indicate statistical significance.
Several studies have indicated severe neuronal death following exposure to volatile anesthesia [141]. Consistent with these previous reports, increased apoptotic cell counts were observed 6 h after isoflurane exposure in this study (Fig. 1A). A significant increase (p<0.05) in the TUNEL-positive cell count was observed in rat pups exposed to isoflurane alone. Staining with FJB, an anionic X fluorescein derivative that specifically stains degenerating neurons, also revealed severe neuronal apoptosis in the hippocampal CA1, CA3, and dentate gyrus regions (Fig. 1B), and increased numbers of FJB-positive neurons were seen in the hippocampi of P7 rat pups that had been exposed to isoflurane. Genistein at 20, 40, or 80 mg significantly (p<0.05) reduced FJB-positive cell counts, indicating decreased neuronal degeneration. Genistein at a dose of 80 mg, compared with lower doses, decreased TUNEL-positive and FJB-positive cell counts more effectively.
The greatest susceptibility to anesthetic neurotoxicity is considered to occur at P7, as rapid synaptogenesis and brain development occur at this time [142]. Therefore, we examined the effects of genistein on the levels of proteins involved in the apoptotic pathway, including cleaved caspase-3. Western blotting analysis revealed marked increases in cleaved caspase-3 levels, a marker of anesthetic-induced toxicity [143], following isoflurane exposure (Fig. 2). Further, significantly (p<0.05) enhanced expression levels of proapoptotic proteins, Bad and Bax, were seen with decreased (p<0.05) levels of the antiapoptotic proteins, Bcl-2 and Bcl-xL (Fig. 2). These alterations in expression indicated increased levels of apoptosis in the hippocampi of P7 rats. The enhanced expression levels of caspase-3, Bad, and Bax proteins were consistent with the changes in TUNEL-positive and FJB-positive cell counts. However, genistein administration to the rat pups prior to isoflurane markedly (p<0.05) inhibited expression of the proapoptotic proteins Bax and Bad, resulting in significant (p<0.05) increases in the expression levels of Bcl-2 and Bcl-xL. At all three doses evaluated, genistein enhanced antiapoptotic protein expression and reduced proapoptotic protein expression; however, the effect was more pronounced at a dose of 80 mg than at 40 mg or 20 mg genistein. The levels of Bad and Bax protein expression were reduced to 102.2% and 102.0% respectively, following treatment with 80 mg genistein.
Initial exposure to various anesthetic compounds causes neuroapoptosis, memory deficits, and cognitive impairments [444546]. CREB is a significant downstream transcription factor of the cAMP and Ca2+ signal transduction pathways that critically regulates the expression of genes involved in memory consolidation [4748]. We assessed the influence of genistein on CREB expression and activation. CREB and CaMKIV expression levels were significantly (p<0.05) reduced following isoflurane exposure (Fig. 3). Levels of phosphorylated CREB were also markedly (p<0.05) reduced, while expression of the enzyme PDE4 was upregulated (p<0.05). Nevertheless, genistein inhibited (p<0.05) PDE4 levels and enhanced phosphorylation of CREB in a dose-dependent manner. Genistein at a dose of 80 mg significantly (p<0.05) increased the expression levels of total CREB and CaMKIV to 101.0% and 100.0% respectively. The upregulation of phosphorylated CREB levels could be due to direct upregulation of phosphorylation by genistein or may be due to enhanced expression of CREB by genistein itself. However, the enhanced phosphorylation of CREB along with upregulation of CaMKIV levels suggested activation of CREB and improved CREB signaling.
CREB is phosphorylated and activated by the enzymes protein kinase A (PKA) and CaMKIV [474950]. cAMP accumulation activates PKA [51]. We assessed the levels of cAMP following isoflurane exposure. A marked (p<0.05) decrease in cAMP levels after isoflurane exposure (Fig. 4) was observed, indicating that this decrease could have contributed in part to the decrease in CREB phosphorylation. Consistent with increased pCREB levels, we observed marked (p<0.05) increases in cAMP levels after genistein administration. The expression levels of cAMP increased by 0.41%, 0.67%, and 0.81% following treatment with genistein at doses of 20, 40, and 80 mg, respectively. These observations indicate that genistein can increase a part of CREB phosphorylation via the cAMP pathway, thereby upregulating cAMP/CREB signaling.
