Abstract
Shepherd's purse, Capsella bursa-pastoris (L.) Medik., has been considered a health food for centuries in Asia and is known to contain the isothiocyanate compound sulforaphane. In this study, we evaluated the anti-inflammatory and antibacterial properties of a sulforaphane-containing solution (SCS) isolated from shepherd's purse. SCS had significant anti-inflammatory activity indicated by the decreased levels of nitric oxide (NO), cytokines (interleukin 1β [IL-1β], IL-6, and IL-10), and prostaglandin E2 (PGE2) in lipopolysaccharide-stimulated RAW 264.7 murine macrophages. In addition, SCS decreased the inducible NO synthase (iNOS) and cyclooxygenase 2 (COX-2) levels, which confirmed the anti-inflammatory activity of SCS. Further, SCS inhibited vancomycin-resistant enterococci (VRE) and Bacillus anthracis. The minimal inhibitory concentration was 250 µg/ml for VRE and 1,000 µg/ml for B. anthracis. Taken together, these data indicate that SCS has potential anti-inflammatory and anti-superbacterial properties, and thus it can be used as a functional food or pharmaceutical.
Shepherd's purse (Capsella bursa-pastoris [L.] Medik.) is one of the most abundant flowering plants worldwide [1,2]. Shepherd's purse is a member of the Brassicaceae family and is closely related to the genetic model plant Arabidopsis thaliana. Although it is an abundant weed during early spring, shepherd's purse has been used as a food and folk medicine for centuries in Asian and European countries. Moreover, this plant has traditionally been used as a diuretic and for the treatment of dysentery, furuncle, gonorrhea, menstrual disorders, and fever [3,4]. In particular, plants belonging the Brassicaceae family contain high levels of sulforaphane (SF). SF is an isothiocyanate, and SF has received extensive attention for its potent chemopreventive activity [5,6]. SF is not only effective in preventing chemically induced cancers in animal models [6-9], but also effective in inhibiting the growth of established tumors [10,11]. In addition, a sulforaphane-containing solution (SCS) isolated from broccoli had an antioxidant effect [12]. Furthermore, shepherd's purse contains vitamin A, ascorbic acid, linoleic acid, and omega-3 polyunsaturated fatty acids, which are beneficial for human health [13-15]. To date, several classes of phenolic compounds have been isolated from shepherd's purse, such as flavonoids, alkaloids, calystegines, glucosinolates, and saponins [16-19].
Inflammation is an important process in the pathogenesis of a variety of diseases. Macrophages are immune cells that are critical for the regulation of inflammatory responses. Activation of macrophages leads to secretion of a number of various inflammatory mediators, including interleukin-1β (IL-1β), IL-6, IL-10 prostaglandin E2 (PGE2), and nitric oxide (NO) [20-22]. Cytokines such as IL-1β, IL-6, and IL-10, are soluble proteins secreted by cells of the immune system, which alter the properties of different cell types and provide essential communication signals for motile immune cells [23]. PGs are generated by a variety of cell types, including activated macrophages. The rate-limiting enzyme in PG synthesis is cyclooxygenase (COX). Expression of the COX-2 isoform is induced by several stimuli, including growth factors, mitogens, cytokines, and tumor promoters. The uncontrolled activity of COX-2 is thought to play a major role in the pathogenesis of a host of chronic inflammatory disorders [24]. NO is produced by 3 distinct nitric oxide synthase (NOS) isoforms, and inducible NOS (iNOS) the most essential isoform involved in the regulation of inflammatory responses [25,26].
Currently, the emergence of multiple-antimicrobial-resistant bacteria such as vancomycin-resistant enterococci (VRE) and bioterrorism agents, namely Bacillus anthracis, represent a serious worldwide health crisis [27,28]. VRE were first reported in 1986 and pose a major problem in many countries because of the difficulty of treating and managing infections [29]. B. anthracis (often referred to as "anthrax"), which is one of the most notorious biological agents, has been widely used as a weapon for the intent to cause substantial morbidity and mortality, which can potentially cripple an entire city or region [30].
Although a number of studies have been performed to assess the medicinal efficacy of SCS, its anti-inflammatory and anti-superbacterial effects are currently unknown. In this study, we investigated the impact of SCS on the production of IL-1β, IL-6, IL-10, NO, PGE2, iNOS, and COX-2 in lipopolysaccharide (LPS)-stimulated RAW 264.7 cells and its anti-bacterial effects against multiple-antimicrobial-resistant bacteria to determine whether it could be used as a functional food and pharmaceutical.
