Abstract
Purpose
Nasal polyps are associated with chronic inflammation of the mucous membranes in the nose and paranasal sinuses and involved in extracellular matrix (ECM) accumulation. Delphinidin promotes ECM degradation in hepatitis and cardiac fibrosis. The aims of this study were to examine the inhibitory effect of delphinidin on TGF-β1-induced myofibroblast differentiation and ECM accumulation, and to determine the underlying mechanisms in nasal polyp-derived fibroblasts (NPDFs).
Methods
NPDFs were stimulated with TGF-β1, with or without delphinidin, and the expression levels of α-SMA, fibronectin, and collagen type I were determined by RT-PCR, Western blot analysis, and collagen assay. The expression of α-SMA protein was measured by immunocytochemical staining. Mitogen-activated protein kinase and NF-κB activation induced by TGF-β1 were determined by Western blot analysis. The transcriptional activity of NF-κB was measured by luciferase assay.
Results
The expression levels of α-SMA, fibronectin, and collagen type I increased in TGF-β1-stimulated NPDFs. In TGF-β1-induced NPDFs, delphinidin inhibited the expression of α-SMA, fibronectin, and collagen. Inhibitors of MAPK and NF-κB blocked the expression of α-SMA, fibronectin, and collagen type I. Delphinidin suppressed the activation of MAPK and NF-κB induced by TGF-β1 stimulation.
Nasal polyps are a chronic inflammatory disorder characterized by tissue remodeling consisting of epithelial proliferation, goblet cell hyperplasia, pseudocyst formation, basement membrane thickening, focal fibrosis, and edema in the nose and paranasal sinuses.1,2 In nasal polyps, the highest density of myofibroblasts is found in the pedicle area.3 Fibroblasts, which are the cellular source of extracellular matrix (ECM) protein, could therefore be involved in the growth of nasal polyps. Myofibroblasts represent an activated cell phenotype with contractile tissue properties and a high capacity for ECM protein secretion.4 However, the etiology and pathogenesis of nasal polyps have not been elucidated.
Myofibroblasts that express alpha-smooth muscle actin (α-SMA) comprise an activated cell phenotype of fibroblasts with a high capacity for ECM protein secretion and play an important role in ECM remodeling of many pathologic conditions of the human airway, including asthma, chronic rhinosinusitis, and nasal polyps.5 TGF-β1 induces myofibroblast differentiation and the accumulation of ECM, including collagen and fibronectin, in nasal polyps.6
Three isoforms of transforming growth factor-beta (TGF-β) are TGF-β1, TGF-β2, and TGF-β3. TGF-β1 is believed to be the main isoform in nasal polyps and produced by a variety of cells, including lymphocytes, macrophages, eosinophils, and fibroblasts.7,8 Stimulation with low levels of TGF-β1 enhances fibroblast proliferation and increases the release of profibrotic factors, while higher TGF-β1 levels enhance differentiation to myofibroblasts.6,7 TGF-β1 enhances the changes in fibroblast phenotype and the synthesis of ECM proteins.9
Delphinidin (2-(3,4,5-trihydroxyphenyl) chromenylium-3,5,7-triol) is an active ingredient of pomegranate and other pigmented fruits with diverse pharmacological properties, which have been shown to be rich sources of phytochemicals.10 Delphinidin is the major anthocyanidin present in pigmented fruits, such as pomegranate, berries and dark grapes, and vegetables, such as eggplant, tomato, carrot, purple sweet potato, red cabbage and red onion, and it possesses strong antioxidant and anti-inflammatory properties.10,11 Delphinidin inhibits liver and cardiac fibrosis by promoting ECM degradation12; thus, delphinidin may be a novel therapeutic agent for nasal polyps.
In this study, we investigated whether delphinidin inhibits TGF-β1-induced myofibroblast differentiation and ECM accumulation in nasal polyp-derived fibroblasts (NPDFs).
Human recombinant TGF-β1 was obtained from R&D Systems (Minneapolis, MN, USA). Delphinidin was provided by Sigma (St. Louis, MO, USA). Inhibitors of ERK kinase (U0126), p38 (SB203580), and JNK (SP600125) were purchased from Calbiochem (Billerica, MA, USA) and were dissolved in dimethyl sulfoxide. NF-κB inhibitor (BAY-11) was provided by Sigma and dissolved in dimethyl sulfoxide. Antibodies against phospho-ERK, phospho-p38, phospho-JNK, p50, fibronectin, α-SMA, and β-actin were obtained from Santa Cruz Biotechnology (Santa Cruz, CA, USA).