Growth factor signaling induces the intracellular signaling cascade that leads to phosphorylation and activation of CREB [52]. Isoflurane was found to cause significant (p<0.05) downregulation of BDNF (Fig. 5) and TrkB expression. Activation of TrkB was reduced following exposure to isoflurane. RT-PCR analysis indicated significant (p<0.05) upregulation of BDNF and TrkB mRNA levels in rats administered genistein prior to anesthetic exposure. Further, BDNF and TrkB protein expression levels were upregulated (p<0.05). Genistein improved (p<0.05) TrkB activation in a dose-dependent manner, as indicated by significantly elevated (p<0.05) phosphorylated TrkB levels; phosphorylated TrkB levels increased by 66.7% after treatment with 20 mg genistein, while 80 mg genistein resulted in an increase of 93.2%. Genistein-mediated upregulation in phosphorylated TrkB levels could be due either to the direct influence of the drug on increased phosphorylation or to the increase in expression of TrkB itself. Genistein-mediated upregulated BDNF/TrkB signaling may have contributed to the increased levels of phosphorylated CREB expression.
BDNF-TrkB-dependent and -independent cascades have been reported to modulate PI3K/Akt signaling, a major cell survival pathway in neurons [5354]. After finding that genistein upregulates BDNF-TrkB expression, we also examined whether it modulates the PI3K/Akt pathway. We observed significant (p<0.05) reductions in the levels of Akt, GSK-3β, and mTORc1 (Fig. 6). Western blotting analysis revealed significant increases in PTEN levels after isoflurane exposure, indicating downregulation of the pathway. Further, marked upregulation of the phosphorylated forms of Akt and GSK-3β were observed in genistein-treated animals. The levels of mTORc1 were also significantly increased (p<0.05), while those of PTEN were markedly decreased (p<0.05). PTEN expression was decreased from 176.1% to 114% after treatment with 80 mg genistein. These observations indicated that genistein activated PI3K/Akt signaling and aided neuronal survival. The observed effects of genistein could be either direct and/or mediated by activation of BDNF-TrkB signaling.
The general behavior of P35 rats following isoflurane exposure on P7 was examined. In the open field test, minor changes in the behavior of the rats exposed to anesthesia were noticed in comparison with control rats (Fig. 7). The fear conditioning test, a reliable test used to assess learning capacity, was also performed. The fear responses to shock and noise were recorded as freezing responses. The responses to cued and context conditioning of rats exposed to isoflurane alone were significantly impaired (p<0.05) (Fig. 7). The intensities of the fear responses were much higher (p<0.05) in rats treated with genistein compared with those treated with isoflurane alone, suggesting better learning.
Learning and memory are the most important features of cognition. We assessed learning and memory in P35 rats using Morris water maze tests. The experimental data revealed negligible changes in the escape latencies of the rats in the different test groups on day 1 of training (Fig. 8). Nevertheless, on day 2, minor changes in the latency were noted between rats exposed to isoflurane alone in comparison with the other groups; however, on days 3 and 4, the escape latencies were considerably longer. Differences were also noted in escape latencies on days 3 and 4 between rats treated with genistein versus isoflurane alone; rats treated with genistein showed a latency similar to that of normal controls.
The cued and place trials were performed to assess the ability of the rats to identify the submerged platform as a measure of their spatial navigation and memory. Rats exposed only to the anesthetic isoflurane showed longer escape latencies than did those in the other groups. This longer latency reflects the effects of isoflurane on spatial learning and memory. While the rats in the anesthetic control group took a longer time to reach the submerged platform in the pool, genistein-treated rats showed significantly better performance (Fig. 8). The animals took significantly (p<0.05) less time to reach the platform even in the absence of visual cues. Nevertheless, in probed trials, the rats treated with isoflurane alone spent considerably (p<0.05) less time in the target quadrant, suggesting impaired memory capacity. Rats administered genistein spent a longer time in the target quadrant searching for the platform. These observations indicated memory retention in the rats to search for the platform, suggesting that genistein supplementation significantly improved learning and memory in these animals.
