Abstract
Although various derivatives of caffeic acid have been reported to possess a wide variety of biological activities such as protection of neuronal cells against excitotoxicity, the biological activity of 1-docosanoyl cafferate (DC) has not been examined. The objective of the present study was to evaluate the anti-inflammatory effects of DC, isolated from the stem bark of Rhus verniciflua, on lipopolysaccharide (LPS)-stimulated BV2 microglial cells. Pretreatment of cells with DC significantly attenuated LPS-induced NO production, and mRNA and protein expression of iNOS in a concentration-dependent manner. DC also significantly suppressed LPS-induced release of cytokines such as TNF-α and IL-1β. Consistent with the decrease in cytokine release, DC dose-dependently and significantly attenuated LPS-induced mRNA expression of these cytokines. Furthermore, DC significantly suppressed LPS-induced degradation of IKB, which retains NF-kB in the cytoplasm. Therefore, nuclear translocation of NF-kB induced by LPS stimulation was significantly suppressed with DC pretreatment. Taken together, the present study suggests that DC exerts its anti-inflammatory activity through the suppression of NF-kB translocation to the nucleus.
References
1. Perry VH, Gordon S. Macrophages and microglia in the nervous system. Trends Neurosci. 1988; 11:273–277.


2. Hailer NP. Immunosuppression after traumatic or ischemic CNS damage: it is neuroprotective and illuminates the role of microglial cells. Prog Neurobiol. 2008; 84:211–233.


3. Matsumoto H. Some markers reflecting the pathology and disease activity of multiple sclerosis. No To Shinkei. 1992; 44:95–102.
4. McGeer PL, McGeer EG. Glial cell reactions in neurodegenerative diseases: pathophysiology and therapeutic interventions. Alzheimer Dis Assoc Disord. 1998; 12 Suppl. 2:S1–6.


5. Itagaki S, McGeer PL, Akiyama H, Zhu S, Selkoe D. Relationship of microglia and astrocytes to amyloid deposits of Alzheimer disease. J Neuroimmunol. 1989; 24:173–182.


6. Merrill JE, Chen IS. HIV-1, macrophages, glial cells, and cytokines in AIDS nervous system disease. FASEB J. 1991; 5:2391–2397.


7. Chao CC, Hu S, Close K, Choi CS, Molitor TW, Novick WJ, Peterson PK. Cytokine release from microglia: differential inhibition by pentoxifylline and dexamethasone. J Infect Dis. 1992; 166:847–853.


9. Merrill JE, Benveniste EN. Cytokines in inflammatory brain lesions: helpful and harmful. Trends Neurosci. 1996; 19:331–338.


10. Tuttolomondo A, Di Raimondo D, di Sciacca R, Pinto A, Licata G. Inflammatory cytokines in acute ischemic stroke. Curr Pharm Des. 2008; 14:3574–3589.


11. Kim MJ, Choi WC, Barshinikov AM, Koba-yashi A. Anticancer and antioxidant activity of allergen-removed extract in Rhus verniciflua stokes. Korean J Med Crop Sci. 2002; 10:288–293.
12. Kim ML, Choi YH, Kim WG, Kwak SS. Antioxidative activity of urushiol derivatives from the sap oflacquer tree (Rhus verniciflua Stokes). Korean J Plant Resour. 1997; 10:227–230.
13. Kim MJ, Kim CJ, Kwak SS. Antifungal activity of urushiol component in the sap of Korean lacquer tree (Rhus vernicifera Stokes). Korean J Plant Resour. 1997; 10:231–234.
14. Park KY, Jung GO, Lee KT, Choi J, Choi MY, Kim GT, Jung HJ, Park HJ. Antimutagenic activity of flavonoids from the heartwood of Rhus verniciflua. J Ethnopharmacol. 2004; 90:73–79.