Shepherd's purse plant material (500 g) was ground for 10 s in a Tekmar analytical grinder cooled with pure water. The plant material was defatted 3 times with excess hexane and allowed to dry overnight in a fume hood. Then, glucosinolates were hydrolyzed by adding water in a ratio of 3 : 1 water/shepherd's purse (w/w), and the mixture was allowed to autolyse for 8 h at room temperature. Sodium chloride, wet plant, and sodium sulfate were combined in the ratio of 1 : 1 : 0.75 (w/w/w) and mixed thoroughly. This paste was extracted three times with an equal volume of excess ethanol, which was combined and dried at 32℃ under vacuum in a rotary evaporator. The resulting residue was dissolved in 120 ml of 5% ethanol in water (v/v) and washed 3 times with excess hexane to remove nonpolar contaminants. The aqueous phase was then filtered through a 0.22-µm membrane filter in preparation for injection onto HPLC [31].
RAW 264.7 murine macrophages were acquired from the Korea Cell Bank (Seoul, Korea) and cultured in Dulbecco's minimum essential medium (DMEM; Lonza, Walkersville, MD, USA) containing 10% heat-inactivated fetal bovine serum (FBS; GIBCO BRL, Grand Island, NY, USA) and 20 µg/ml gentamycin (GIBCO, China) at 37℃ in a water-jacketed CO2 incubator (Model 3111; Thermo Fisher Scientific, Waltham, MA, USA). Cell viability was determined using 0, 1, 5, 10, 50, 100, and 500 µg/ml samples of SCS. RAW 264.7 cells (5×105 cells/well) were seeded in 24-well plates. After 24 h treatments, the cells were subjected to 3-(4,5-dimethylthiazol-2-yl)-2,5-diphenyltetrazolium bromide (MTT) assay. Media were replaced by 500 µl of fresh serum-free media containing 0.5 mg/ml MTT (Sigma-Aldrich, St. Louis, MO, USA). After incubation for 1 h (37℃, 5% CO2), MTT-containing media were removed, and the reduced formazan dye was solubilized by adding 500 µl of dimethyl sulfoxide (DMSO) to each well. After gentle mixing, the absorbance was monitored at 590 nm by using a microplate reader (Tecan, Männedorf, Switzerland).
Cells cytotoxicity was determined using 0, 1, 5, 10, 50, 100, and 500 µg/ml of SCS. RAW 264.7 cells were plated at a density of 2×104 cells in 96-well cell culture plates with 100 µl of culture medium and incubated for 24 h. Cells was evaluated by measuring the release of the enzyme LDH using a LDH cytotoxicity Detection kit (Takara Bio Inc., Shiga, Japan). A value for maximal LDH release was obtained from the supernatant of cells treated with 1% Triton X-100 (Sigma-Aldrich). The percentage of LDH release was calculated using the following equation: 100×(experimental LDH release-spontaneous LDH release)/(maximal LDH release-spontaneous LDH release).
RAW 264.7 cells were plated at a density of 5×105 cells in 24-well cell culture plates with 1 ml of culture medium and incubated for 24 h. Then, the cells were treated with 0, 1, 5, 10, 50, 100, and 500 µg/ml SCS in LPS (0.1 µg/ml) and incubated for an additional 24 h. The quantity of nitrite generated was measured using the Griess reagent system (Sigma-Aldrich). Equal volumes of culture supernatant and Griess reagent were mixed and incubated for 10 min at room temperature. Absorbance was measured at 540 nm by using a spectrophotometer and was compared to the absorbance of a nitrite standard curve to determine the nitrite concentration in the supernatants.
The levels of IL-1β, 6, 10, and PGE2 in the supernatants from macrophage cultures were determined using an enzyme-linked immunosorbent assay (ELISA) kit according to manufacturer's instructions (R&D Systems, Minneapolis, MN, USA). RAW 264.7 cells were incubated with LPS (0.1 µg/ml) in the presence of different concentrations of SCS for 24 h. The supernatants were collected and stored at -80℃ until analysis.
RAW 264.7 cells were incubated with LPS (0.1 µg/ml) in the presence of different concentrations of SCS for 24 h. Total protein was extracted from cultured cells using the freeze-thaw lysis method. Equivalent total protein samples were separated using polyacrylamide gel electrophoresis (PAGE) on a 12% sodium dodecyl sulfate (SDS) gel and blotted onto polyvinylidene fluoride (PVDF) membranes (BioRad, Hercules, CA, USA). After blotting, the membrane was incubated overnight with specific primary antibodies at 4℃, and then incubated for 1 h with secondary antibodies. The membranes were exposed to Hyperfilm ECL (Amersham Biosciences, Buckinghamshire, UK) for visualization.