Inhalation anesthetics can cause neuroapoptosis in the evolving brain [1555], and in line with earlier reports, the TUNEL assay and FJB staining revealed that exposure to isoflurane for 6 hours caused robust apoptosis in the hippocampal regions. Further, Western blot analysis was performed to assess the levels of cleaved caspase-3, a marker of apoptosis, and apoptotic pathway proteins. Isoflurane exposure caused significant upregulation of cleaved caspase-3 levels along with increased levels of the proapoptotic proteins Bax and Bad. Whereas the levels of Bad and Bax increased, those of Bcl-2 and Bcl-xL were severely suppressed by isoflurane. Bcl-2 and Bcl-xL are antiapoptotic proteins that belong to the Bcl-2 family. They block the translocation of Bax to the mitochondria, maintain mitochondrial integrity and membrane potential, and prevent the discharge of cytochrome C from the mitochondria and the initiation of apoptosis [56]. The increased levels of Bcl-2 and Bcl-xL with decreased levels of Bad and Bax observed after genistein treatment are indicative of apoptosis suppression. Decreased cleaved caspase-3 levels were observed after genistein treatment. This suppression of proapoptotic proteins and cleaved caspase-3 could have contributed to decreased apoptotic cell counts as observed in the TUNEL and FJB staining assays. These observations suggest the potential protective effects of genistein against isoflurane-induced neurotoxicity.
The PI3/Akt pathway is a major pathway involved in neuronal cell survival [57]. We observed significantly reduced expression of Akt, phosphorylated Akt, and mTORc1 following isoflurane exposure, indicating downregulated PI3K/Akt signaling. Genistein significantly increased Akt, phosphorylated Akt, and mTORc1 expression, while reducing PTEN levels in a dose-dependent manner, leading to activation of the PI3K/Akt signaling pathway. Activated Akt blocks Bad, which eventually leads to release of the antiapoptotic protein Bcl-xL, thus inhibiting apoptosis [58]. Therefore, activation of the pathway by genistein inhibited apoptosis and improved neuronal survival.
CREB plays a major role in long-term memory formation [475960]. Activation of CREB by phosphorylation is necessary for its function [52]. cAMP levels regulate activation of the enzyme PKA, which phosphorylates CREB [5161]. BDNF is a key target of CREB signaling [6263] and is important in the development and survival of neurons as well as in maturation of the developing brain and regulation of synaptic transmission in the hippocampus [2264]. BDNF/TrkB signaling is a major pathway in neuronal signaling in synaptic plasticity and memory [10].
Reduced levels of cAMP following isoflurane exposure suggest reduced activation of PKA, subsequently leading to decreased phosphorylated CREB levels and thus inhibition of cAMP/CREB signaling. Further, phosphorylated CREB levels suppressed by isoflurane exposure could also be due to decreased CaMKIV expression. Interestingly, significantly increased cAMP and CaMKIV levels along with decreased PDE4 observed after genistein administration indicate activated cAMP/CREB signaling. The increases in expression of BDNF and TrkB at both the gene and protein levels could be due to either direct action of genistein or indirect activation of CREB signaling. Further, BDNF has been reported to stimulate PI3K/Akt signaling, and thus activation of PI3K/Akt signaling also could have been due to increased BDNF expression, as observed with genistein treatment. Thus, genistein-mediated upregulation of PI3K/Akt signaling may have contributed partially to the decrease in neuronal apoptosis.
Isoflurane-induced cognitive dysfunction and memory impairments have been reported [13]. Genistein was shown to improve the general behavior as well as fear responses of the rats in a novel environment. Fear conditioning tests the spatial memory of rats. Improved responses suggest that genistein enhanced memory in the rats. Further, the behavior of rats exposed to isoflurane was also assessed using the Morris water maze test, which is considered the gold standard for assessing both learning ability and memory retention in behavioral neuroscience [65]. Spatial attainment of learning in the Morris water maze, represented as escape latency, was significantly improved by genistein. In addition, memory retention was higher in genistein-treated rats, suggesting the ability of genistein to improve the learning and memory of isoflurane-exposed rats effectively. Increased CREB-BDNF-TrkB signaling and decreased hippocampal neuroapoptosis observed after genistein treatment could have contributed to improved learning and memory.
The results of the present study suggested that genistein effectively activates cAMP/CREB-BDNF-TrkB–PI3K/Akt signaling, suppresses neuroapoptosis, and enhances learning and memory in rats following exposure to isoflurane. Further studies exploring the potential of genistein to reduce anesthetic-induced neurotoxicity are warranted.