15. Jeong JS, Park JW, Yoon SW. Carcinostatic effect of allergen removed Rhus verniciflua Stokes based traditional Korean medicine on a patient with lung adenocarcinoma; single case report. Orient Pharm Exp Med. 2008; 7:573–578.


16. Jeon WK, Lee JH, Kim HK, Lee AY, Lee SO, Kim YS, Ryu SY, Kim SY, Lee YJ, Ko BS. Anti-platelet effects of bioactive compounds isolated from the bark of Rhus verniciflua Stokes. J Ethnopharmacol. 2006; 106:62–69.


17. Byun JS, Han YH, Hong SJ, Hwang SM, Kwon YS, Lee HJ, Kim SS, Kim MJ, Chun W. Bark constituents from mushroom-detoxified Rhus verniciflua suppress kainic acid-induced neuronal cell death in mouse hippocampus. Korean J Physiol Pharmacol. 2010; 14:279–283.
18. Kim SR, Kim YC. Neuroprotective phenylpropanoid esters of rhamnose isolated from roots of Scrophularia buergeriana. Phytochemistry. 2000; 54:503–509.


19. Sul D, Kim HS, Lee D, Joo SS, Hwang KW, Park SY. Protective effect of caffeic acid against beta-amyloid-induced neurotoxicity by the inhibition of calcium influx and tau phosphorylation. Life Sci. 2009; 84:257–262.


21. Wei X, Ma Z, Fontanilla CV, Zhao L, Xu ZC, Taggliabraci V, Johnstone BH, Dodel RC, Farlow MR, Du Y. Caffeic acid phenethyl ester prevents cerebellar granule neurons (CGNs) against glutamate-induced neurotoxicity. Neuroscience. 2008; 155:1098–1105.


22. Tsai SK, Lin MJ, Liao PH, Yang CY, Lin SM, Liu SM, Lin RH, Chih CL, Huang SS. Caffeic acid phenethyl ester ameliorates cerebral infarction in rats subjected to focal cerebral ischemia. Life Sci. 2006; 78:2758–2762.


23. Komatsu M, Tomimori T, Hatayama K, Makiguchi Y. Studies on the constituents of Sophora species. 3. Constituents of the root of Sophora subprostrata Chun et T. Chen. Yakugaku Zasshi. 1970; 90:459–462.
24. Ghosh S, May MJ, Kopp EB. NF-kappa B and Rel proteins: evolutionarily conserved mediators of immune responses. Annu Rev Immunol. 1998; 16:225–260.
25. Bonizzi G, Karin M. The two NF-kappaB activation pathways and their role in innate and adaptive immunity. Trends Immunol. 2004; 25:280–288.
26. Lapchak PA. The phenylpropanoid micronutrient chlorogenic acid improves clinical rating scores in rabbits following multiple infarct ischemic strokes: synergism with tissue plasminogen activator. Exp Neurol. 2007; 205:407–413.


27. Li YY, Lu JH, Li Q, Zhao YY, Pu XP. Pedicularioside A from Buddleia lindleyana inhibits cell death induced by 1-methyl-4-phenylpyridinium ions (MPP+) in primary cultures of rat mesencephalic neurons. Eur J Pharmacol. 2008; 579:134–140.


28. Jiang WL, Tian JW, Fu FH, Zhu HB, Hou J. Neuroprotective efficacy and therapeutic window of Forsythoside B: in a rat model of cerebral ischemia and reperfusion injury. Eur J Pharmacol. 2010; 640:75–81.


29. Deng M, Zhao JY, Tu PF, Jiang Y, Li ZB, Wang YH. Echinacoside rescues the SHSY5Y neuronal cells from TNFalpha-induced apoptosis. Eur J Pharmacol. 2004; 505:11–18.
30. Kang SS, Kim CM. Studies on the Korean Indigenous Plants. Isolation of 1-eicosanoyl cafferate from Echinosophora koreensis. Arch Pharm Res. 1987; 10:67–68.
31. Kreutzberg GW. Microglia: a sensor for pathological events in the CNS. Trends Neurosci. 1996; 19:312–318.