RAW 264.7 cells were incubated with LPS (0.1 µg/ml) in the presence of different concentrations of SCS for 24 h. Total RNA was extracted from cultured cells by using the Trizol method (Gibco BRL). Reverse transcription of total RNA was performed using AMV reverse transcriptase (PROMEGA, Madison, WI, USA). Real-time polymerase chain reaction (RT-PCR) assay was performed and monitored using SYBR Green chemistry with a 7500 Fast Realtime PCR System (Applied Biosystems, Foster City, CA, USA). All reactions were performed in triplicate to confirm reproducibility. The quantity of mRNA in each sample was normalized using that for mean β-actin levels. Primer sequences were as follows: iNOS, forward primer 5'-TCCTACACCACACCAAAC-3', reverse primer 5'-CTCCAATCTCTGCCTATCC-3'; COX-2, forward primer 5'-CCTCTGCGATGCTCTTCC-3', reverse primer 5'-TCACACTTATACTGGTCAAATCC-3' [32]; and β-actin, forward primer 5'-TGAGAGGGAAATCGTGCGTGAC-3', reverse primer 5'-GCTCGTTGCCAATAGTGATGACC-3' [33].
Antimicrobial activity against 2 microorganisms was primarily determined using the agar disc diffusion method using the diameter of the clear zone. VRE CAU 8105 was isolated in our laboratory. B. anthracis ATCC 14578T was obtained from the American Type Culture Collection (ATCC; Manassas, VA, USA). These strains were grown aerobically on Mueller Hinton agar (Difco, Detroit, MI, USA) at 37℃ for 18 h. Media from test microorganism cultures were added to sterilized paper discs (10 mm), and these discs were placed on the inoculated agar surface. Media from isolated bacterial cultures were used to fill in the paper discs (100 µl/disc). After pre-diffusion at room temperature for 1 h, the plates were incubated at 37℃ for 19 h. Discs with non-cultured-media were used as negative controls.
A broth microdilution susceptibility assay was performed in accordance with National Committee for Clinical Laboratory Standards (NCCLS, 2009) guidelines for the determination of minimal inhibitory concentrations (MICs). All tests were performed using Mueller Hinton agar (Difco), and the strains were cultured overnight at 37℃. Bacterial suspensions were diluted to match the 0.5 MacFarland standards (approximately 1.5×108 CFU/ml). The purified SCS was dissolved in DMSO, and two-fold serial dilutions were prepared and added to brain heart infusion (BHI) media (final concentration range, 3.9~1,000 µg/ml). Culture media were incubated under normal atmospheric conditions for 24 h at 37℃. Bacterial growth was indicated by the presence of a white pellet on the bottom of the tube. The MIC value was defined as the lowest SCS concentration at which no visible growth was observed.
The sulforaphane concentrations in the Shepherd's purse plant was 53.7 µg/g (0.000537%). The cytotoxic effects of SCS (up to a concentration of 500 µg/ml) were not apparent after incubation for 24 h in RAW 264.7 cells by using the MTT assay (Fig. 1A). In addition, RAW 264.7 cells did not increase LDH levels from any of the concentration tested (Fig. 1B).
The inhibitory effects of SCS were evaluated by assessing the production of nitrite, a stable metabolite of NO, and PGE2 in LPS-stimulated RAW 264.7 cells treated with the SCS. SCS effectively suppressed LPS-induced nitrite production in a dose-dependent manner (Fig. 2). Consistent with these observations, SCS markedly inhibited PGE2 production. NO production was significantly lower in the groups treated with 50 and 100 µg/ml of SCS than in the control group. The NO levels in LPS-stimulated RAW 264.7 cells exposed to 50 µg/ml SCS were 40±2% of control values. PGE2 production after treatment with 100 µg/ml SCS was 35±5% of the controls. These results show that SCS exerts an anti-inflammatory effect by ameliorating the production of inflammatory mediators, including NO and PGE2.
We examined the production of IL-1β, IL-6, and IL-10 (major pro-inflammatory cytokines) in LPS-stimulated RAW 264.7 cells treated with SCS. The production of IL-1β, IL-6, and IL-10 was significantly reduced after SCS treatment (Fig. 3). The IL-1β concentration in LPS-stimulated RAW 264.7 cells exposed to 1, 5, 10, 50, 100, or 500 µg/ml SCS was 113.5±2.2 pg/ml, 114.6±5.7 pg/ml, 96.5±4.1 pg/ml, 84.1±1.8 pg/ml, 60.7±3.6 pg/ml, and 10.6±3.3 pg/ml, respectively. The corresponding concentrations of IL-6 were 1015.9±1.7 pg/ml, 979.5±3.4 pg/ml, 950.1±0.8 pg/ml, 395.1±2.9 pg/ml, 312.9±3.2 pg/ml, and 63.8±0.1 pg/ml. The corresponding concentrations of IL-10 were 167.9±8.9 pg/ml, 159.0±9.9 pg/ml, 152.2±8.4 pg/ml, 93.9±4.1 pg/ml, 53.6±1.8 pg/ml, and 23.8±1.6 pg/ml (Fig. 3).
SCS decreased the expression of iNOS and COX-2 in a concentration-dependent manner, and was marked by inhibitory effects were observed at the highest concentration tested (50 µg/ml; Fig. 4).