Figures and Tables
Fig. 1
Genistein effectively reduced isoflurane-induced neuroapoptosis.
The effect of genistein (20, 40 or 80 mg) on neuronal apoptosis following isoflurane exposure was assessed in the hippocampal regions by (A) TUNEL assay and (B) Fluoro-Jade B staining. Geinstein dose-dependently reduced isoflurane-induced apoptosis. The data are presented as mean±SD, n=6. aStatistically significant differences at p<0.05 vs respective control. b–fSignificant differences (p<0.05) between mean values as determined by one-way analysis of variance (ANOVA) followed by Duncan's multiple range test.

Fig. 2
Genistein modulated the expression of proteins of the apoptotic cascade.
(A) Western blotting analysis revealed that genistein significantly (p<0.05) reduced isoflurane-induced raised expressions of cleaved caspase-3, and pro-apoptotic proteins (Bad, and Bax), while geinstein markedly (p<0.05) up-regulated the expression of anti-apoptotic proteins, Bcl-2 and Bcl-xL. (B) Represents relative expressions of proteins with control expressions set at 100%. The data are presented as mean±SD, n=6. aStatistically significant difference at p<0.05 vs respective control. b–eSignificant differences (p<0.05) between mean values as determined by one-way analysis of variance (ANOVA) followed by Duncan's multiple range test (Lane 1, Control; Lane 2, Isoflurane; Lane 3, Isoflurane+20 mg Genistein; Lane 4, Isoflurane+40 mg Genistein; Lane 5, Isoflurane +80 mg Genistein).
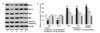
Fig. 3
Genistein effectively upregulated CREB signalling.
(A) Expression analysis by Western blotting revealed up-regulated CREB signaling in the hippocampal tissues on geinstein supplementation. Genistein significantly (p<0.05) increased isoflurane suppressed CREB phosphorylation and CaMKIV expression. (B) Represents relative expressions of proteins with control expressions set at 100%.The data are presented as mean±SD, n=6. aStatistically significant differences at p<0.05 vs respective control. b–eSignificant differences (p<0.05) between mean values as determined by one-way analysis of variance (ANOVA) followed by Duncan's multiple range test (Lane 1, Control; Lane 2, Isoflurane; Lane 3, Isoflurane + 20 mg Genistein; Lane 4, Isoflurane + 40 mg Genistein; Lane 5, Isoflurane + 80 mg Genistein).

Fig. 4
Genistein increased hippocampal cAMP levels.
cAMP levels in the hippocampal tissues following isoflurane exposure was assessed by ELISA. Genistein was found to cause significant (p<0.05) increase in the cAMP levels in a dose-dependent manner. The data are presented as mean±SD, n=6. aStatistically significant difference at p<0.05 vs respective control. b–eSignificant differences (p<0.05) between mean values as determined by one-way analysis of variance (ANOVA) followed by Duncan's multiple range test.
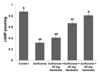
Fig. 5
Genistein up-regulated BDNF –TrkB signaling.
The BDNF and TrkB expressions in the hippocampal tissues were assessed both at mRNA and as well protein levels. Genistein dose-dependently increased the expression of BDNF and TrkB at both (A and B) mRNA and (C and D) protein levels. (C and D) Geinstein increased phosphorylation of TrkB. (B and D) Represents relative expressions of proteins with control expressions set at 100%. the data are presented as mean±SD, n=6. aStatistically significant difference at p<0.05 vs respective control. b–fSignificant differences (p<0.05) between mean values as determined by one-way analysis of variance (ANOVA) followed by Duncan's multiple range test (Lane 1, Control; Lane 2, Isoflurane; Lane 3, Isoflurane+20 mg Genistein; Lane 4, Isoflurane + 40 mg Genistein; Lane 5, Isoflurane+80 mg Genistein).
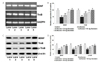
Fig. 6
Genistein modulated the expression of PI3K/Akt pathway proteins.
(A) Western blot analysis of the PI3K pathway proteins revealed that genistein upregulated the expression of Akt, GSK-3β, phospho-Akt and phospho- GSK-3β and mTORc1 while suppressed PTEN, signifying activation of PI3K/Akt signaling cascade. (B) The data are presented as mean±SD, n=6. aStatistically significant difference at p<0.05 vs respective control. b–fSignificant differences (p<0.05) between mean values as determined by one-way analysis of variance (ANOVA) followed by Duncan's multiple range test (Lane 1, Control; Lane 2, Isoflurane; Lane 3, Isoflurane+20 mg Genistein; Lane 4, Isoflurane+40 mg Genistein; Lane 5, Isoflurane+80 mg Genistein).