32. Aquilano K, Baldelli S, Rotilio G, Ciriolo MR. Role of nitric oxide synthases in Parkinson's disease: a review on the antioxidant and anti-inflammatory activity of polyphenols. Neurochem Res. 2008; 33:2416–2426.


33. Hashioka S, McGeer PL, Monji A, Kanba S. Anti-inflammatory effects of antidepressants: possibilities for preventives against Alzheimer's disease. Cent Nerv Syst Agents Med Chem. 2009; 9:12–19.


34. Ray B, Lahiri DK. Neuroinflammation in Alzheimer's disease: different molecular targets and potential therapeutic agents including curcumin. Curr Opin Pharmacol. 2009; 9:434–444.


35. Li Q, Verma IM. NF-kappaB regulation in the immune system. Nat Rev Immunol. 2002; 2:725–734.
36. Karin M, Takahashi T, Kapahi P, Delhase M, Chen Y, Makris C, Rothwarf D, Baud V, Natoli G, Guido F, Li N. Oxidative stress and gene expression: the AP-1 and NF-kappaB connections. Biofactors. 2001; 15:87–89.
37. Cho IH, Hong J, Suh EC, Kim JH, Lee H, Lee JE, Lee S, Kim CH, Kim DW, Jo EK, Lee KE, Karin M, Lee SJ. Role of microglial IKKbeta in kainic acid-induced hippocampal neuronal cell death. Brain. 2008; 131:3019–3033.
38. Romano MF, Avellino R, Petrella A, Bisogni R, Romano S, Venuta S. Rapamycin inhibits doxorubicin-induced NF-kappaB/Rel nuclear activity and enhances the apoptosis of melanoma cells. Eur J Cancer. 2004; 40:2829–2836.
39. Barger SW, Hörster D, Furukawa K, Goodman Y, Krieglstein J, Mattson MP. Tumor necrosis factors alpha and beta protect neurons against amyloid beta-peptide toxicity: evidence for involvement of a kappa B-binding factor and attenuation of peroxide and Ca2+ accumulation. Proc Natl Acad Sci U S A. 1995; 92:9328–9332.
40. Goodman Y, Mattson MP. Ceramide protects hippocampal neurons against excitotoxic and oxidative insults, and amyloid beta-peptide toxicity. J Neurochem. 1996; 66:869–872.