The iNOS and COX-2 mRNA levels were determined using qRT-PCR to confirm the inhibitory effects of SCS on NO and PGE2 production in LPS-stimulated RAW 264.7 cells. Similar to the dose-dependent decrease in NO, iNOS and COX-2 mRNA expression was reduced (Fig. 2 and 5). Treatment with 1, 5, 10, 50, 100, and 500 µg/ml SCS decreased iNOS mRNA levels in a dose-dependent manner by 49, 62, 70, 78, 87, and 93%, respectively, relative to the corresponding iNOS levels in LPS-treated positive control cells. In addition, our data showed that 1, 5, 10, 50, 100, and 500 µg/ml SCS decreased COX-2 mRNA levels by 58, 79, 78, 70, 71, and 99%, respectively, relative to these levels in LPS-treated positive control cells.
SCS effectively inhibited VRE and B. anthracis (Table 1). The disc diffusion values corresponding to 14 mg/ml SCS treatment are provided for VRE and B. anthracis. The diameters for the different strains were 16, 19, 29, 23, 19, and 14 mm, and 17, 15, 15, and 14 mm after exposure for 18 h. The MICs of the SCS against VRE and B. anthracis strains are shown in Table 2. The MIC was 250 µg/ml for VRE and 1,000 µg/ml for B. anthracis.
Shepherd's purse has potent superoxide radical-scavenging activity [34]. Additionally, it possesses several medicinal properties such as anti-coagulant, anti-cancer, anti-thrombin, wound-healing, and anti-oxidant activities and is a potential therapeutic agent for treating diabetes and fever [15,35-37]. In addition, SF also inhibits cancer growth in vitro and in experimental animals [38,39]. In addition to shepherd's purse, plants belonging to the Brassicaceae family, such as broccoli, cabbage, and radish, also contain high amounts of SF [40,41]. Thus, many studies have investigated materials from these plants. In particular, SF is a natural isothiocyanate found in plants belonging to the Brassicaceae family and has cancer chemopreventive activity [5,6]. However, to our knowledge, this is the first study in which the anti-inflammatory and anti-bacterial activity of SF isolated from shepherd's purse has been examined.
The well-known immune stimulant LPS is known to induce several intracellular signaling pathways in monocytes/macrophages and also affects the production of a variety of inflammatory cytokines and mediators. LPS causes dramatic pathophysiological reactions via production of inflammatory mediators NO and PGE2, pro-inflammatory cytokines IL-1β, IL-6, and IL-10, and by induction of iNOS and COX-2 [25,26,42]. Pro-inflammatory cytokines and mediators regulate the functional activity of immune cells and are involved in the pathogenesis of a host of inflammatory diseases, including septic shock, hemorrhagic shock, multiple sclerosis, rheumatoid arthritis, ulcerative colitis, and atherosclerosis [43-46]. Inhibiting the production and biological activities of iNOS, and COX-2 by selective inhibitors, neutralizing antibodies, or gene targeting results in significant amelioration of the development of rheumatoid arthritis, cardiovascular disease, and septic shock [47-50]. Therefore, drugs that suppress or inhibit the expression of these inflammation-associated genes are considered to harbor therapeutic potential for the prevention of inflammatory reactions and diseases. In this study, we showed that SCS downregulates NO, PGE2, IL-1β, IL-6, and IL-10 production in LPS-stimulated RAW 264.7 cells.
SCS was effective against superbacteria such as VRE and B. anthracis. Generally, the most serious infections caused by superbacteria develop in severely immunocompromised patients. The most common nosocomial infections produced by VRE are urinary tract infections followed by intra-abdominal and pelvic infections. They also cause surgical wound infections, bacteremia, endocarditis, neonatal sepsis, and in rare cases, meningitis [27,51]. Anthrax is an acute infection caused by B. anthracis. There are 3 forms of anthrax infection: cutaneous, inhalation, and gastrointestinal. In bioterrorism-related release of B. anthracis, in which people are intentionally exposed to the organism, contact with skin would be the most likely route of exposure [30]. Previously, sulforaphane reported to have antimicrobial effects against Helicobacter pylori and fungal pathogens, and also had an anticancer activity to stomach tumors [52-54]. However, mechanism of antimicrobial activities is not clear until now. Thus, further study is needed to elucidate functional mechanism of sulforaphane. Our present findings clearly indicate that SCS is a novel candidate for a functional food or therapeutic agent for control of inflammation and multi-drug resistant bacteria.
ACKNOWLEDGEMENTS
This research was supported by the Chung-Ang University Research Scholarship Grants in 2012.
References
1. Koch MA, Kiefer M. Genome evolution among cruciferous plants: a lecture from the comparison of the genetic maps of three diploid species--Capsella rubella, Arabidopsis lyrata subsp. petraea, and A. thaliana. Am J Bot. 2005; 92:761–767. PMID: 21652456.