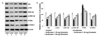
Fig. 7
Geinstein improved the general behavior of P35 rats following isoflurane exposure at P7.
(A) The behavior of the rats in a novel environment was assessed by open-field test. Geinstein treatment improved the movement of the rats in comparison with isoflurane-alone exposed rats. (B) Genistein enhanced the freezing responses of P35 rats in context and cued fear conditioning tests. The data are presented as mean±SD, n=6. aStatistically significant difference at p<0.05 vs respective control. b–fSignificant differences (p<0.05) between mean values as determined by one-way analysis of variance (ANOVA) followed by Duncan's multiple range test.
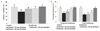
Fig. 8
Geinstein effectively improved learning and memory of rats in the Morris water maze tests.
(A) The escape latency of the rats during training sessions reveals that geinstein treatment reduced the latency time. (B) Genistein treatment enhanced the performance of the rats in identifying the submerged platform at a shorter latency time demonstrating effectively improved spatial navigation in cued and place trials. In probe trials geinstein-treated rats spent longer time looking out for the platform in the target quadrant in comparison with isoflurane-alone exposed rats, indicating improved memory retention on geinstein treatment. The data are presented as means±SD, n=6. aStatistically significant at p<0.05 vs respective control. b–fSignificant differences (p<0.05) between mean values as determined by one-way analysis of variance (ANOVA) followed by Duncan's multiple range test.
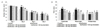
Notes
References
1. Jevtovic-Todorovic V, Hartman RE, Izumi Y, Benshoff ND, Dikranian K, Zorumski CF, Olney JW, Wozniak DF. Early exposure to common anesthetic agents causes widespread neurodegeneration in the developing rat brain and persistent learning deficits. J Neurosci. 2003; 23:876–882.
2. Brambrink AM, Evers AS, Avidan MS, Farber NB, Smith DJ, Zhang X, Dissen GA, Creeley CE, Olney JW. Isoflurane-induced neuroapoptosis in the neonatal rhesus macaque brain. Anesthesiology. 2010; 112:834–841.
3. Kong F, Xu L, He D, Zhang X, Lu H. Effects of gestational isoflurane exposure on postnatal memory and learning in rats. Eur J Pharmacol. 2011; 670:168–174.
4. Li Y, Liu C, Zhao Y, Hu K, Zhang J, Zeng M, Luo T, Jiang W, Wang H. Sevoflurane induces short-term changes in proteins in the cerebral cortices of developing rats. Acta Anaesthesiol Scand. 2013; 57:380–390.
5. Li Y, Wang F, Liu C, Zeng M, Han X, Luo T, Jiang W, Xu J, Wang H. JNK pathway may be involved in isoflurane-induced apoptosis in the hippocampi of neonatal rats. Neurosci Lett. 2013; 545:17–22.
6. Flick RP, Katusic SK, Colligan RC, Wilder RT, Voigt RG, Olson MD, Sprung J, Weaver AL, Schroeder DR, Warner DO. Cognitive and behavioral outcomes after early exposure to anesthesia and surgery. Pediatrics. 2011; 128:e1053–e1061.
7. Gentry KR, Steele LM, Sedensky MM, Morgan PG. Early developmental exposure to volatile anesthetics causes behavioral defects in Caenorhabditis elegans. Anesth Analg. 2013; 116:185–189.
8. Head BP, Patel HH, Niesman IR, Drummond JC, Roth DM, Patel PM. Inhibition of p75 neurotrophin receptor attenuates isoflurane-mediated neuronal apoptosis in the neonatal central nervous system. Anesthesiology. 2009; 110:813–825.
9. Xiong WX, Zhou GX, Wang B, Xue ZG, Wang L, Sun HC, Ge SJ. Impaired spatial learning and memory after sevoflurane-nitrous oxide anesthesia in aged rats is associated with down-regulated cAMP/CREB signaling. PLoS One. 2013; 8:e79408.