41. Mattson MP, Goodman Y, Luo H, Fu W, Furukawa K. Activation of NF-kappaB protects hippocampal neurons against oxidative stress-induced apoptosis: evidence for induction of manganese superoxide dismutase and suppression of peroxynitrite production and protein tyrosine nitration. J Neurosci Res. 1997; 49:681–697.
Fig. 2.
Effects of DC on NO production and iNOS expression in LPS-stimulated BV2 microglial cells. (A) Effect of DC on the viability of BV2 microglial cells. No noticeable cell death was observed up to 20 μM. (B) Concentration-dependent suppression of LPS-induced NO production by DC and CA (caffeic acid). (C) Inhibitory effect of DC on LPS-induced upregulation of iNOS mRNA expression. (D) Suppression of LPS-induced iNOS protein expression by DC: top, quantitative analysis of immunoblots; bottom, representative immunoblot of iNOS. β-Actin was used as an internal control. Quantitative data represent three independent experiments and are expressed as mean±SD. ∗p<0.05, ∗∗p<0.01, and ∗∗∗p< 0.001 indicate statistically significant differences compared to LPS alone. #p<0.05 indicate statistically significant differences between the indicated groups.
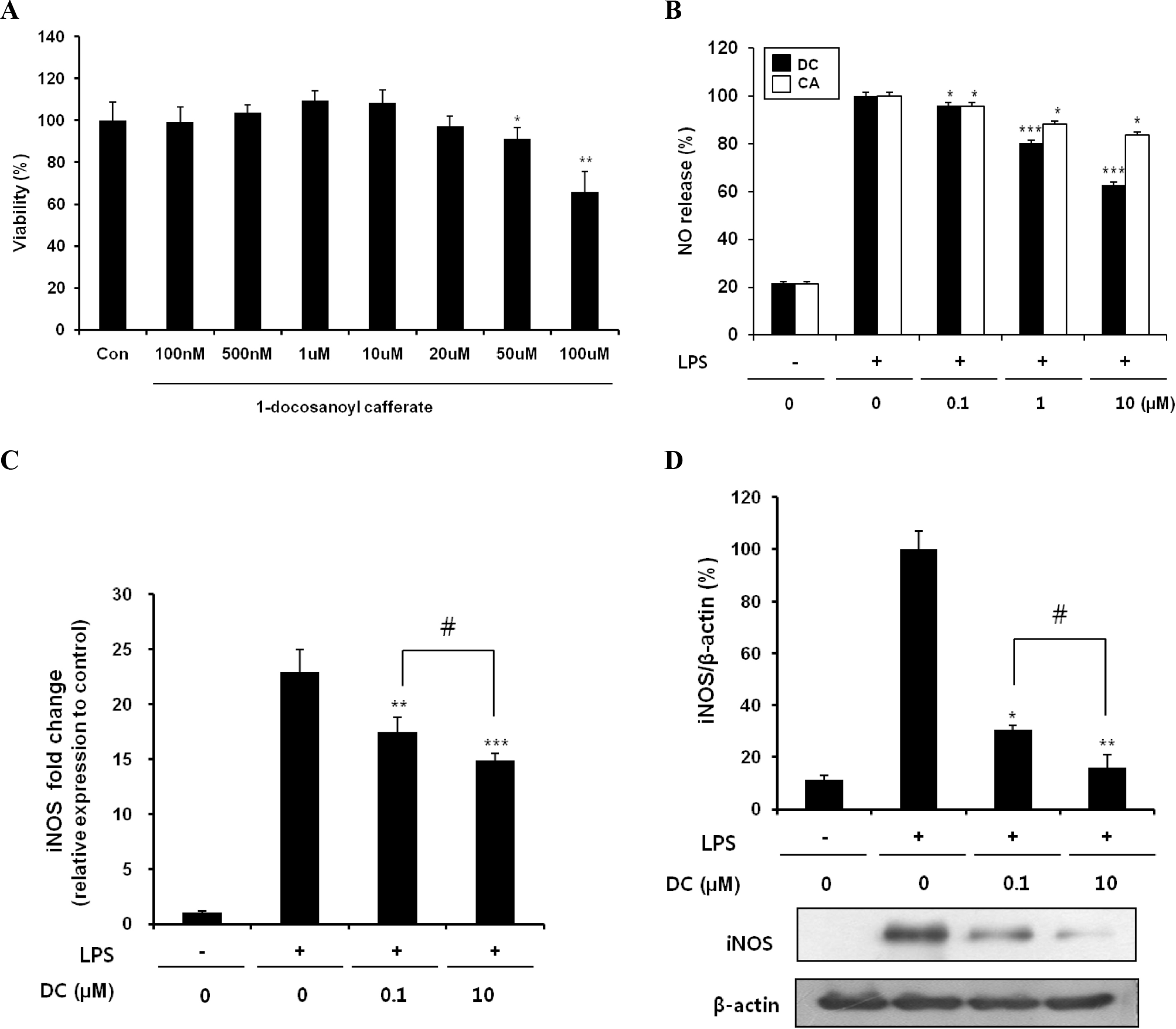
Fig. 3.
Inhibitory effects of DC on LPS-induced release of TNF-α (A) and IL-1β (B) in BV2 microglial cells. BV2 microglia cells were incubated with 200 ng/ml of LPS in the presence or absence of the indicated concentrations of DC for 24 hr. Cell culture media were collected and subjected to TNF-α and IL-1β sandwich ELISAs. Data represent three independent experiments, each run in triplicate, and are expressed as mean±SD. ∗p<0.05 and ∗∗p<0.01 indicate statistically significant differences compared to LPS alone. ##p<0.01 indicates a statistically significant difference between the indicated groups.
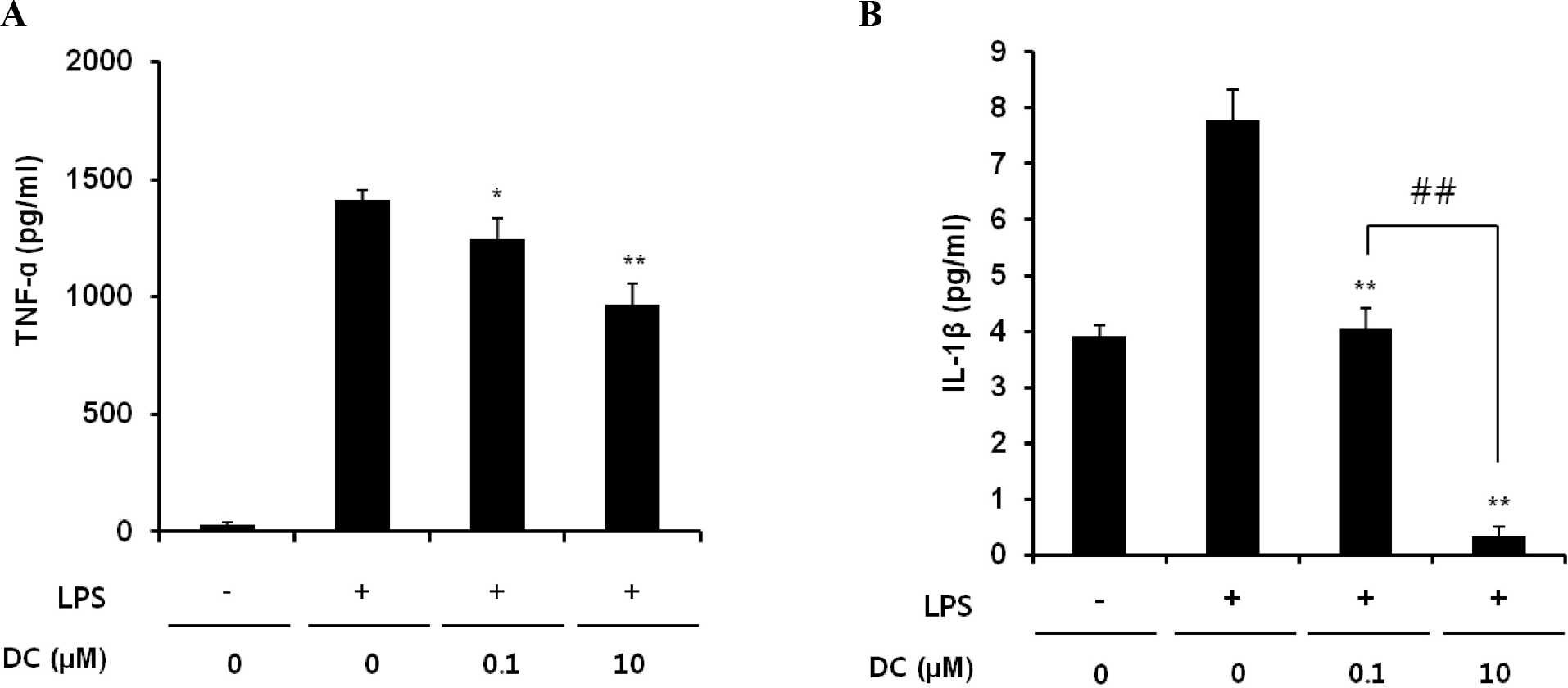
Fig. 4.