2. Hurka H, Bleeker W, Neuffer B. Evolutionary processes associated with biological invasions in the brassicaceae. Biol Invasions. 2003; 5:281–292.


3. Kim H, Song MJ, Potter D. Medicinal efficacy of plants utilized as temple food in traditional Korean Buddhism. J Ethnopharmacol. 2006; 104:32–46. PMID: 16216457.


4. Zhou W. Ethnobotany of Capsella bursa-pastoris (L.) Medic. J Plant Resour Environ. 1998; 7:49–53.
5. Conaway CC, Wang CX, Pittman B, Yang YM, Schwartz JE, Tian D, McIntee EJ, Hecht SS, Chung FL. Phenethyl isothiocyanate and sulforaphane and their N-acetylcysteine conjugates inhibit malignant progression of lung adenomas induced by tobacco carcinogens in A/J mice. Cancer Res. 2005; 65:8548–8557. PMID: 16166336.


6. Fahey JW, Zhang Y, Talalay P. Broccoli sprouts: an exceptionally rich source of inducers of enzymes that protect against chemical carcinogens. Proc Natl Acad Sci U S A. 1997; 94:10367–10372. PMID: 9294217.


7. Fahey JW, Haristoy X, Dolan PM, Kensler TW, Scholtus I, Stephenson KK, Talalay P, Lozniewski A. Sulforaphane inhibits extracellular, intracellular, and antibiotic-resistant strains of Helicobacter pylori and prevents benzo[a]pyrene-induced stomach tumors. Proc Natl Acad Sci U S A. 2002; 99:7610–7615. PMID: 12032331.


8. Zhang Y, Kensler TW, Cho CG, Posner GH, Talalay P. Anticarcinogenic activities of sulforaphane and structurally related synthetic norbornyl isothiocyanates. Proc Natl Acad Sci U S A. 1994; 91:3147–3150. PMID: 8159717.


9. Chung FL, Conaway CC, Rao CV, Reddy BS. Chemoprevention of colonic aberrant crypt foci in Fischer rats by sulforaphane and phenethyl isothiocyanate. Carcinogenesis. 2000; 21:2287–2291. PMID: 11133820.


10. Singh AV, Xiao D, Lew KL, Dhir R, Singh SV. Sulforaphane induces caspase-mediated apoptosis in cultured PC-3 human prostate cancer cells and retards growth of PC-3 xenografts in vivo. Carcinogenesis. 2004; 25:83–90. PMID: 14514658.


11. Jackson SJ, Singletary KW. Sulforaphane: a naturally occurring mammary carcinoma mitotic inhibitor, which disrupts tubulin polymerization. Carcinogenesis. 2004; 25:219–227. PMID: 14578157.


12. Boddupalli S, Mein JR, Lakkanna S, James DR. Induction of phase 2 antioxidant enzymes by broccoli sulforaphane: perspectives in maintaining the antioxidant activity of vitamins a, C, and e. Front Genet. 2012; 3:7. PMID: 22303412.


13. Defelice MS. Shepherd's-purse, Capsella bursa-pastoris (L.) Medic. Weed Technol. 2001; 15:892–895.
14. Zennie TM, Ogzewalla D. Ascorbic acid and Vitamin A content of edible wild plants of Ohio and Kentucky. Economic Botany. 1977; 31:76–79.


15. Kweon MH, Kwak JH, Ra KS, Sung HC, Yang HC. Structural characterization of a flavonoid compound scavenging superoxide anion radical isolated from capsella bursa - pastoris. J Biochem Mol Biol. 1996; 29:423–428.
16. Song N, Xu W, Guan H, Liu X, Wang Y, Nie X. Several flavonoids from Capsella bursa-pastoris (L.) Medic. Asian J Trad Med. 2007; 2:218–222.
17. Brock A, Herzfeld T, Paschke R, Koch M, Dräger B. Brassicaceae contain nortropane alkaloids. Phytochemistry. 2006; 67:2050–2057. PMID: 16884746.


18. Cole PA. Association of canine splenic hemangiosarcomas and hematomas with nodular lymphoid hyperplasia or siderotic nodules. J Vet Diagn Invest. 2012; 24:759–762. PMID: 22621950.


19. Marquina JMG, Villa MG, Garriga AB. Fracción saponínica de la "Capsella bursa pastoris". An R Acad Nac Farmac. 1955; 21:49–60.
20. Boscá L, Zeini M, Través PG, Hortelano S. Nitric oxide and cell viability in inflammatory cells: a role for NO in macrophage function and fate. Toxicology. 2005; 208:249–258. PMID: 15691589.


21. Lawrence T, Willoughby DA, Gilroy DW. Anti-inflammatory lipid mediators and insights into the resolution of inflammation. Nat Rev Immunol. 2002; 2:787–795. PMID: 12360216.


22. Kaplanski G, Marin V, Montero-Julian F, Mantovani A, Farnarier C. IL-6: a regulator of the transition from neutrophil to monocyte recruitment during inflammation. Trends Immunol. 2003; 24:25–29. PMID: 12495721.


23. Ware CF. Network communications: lymphotoxins, LIGHT, and TNF. Annu Rev Immunol. 2005; 23:787–819. PMID: 15771586.


24. Harris SG, Padilla J, Koumas L, Ray D, Phipps RP. Prostaglandins as modulators of immunity. Trends Immunol. 2002; 23:144–150. PMID: 11864843.


26. Wu G, Morris SM Jr. Arginine metabolism: nitric oxide and beyond. Biochem J. 1998; 336:1–17. PMID: 9806879.


27. Moellering RC Jr. Vancomycin-resistant enterococci. Clin Infect Dis. 1998; 26:1196–1199. PMID: 9597252.


28. Boucher HW, Talbot GH, Bradley JS, Edwards JE, Gilbert D, Rice LB, Scheld M, Spellberg B, Bartlett J. Bad bugs, no drugs: no ESKAPE! An update from the Infectious Diseases Society of America. Clin Infect Dis. 2009; 48:1–12. PMID: 19035777.