10. Wu J, Zhang M, Li H, Sun X, Hao S, Ji M, Yang J, Li K. BDNF pathway is involved in the protective effects of SS-31 on isoflurane-induced cognitive deficits in aging mice. Behav Brain Res. 2016; 305:115–121.
11. Bourtchuladze R, Frenguelli B, Blendy J, Cioffi D, Schutz G, Silva AJ. Deficient long-term memory in mice with a targeted mutation of the cAMP-responsive element-binding protein. Cell. 1994; 79:59–68.
12. Impey S, Mark M, Villacres EC, Poser S, Chavkin C, Storm DR. Induction of CRE-mediated gene expression by stimuli that generate long-lasting LTP in area CA1 of the hippocampus. Neuron. 1996; 16:973–982.
13. Nakagawa S, Kim JE, Lee R, Chen J, Fujioka T, Malberg J, Tsuji S, Duman RS. Localization of phosphorylated cAMP response element-binding protein in immature neurons of adult hippocampus. J Neurosci. 2002; 22:9868–9876.
14. Fujioka T, Fujioka A, Duman RS. Activation of cAMP signaling facilitates the morphological maturation of newborn neurons in adult hippocampus. J Neurosci. 2004; 24:319–328.
15. Ao H, Ko SW, Zhuo M. CREB activity maintains the survival of cingulate cortical pyramidal neurons in the adult mouse brain. Mol Pain. 2006; 2:15.
16. Schneider HH. Brain cAMP response to phosphodiesterase inhibitors in rats killed by microwave irradiation or decapitation. Biochem Pharmacol. 1984; 33:1690–1693.
17. Li YF, Huang Y, Amsdell SL, Xiao L, O'Donnell JM, Zhang HT. Antidepressant- and anxiolytic-like effects of the phosphodiesterase-4 inhibitor rolipram on behavior depend on cyclic AMP response element binding protein-mediated neurogenesis in the hip pocampus. Neuropsychopharmacology. 2009; 34:2404–2419.
18. Brightwell JJ, Smith CA, Neve RL, Colombo PJ. Long-term memory for place learning is facilitated by expression of cAMP response element-binding protein in the dorsal hippocampus. Learn Mem. 2007; 14:195–199.
19. Rosenegger D, Parvez K, Lukowiak K. Enhancing memory formation by altering protein phosphorylation balance. Neurobiol Learn Mem. 2008; 90:544–552.
20. Zhang F, Zhu ZQ, Liu DX, Zhang C, Gong QH, Zhu YH. Emulsified isoflurane anesthesia decreases brain-derived neurotrophic factor expression and induces cognitive dysfunction in adult rats. Exp Ther Med. 2014; 8:471–477.
21. Minichiello L. TrkB signalling pathways in LTP and learning. Nat Rev Neurosci. 2009; 10:850–860.
22. Park H, Poo MM. Neurotrophin regulation of neural circuit development and function. Nat Rev Neurosci. 2013; 14:7–23.
23. Huang EJ, Reichardt LF. Trk receptors: roles in neuronal signal transduction. Annu Rev Biochem. 2003; 72:609–642.
24. Reichardt LF. Neurotrophin-regulated signalling pathways. Philos Trans R Soc Lond B Biol Sci. 2006; 361:1545–1564.
25. Takei N, Kawamura M, Hara K, Yonezawa K, Nawa H. Brain-derived neurotrophic factor enhances neuronal translation by activating multiple initiation processes: comparison with the effects of insulin. J Biol Chem. 2001; 276:42818–42825.
26. Horwood JM1, Dufour F, Laroche S, Davis S. Signalling mechanisms mediated by the phosphoinositide 3-kinase/Akt cascade in synaptic plasticity and memory in the rat. Eur J Neurosci. 2006; 23:3375–3384.
27. Chiang HC, Wang L, Xie Z, Yau A, Zhong Y. PI3 kinase signaling is involved in Abeta-induced memory loss in Drosophila. Proc Natl Acad Sci U S A. 2010; 107:7060–7065.
28. Mizuno M, Yamada K, Takei N, Tran MH, He J, Nakajima A, Nawa H, Nabeshima T. Phosphatidylinositol 3-kinase: a molecule mediating BDNF-dependent spatial memory formation. Mol Psychiatry. 2003; 8:217–224.