Effects of DC on the gene expression of TNF-α (A) and IL-1β (B) in LPS-stimulated BV2 microglial cells. Cells were incubated with DC for 1 hr prior to exposure to 200 ng/ml LPS. Total RNA was isolated 6 hr after LPS treatment. TNF-α and IL-1β mRNA levels were determined by real time PCR. Data represent three independent experiments, each done in triplicate, and are expressed as mean±SD. ∗p<0.05 indicates a statistically significant difference compared to LPS alone.
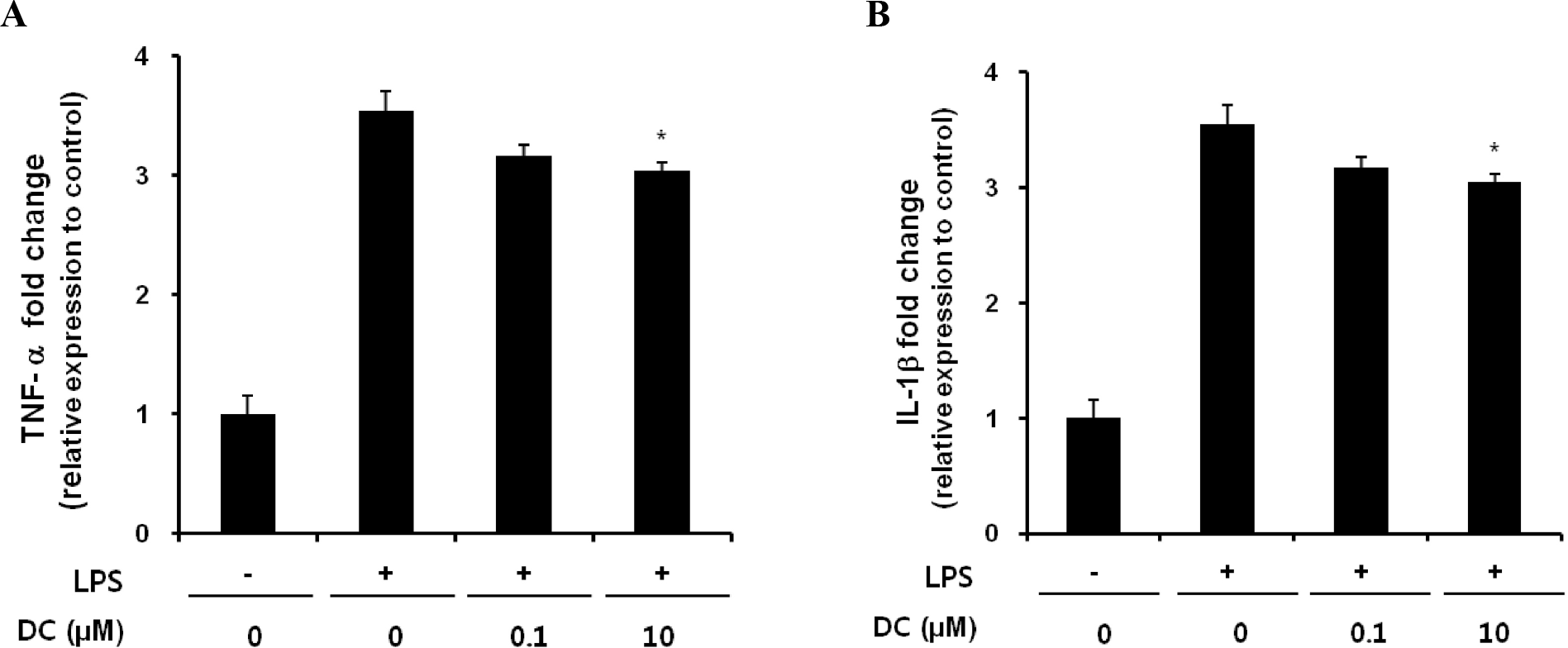
Fig. 5.
Inhibitory effects of DC on LPS-induced IkB-α degradation in BV2 microglial cells. The intracellular level of IKB-α was determined using immunoblotting analysis: top, quantitative analysis of immunoblots; bottom, representative immunoblot of IKB-α. β-Actin was used as an internal control. Quantitative data represent three independent experiments and are expressed as mean±SD. ∗p <0.05 and ∗∗p<0.01 