29. Horiuchi K, Shiota S, Hatano T, Yoshida T, Kuroda T, Tsuchiya T. Antimicrobial activity of oleanolic acid from Salvia officinalis and related compounds on vancomycin-resistant enterococci (VRE). Biol Pharm Bull. 2007; 30:1147–1149. PMID: 17541170.


30. Inglesby TV, Henderson DA, Bartlett JG, Ascher MS, Eitzen E, Friedlander AM, Hauer J, McDade J, Osterholm MT, O'Toole T, Parker G, Perl TM, Russell PK, Tonat K. Working Group on Civilian Biodefense. Anthrax as a biological weapon: medical and public health management. JAMA. 1999; 281:1735–1745. PMID: 10328075.
31. Matusheski NV, Wallig MA, Juvik JA, Klein BP, Kushad MM, Jeffery EH. Preparative HPLC method for the purification of sulforaphane and sulforaphane nitrile from Brassica oleracea. J Agric Food Chem. 2001; 49:1867–1872. PMID: 11308338.


32. Pan MH, Lai CS, Wang YJ, Ho CT. Acacetin suppressed LPS-induced up-expression of iNOS and COX-2 in murine macrophages and TPA-induced tumor promotion in mice. Biochem Pharmacol. 2006; 72:1293–1303. PMID: 16949556.


33. Kim SJ. Curcumin suppresses the production of interleukin-6 in Prevotella intermedia lipopolysaccharide-activated RAW 264.7 cells. J Periodontal Implant Sci. 2011; 41:157–163. PMID: 21811692.
34. Hong JI, Ra KS, Yang HC. Free radical scavenging and antioxidative activities by ethanol extract from Capsella bursa-pastoris. Korean J Food Nutr. 1994; 7:169–176.
35. Goun EA, Petrichenko VM, Solodnikov SU, Suhinina TV, Kline MA, Cunningham G, Nguyen C, Miles H. Anticancer and antithrombin activity of Russian plants. J Ethnopharmacol. 2002; 81:337–342. PMID: 12127234.


36. Park CJ, Park CB, Hong SS, Lee HS, Lee SY, Kim SC. Characterization and cDNA cloning of two glycine- and histidine-rich antimicrobial peptides from the roots of shepherd's purse, Capsella bursa-pastoris. Plant Mol Biol. 2000; 44:187–197. PMID: 11117262.
37. Ivanova D, Gerova D, Chervenkov T, Yankova T. Polyphenols and antioxidant capacity of Bulgarian medicinal plants. J Ethnopharmacol. 2005; 96:145–150. PMID: 15588663.


38. Kuroda K, Akao M, Kanisawa M, Miyaki K. Inhibitory effect of Capsella bursa-pastoris extract on growth of Ehrlich solid tumor in mice. Cancer Res. 1976; 36:1900–1903. PMID: 1268843.
39. Li Y, Zhang T, Korkaya H, Liu S, Lee HF, Newman B, Yu Y, Clouthier SG, Schwartz SJ, Wicha MS, Sun D. Sulforaphane, a dietary component of broccoli/broccoli sprouts, inhibits breast cancer stem cells. Clin Cancer Res. 2010; 16:2580–2590. PMID: 20388854.


40. Lianga H, Yuana QP, Dong HR, Liu YM. Determination of sulforaphane in broccoli and cabbage by high-performance liquid chromatography. J Food Compos Anal. 2006; 19:473–476.
41. Baek SH, Park M, Suh JH, Choi HS. Protective effects of an extract of young radish (Raphanus sativus L) cultivated with sulfur (sulfur-radish extract) and of sulforaphane on carbon tetrachloride-induced hepatotoxicity. Biosci Biotechnol Biochem. 2008; 72:1176–1182. PMID: 18460814.


42. Ishiguro Y. Mucosal proinflammatory cytokine production correlates with endoscopic activity of ulcerative colitis. J Gastroenterol. 1999; 34:66–74. PMID: 10204613.


43. Kawasaki T, Fujimi S, Lederer JA, Hubbard WJ, Choudhry MA, Schwacha MG, Bland KI, Chaudry IH. Trauma-hemorrhage induces depressed splenic dendritic cell functions in mice. J Immunol. 2006; 177:4514–4520. PMID: 16982888.


44. Klotz L, Schmidt M, Giese T, Sastre M, Knolle P, Klockgether T, Heneka MT. Proinflammatory stimulation and pioglitazone treatment regulate peroxisome proliferator-activated receptor gamma levels in peripheral blood mononuclear cells from healthy controls and multiple sclerosis patients. J Immunol. 2005; 175:4948–4955. PMID: 16210596.
45. Calder PC. n-3 polyunsaturated fatty acids, inflammation, and inflammatory diseases. Am J Clin Nutr. 2006; 83(6 Suppl):1505S–1519S. PMID: 16841861.