29. Pontén E, Fredriksson A, Gordh T, Eriksson P, Viberg H. Neonatal exposure to propofol affects BDNF but not CaMKII, GAP-43, synaptophysin and tau in the neonatal brain and causes an altered behavioural response to diazepam in the adult mouse brain. Behav Brain Res. 2011; 223:75–80.
30. Gętek M, Czech N, Muc-Wierzgoń M, Grochowska-Niedworok E, Kokot T, Nowakowska-Zajdel E. The active role of leguminous plant components in type 2 diabetes. Evid Based Complement Alternat Med. 2014; 2014:293961.
31. Xu SZ, Zhong W, Ghavideldarestani M, Saurabh R, Lindow SW, Atkin SL. Multiple mechanisms of soy isoflavones against oxidative stress-induced endothelium injury. Free Radic Biol Med. 2009; 47:167–175.
32. Behloul N, Wu G. Genistein: a promising therapeutic agent for obesity and diabetes treatment. Eur J Pharmacol. 2013; 698:31–38.
33. Ganai AA, Khan AA, Malik ZA, Farooqi H. Genistein modulates the expression of NF-κB and MAPK (p-38 and ERK1/2), thereby attenuating d-Galactosamine induced fulminant hepatic failure in Wistar rats. Toxicol Appl Pharmacol. 2015; 283:139–146.
34. Su P, Zhang J, Wang S, Aschner M, Cao Z, Zhao F, Wang D, Chen J, Luo W. Genistein alleviates lead-induced neurotoxicity in vitro and in vivo: Involvement of multiple signaling pathways. Neurotoxicology. 2016; 53:153–164.
35. Spoerlein C, Mahal K, Schmidt H, Schobert R. Effects of chrysin, apigenin, genistein and their homoleptic copper(II) complexes on the growth and metastatic potential of cancer cells. J Inorg Biochem. 2013; 127:107–115.
36. Orliaguet G, Vivien B, Langeron O, Bouhemad B, Coriat P, Riou B. Minimum alveolar concentration of volatile anesthetics in rats during postnatal maturation. Anesthesiology. 2001; 95:734–739.
37. Wu J, Dong L, Zhang M, Jia M, Zhang G, Qiu L, Ji M, Yang J. Class I histone deacetylase inhibitor valproic acid reverses cognitive deficits in a mouse model of septic encephalopathy. Neurochem Res. 2013; 38:2440–2449.
38. Ji M, Dong L, Jia M, Liu W, Zhang M, Ju L, Yang J, Xie Z, Yang J. Epigenetic enhancement of brain-derived neurotrophic factor signaling pathway improves cognitive impairments induced by isoflurane exposure in aged rats. Mol Neurobiol. 2014; 50:937–944.
39. Kim JJ, Fanselow MS. Modality-specific retrograde amnesia of fear. Science. 1992; 256:675–677.
40. Lin D, Zuo Z. Isoflurane induces hippocampal cell injury and cognitive impairments in adult rats. Neuropharmacology. 2011; 61:1354–1359.
41. Satomoto M, Satoh Y, Terui K, Miyao H, Takishima K, Ito M, Imaki J. Neonatal exposure to sevoflurane induces abnormal social behaviors and deficits in fear conditioning in mice. Anesthesiology. 2009; 110:628–637.
42. Istaphanous GK, Howard J, Nan X, Hughes EA, McCann JC, McAuliffe JJ, Danzer SC, Loepke AW. Comparison of the neuroapoptotic properties of equipotent anesthetic concentrations of desflurane, isoflurane, or sevoflurane in neonatal mice. Anesthesiology. 2011; 114:578–587.
43. Dong Y, Zhang G, Zhang B, Moir RD, Xia W, Marcantonio ER, Culley DJ, Crosby G, Tanzi RE, Xie Z. The common inhalational anesthetic sevoflurane induces apoptosis and increases beta-amyloid protein levels. Arch Neurol. 2009; 66:620–631.
44. Culley DJ, Baxter MG, Yukhananov R, Crosby G. Long-term impairment of acquisition of a spatial memory task following isoflurane-nitrous oxide anesthesia in rats. Anesthesiology. 2004; 100:309–314.
45. DiMaggio CJ, Sun L, Kakavouli A, Li G. Exposure to anesthesia and the risk of developmental and behavioral disorders in young children. Anesthesiology. 2008; 109:A1415.