indicate statistically significant differences compared to LPS alone. ##p<0.01 indicates a statistically significant difference between the indicated groups.
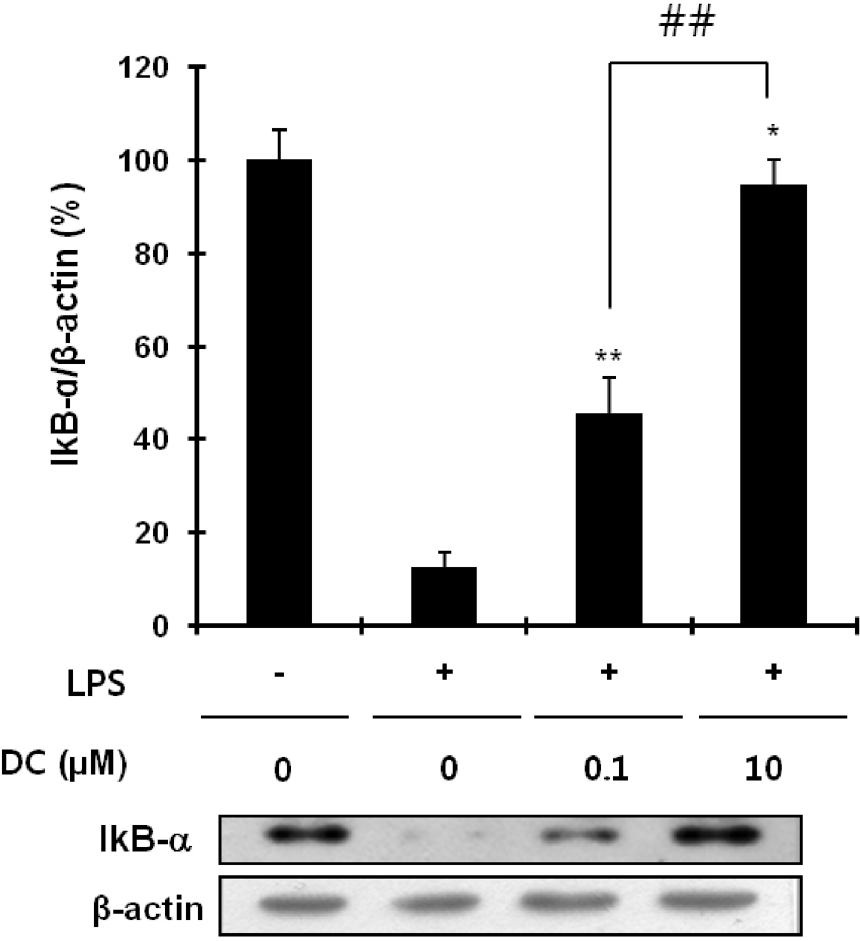
Fig. 6.
Blockade by DC of nuclear translocation of the p65 subunit of NF-kB in LPS-stimulated BV2 microglial cells. (A) Localization of the NF-kB p65 subunit was determined using a p65 antibody and an Alexa 546-labeled goat anti-rabbit IgG antibody. Nuclei were visualized by Hoechst staining (Hoechst 33258). In basal conditions, immunostaining of p65 subunit was diffuse throughout the cytoplasm. LPS stimulation resulted in the translocation of p65 subunits into the nucleus. Pretreatment with DC attenuates LPS-induced nuclear translocation of the p65 subunit. (B) Line scanning analysis of confocal images further visualizes the intracellular localization of the p65. Scale bar, 20μm.
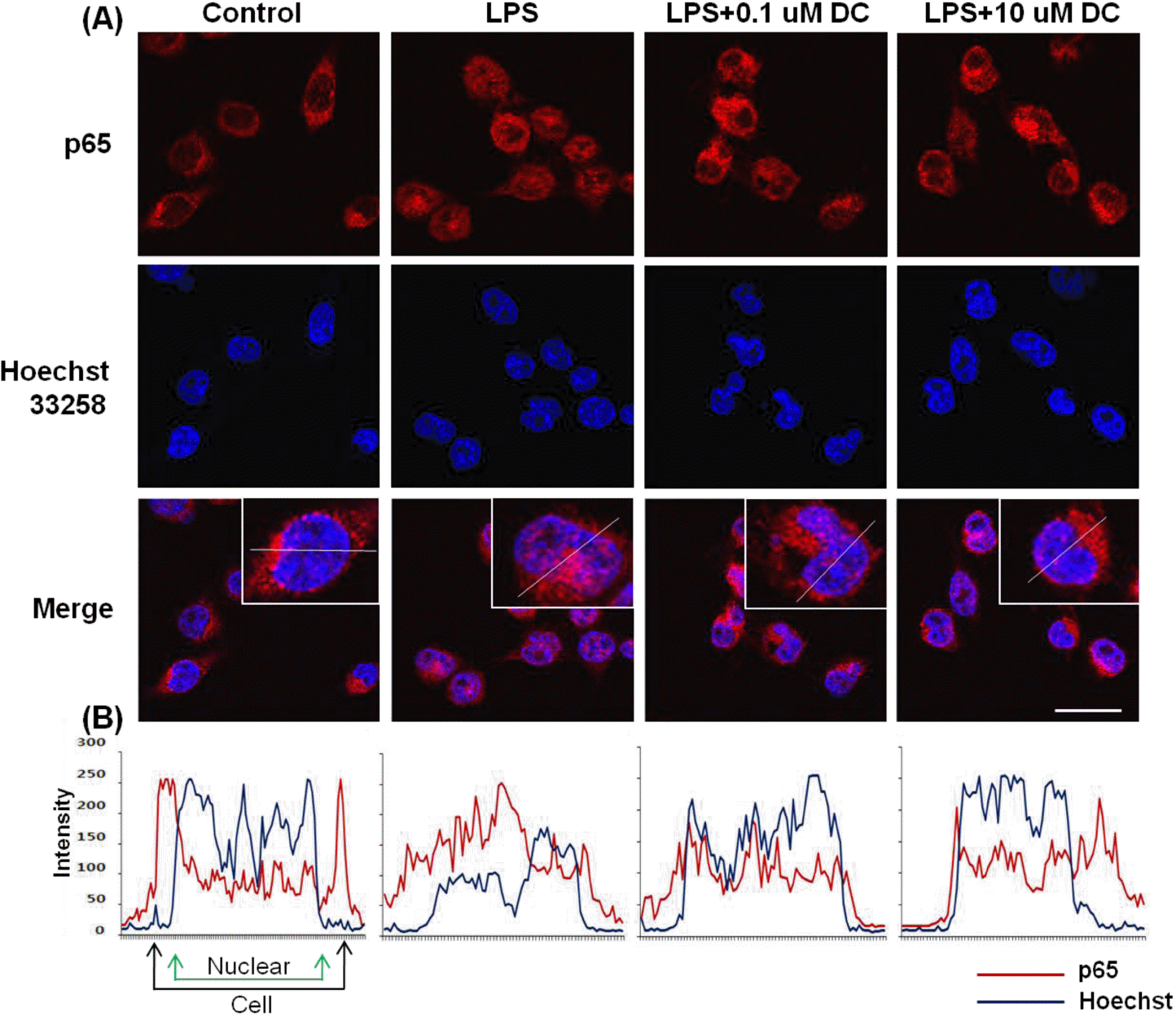