46. Agirbasli M, Inanc N, Baykan OA, Direskeneli H. The effects of TNF alpha inhibition on plasma fibrinolytic balance in patients with chronic inflammatory rheumatical disorders. Clin Exp Rheumatol. 2006; 24:580–583. PMID: 17181930.
47. Bora NS, Sohn JH, Bora PS, Kaplan HJ, Kulkarni P. Anti-inflammatory effects of specific cyclooxygenase 2,5-lipoxygenase, and inducible nitric oxide synthase inhibitors on experimental autoimmune anterior uveitis (EAAU). Ocul Immunol Inflamm. 2005; 13:183–189. PMID: 16019677.


48. Dugo L, Marzocco S, Mazzon E, Di Paola R, Genovese T, Caputi AP, Cuzzocrea S. Effects of GW274150, a novel and selective inhibitor of iNOS activity, in acute lung inflammation. Br J Pharmacol. 2004; 141:979–987. PMID: 14769784.


49. Medeiros AI, Sá-Nunes A, Soares EG, Peres CM, Silva CL, Faccioli LH. Blockade of endogenous leukotrienes exacerbates pulmonary histoplasmosis. Infect Immun. 2004; 72:1637–1644. PMID: 14977971.


50. Skupień K, Oszmiański J, Kostrzewa-Nowak D, Tarasiuk J. In vitro antileukaemic activity of extracts from berry plant leaves against sensitive and multidrug resistant HL60 cells. Cancer Lett. 2006; 236:282–291. PMID: 16039042.


51. Sood S, Malhotra M, Das BK, Kapil A. Enterococcal infections & antimicrobial resistance. Indian J Med Res. 2008; 128:111–121. PMID: 19001673.
52. Fahey JW, Haristoy X, Dolan PM, Kensler TW, Scholtus I, Stephenson KK, Talalay P, Lozniewski A. Sulforaphane inhibits extracellular, intracellular, and antibiotic-resistant strains of Helicobacter pylori and prevents benzo[a]pyrene-induced stomach tumors. Proc Natl Acad Sci U S A. 2002; 99:7610–7615. PMID: 12032331.


53. Fahey JW, Stephenson KK, Wade KL, Talalay P. Urease from Helicobacter pylori is inactivated by sulforaphane and other isothiocyanates. Biochem Biophys Res Commun. 2013; 435:1–7. PMID: 23583386.


54. Johansson NL, Pavia CS, Chiao JW. Growth inhibition of a spectrum of bacterial and fungal pathogens by sulforaphane, an isothiocyanate product found in broccoli and other cruciferous vegetables. Planta Med. 2008; 74:747–750. PMID: 18484523.