46. Wilder RT, Flick RP, Sprung J, Katusic SK, Barbaresi WJ, Mickelson C, Gleich SJ, Schroeder DR, Weaver AL, Warner DO. Early exposure to anesthesia and learning disabilities in a population-based birth cohort. Anesthesiology. 2009; 110:796–804.
47. Sakamoto K, Karelina K, Obrietan K. CREB: a multifaceted regulator of neuronal plasticity and protection. J Neurochem. 2011; 116:1–9.
48. Kida S, Serita T. Functional roles of CREB as a positive regulator in the formation and enhancement of memory. Brain Res Bull. 2014; 105:17–24.
49. Gonzalez GA, Montminy MR. Cyclic AMP stimulates somatostatin gene transcription by phosphorylation of CREB at serine 133. Cell. 1989; 59:675–680.
50. Gonzalez GA, Yamamoto KK, Fischer WH, Karr D, Menzel P, Biggs W 3rd, Vale WW, Montminy MR. A cluster of phosphorylation sites on the cyclic AMP-regulated nuclear factor CREB predicted by its sequence. Nature. 1989; 337:749–752.
51. Carlezon WA Jr, Duman RS, Nestler EJ. The many faces of CREB. Trends Neurosci. 2005; 28:436–445.
52. Yamashima T. 'PUFA-GPR40-CREB signaling' hypothesis for the adult primate neurogenesis. Prog Lipid Res. 2012; 51:221–231.
53. Dudek H, Datta SR, Franke TF, Birnbaum MJ, Yao R, Cooper GM, Segal RA, Kaplan DR, Greenberg ME. Regulation of neuronal survival by the serine-threonine protein kinase Akt. Science. 1997; 275:661–665.
54. Brunet A, Datta SR, Greenberg ME. Transcription-dependent and -independent control of neuronal survival by the PI3K-Akt signaling pathway. Curr Opin Neurobiol. 2001; 11:297–305.
55. Ma D, Williamson P, Januszewski A, Nogaro MC, Hossain M, Ong LP, Shu Y, Franks NP, Maze M. Xenon mitigates isoflurane-induced neuronal apoptosis in the developing rodent brain. Anesthesiology. 2007; 106:746–753.
56. Hsu SY, Kaipia A, Zhu L, Hsueh AJ. Interference of BAD (Bcl-xL/ Bcl-2-associated death promoter)-induced apoptosis in mammalian cells by 14-3-3 isoforms and P11. Mol Endocrinol. 1997; 11:1858–1867.
57. Zhu YM, Wang CC, Chen L, Qian LB, Ma LL, Yu J, Zhu MH, Wen CY, Yu LN, Yan M. Both PI3K/Akt and ERK1/2 pathways participate in the protection by dexmedetomidine against transient focal cerebral ischemia/reperfusion injury in rats. Brain Res. 2013; 1494:1–8.
58. Koh PO. Nicotinamide attenuates the ischemic brain injury-induced decrease of Akt activation and Bad phosphorylation. Neurosci Lett. 2011; 498:105–109.
59. Amano H, Maruyama IN. Aversive olfactory learning and associative long-term memory in Caenorhabditis elegans. Learn Mem. 2011; 18:654–665.
60. Kandel ER. The molecular biology of memory: cAMP, PKA, CRE, CREB-1, CREB-2, and CPEB. Mol Brain. 2012; 5:14.
61. Merz K, Herold S, Lie DC. CREB in adult neurogenesis--master and partner in the development of adult-born neurons? Eur J Neurosci. 2011; 33:1078–1086.
62. Sheng M, Thompson MA, Greenberg ME. CREB: a Ca2+-regulated transcription factor phosphorylated by calmodulin-dependent kinases. Science. 1991; 252:1427–1430.
63. Finkbeiner S, Tavazoie SF, Maloratsky A, Jacobs KM, Harris KM, Greenberg ME. CREB: a major mediator of neuronal neurotrophin responses. Neuron. 1997; 19:1031–1047.
64. Henriksson BG, Söderström S, Gower AJ, Ebendal T, Winblad B, Mohammed AH. Hippocampal nerve growth factor levels are related to spatial learning ability in aged rats. Behav Brain Res. 1992; 48:15–20.
65. Bromley-Brits K, Deng Y, Song W. Morris water maze test for learning and memory deficits in Alzheimer's disease model mice. J Vis Exp. 2011; DOI: 10.3791/2920.