Fig. 1
The cell cytotoxicity of RAW 264.7 cells treated with different concentrations of sulforaphane-containing solution (SCS). Cells were treated with 0, 1, 5, 10, 50, 100, or 500 µg/ml SCS in the presence of 0.1 µg/ml lipopolysaccharide (LPS) for 24 h. The normal group was treated with media only. Results are expressed as mean±standard error (SE) from 3 independent experiments. *p<0.01 indicates significant differences from the LPS treated cells.
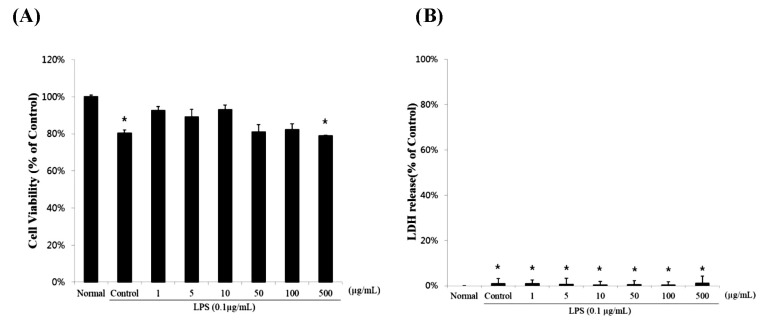
Fig. 2
Suppression of lipopolysaccharide (LPS)-induced nitric oxide (NO) and prostaglandin E2 (PGE2) production in RAW 264.7 cells using sulforaphane-containing solution (SCS). Cells were treated with 0, 1, 5, 10, 50, 100, or 500 µg/ml SCS in the presence of 0.1 µg/ml LPS for 18 h. The normal group was treated with media only. Results are expressed as mean±standard error (SE) from 3 independent experiments. *p<0.01 indicates significant differences from the LPS treated cells.
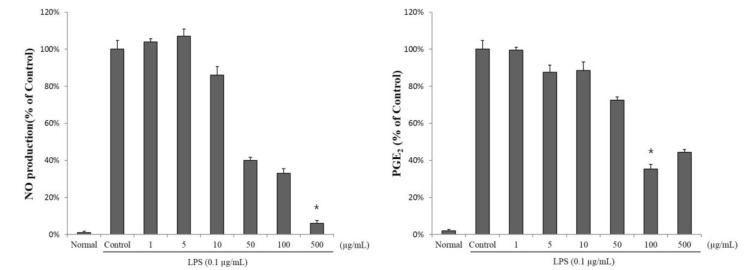
Fig. 3
Suppression of lipopolysaccharide (LPS)-induced cytokine production (interleukin 1β [IL-1β], IL-6, and IL-10 in RAW 264.7 cells using sulforaphane-containing solution (SCS). RAW 264.7 cells were treated with 0, 1, 5, 10, 50, 100, or 500 µg/ml SCS in the presence of 0.1 LPS for 18 h. The normal group was treated with media only. Results are expressed as mean±standard error (SE) from 3 independent experiments. *p<0.01 indicates significant differences from the LPS treated cells.
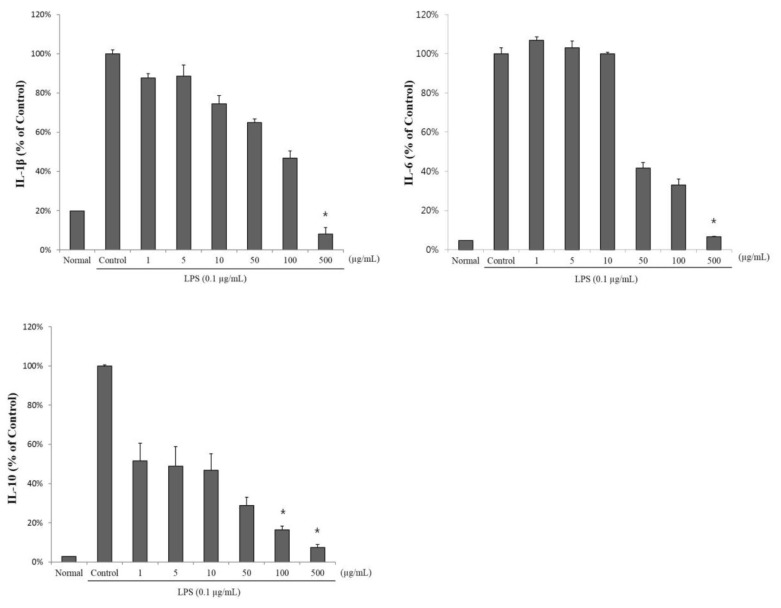
Fig. 4
Effects of sulforaphane-containing solution (SCS) on lipopolysaccharide (LPS)-induced inducible nitric oxide synthase (iNOS) and cyclooxygenase 2 (COX-2) protein expression. Inhibitory effect of SCS on LPS-induced iNOS and COX-2 protein expression in RAW 264.7 cells. Cells were treated with 0, 1, 5, 10, 50, 100, or 500 µg/ml SCS for 24 h. Cell lysates were electrophoretically separated and transferred, and expression levels of iNOS and COX-2 were detected with specific antibodies. β-actin was used as an internal control for western blot analysis.
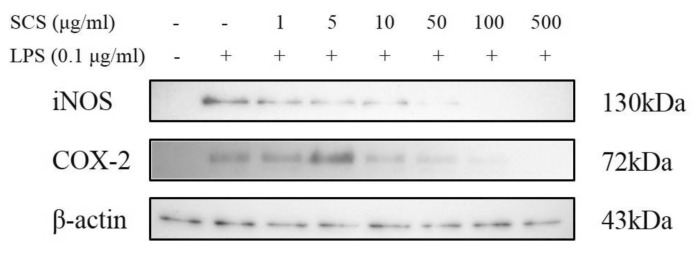
Fig. 5
Effects of sulforaphane-containing solution (SCS) on inducible nitric oxide synthase (iNOS) and cyclooxygenase 2 (COX-2) mRNA levels in lipopolysaccharide (LPS)-stimulated RAW 264.7 cells. Cells were treated with LPS or LPS with SCS at concentrations indicated in Fig. 5. After 24 h of incubation, total RNA was subjected to qRT-PCR. The RT products were labeled with SYBR Green dye. Relative iNOS and COX-2 mRNA expression (2-ΔΔCt) was determined by real-time PCR and calculated by Ct values for iNOS and COX-2 from β-actin mRNA. ΔΔCt=(Cttarget gene-Ctβ-actin)-(Ctcontrol-Ctβ-actin). Each value represents mean±SE of 3 independent experiments. *p<0.01 indicates significant differences from the LPS treated cells.
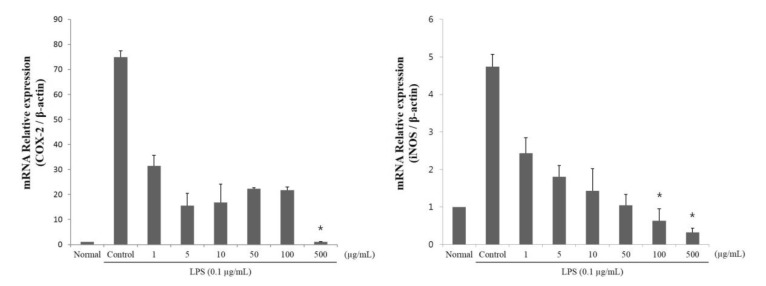