Eight patients with nasal polyps were recruited from the Department of Otorhinolaryngology, Korea University Medical Center, Korea. Informed consent was obtained from each patient, and the study was approved by the Korea University Medical Center Institutional Review Board (KUGGR-2010-050). NPDFs were isolated from surgical tissues by enzymatic digestion with collagenase (500 U/mL, Sigma), hyaluronidase (30 U/mL, Sigma), and DNase (10 U/mL, Sigma). Cells were cultured in Dulbecco's Modified Eagle Medium (DMEM) containing 10% (v/v) heat-inactivated fetal bovine serum (Invitrogen, Carlsbad, CA, USA), 1,000 unit/mL penicillin, and 1,000 µg/mL streptomycin (Invitrogen). The purity of the obtained NPDFs was confirmed by characteristic spindle-shaped cell morphology and flow cytometry. Experimental cells were obtained from the fourth cell passage.
NPDFs were stimulated with TGF-β1 (5 ng/mL), with or without delphinidin (0-20 µM), for 24 hours. Total RNA was isolated with Trizol reagent (Invitrogen). Two micrograms of RNA was reverse-transcribed with MMLV reverse transcriptase (Invitrogen). PCR was performed using the following primers: α-smooth muscle actin (sense sequence 5'-GGT GCT GTC TCT CTA GCC TCT GGA-3' and anti-sense sequence 5'-CCC ATC AGG CAA CTC GAT ACT CTT C-3', 322 bp); collagen type I (sense sequence 5'-CAT CAC CTA CCA CTG CAA GAA C-3' and anti-sense sequence 5'-ACG TCG AAG CCG AAT TCC-3', 278 bp); fibronectin (sense sequence 5'-GGA TGC TCC TGC TGT CAC-3' and anti-sense sequence 5'-CTG TTT GAT CTG GAC CTG CAG-3', 386 bp); GAPDH (sense sequence 5'-GTG GAT ATT GTT GCC ATC AAT GAC C-3' and anti-sense sequence 5'-GCC CCA GCC TTC TTC ATG GTG GT-3', 271 bp). Amplification reactions were performed as follows: an initial 5 minutes denaturation step at 94℃; 30 cycles of 94℃ for 45 seconds, 55℃-65℃ for 45 seconds, 72℃ for 45 seconds; and an extension step at 74℃ for 5 minutes. All reactions were performed in a 20-µL volume. Products were electrophoresed on a 1.5% agarose gel and visualized by staining with ethidium bromide. The gels were captured and visualized with Molecular Imager ChemiDoc XRS+ (Bio-Rad, Hercules, CA, USA).
NPDFs were plated on coverslips and exposed to TGF-β1 (5 ng/mL) with or without delphinidin (20 µM) for 24 hours. NPDFs were fixed with 4% paraformaldehyde. NPDFs were permeabilized with 0.2% Triton X-100 in 1% bovine serum albumin for 10 minutes, blocked with 5% bovine serum albumin for 1 hour at room temperature and incubated overnight at 4℃ with monoclonal anti-α-SMA. NPDFs were then incubated with anti-mouse Alexa 488 secondary antibodies (Invitrogen). Finally, coverslips were counterstained with 4'-6-diamidino-2-phenylindole. Stained NPDFs were captured and visualized using a confocal laser scanning microscope (LSM700, Zeiss, Oberkochen, Germany).
NPDFs were stimulated with TGF-β1 (5 ng/mL), with or without delphinidin (0-20 µM), for 72 hours. The amount of total soluble collagen in cell culture supernatants was quantified using the Sircol collagen assay (Biocolor, Belfast, UK). One milliliter of Sirius red dye, an anionic dye that reacts specifically with basic side chain groups of collagens under assay conditions, was added to 400 mL of the supernatant and incubated with gentle rotation for 30 minutes at room temperature. The samples were then centrifuged at 12,000 rpm for 10 minutes, and the collagen-dye complex precipitate was collected and resolubilized in 0.5 M sodium hydroxide. The dye concentration was estimated by spectrophotometry at 540 nm (Beckman Coulter, Fullerton, CA, USA). The absorbance was directly proportional to the amount of newly formed soluble collagen in the cell culture supernatant.
NPDFs were stimulated with TGF-β1 (5 ng/mL), with or without delphinidin (0-20 µM), for 48 hours. NPDFs were lysed in PRO-PREPTM protein extraction solution (iNtRON Biotechnology, Seongnam, Korea). Lysates were separated by 10% SDS-PAGE and transferred onto polyvinyl difluoride (PVDF) membranes (Millipore Inc., Billerica, MA, USA). Membranes were blocked with a 5% skim milk solution and incubated with the following antibodies: α-SMA, fibronectin, phospho-ERK, phospho-p38, phospho-JNK, p50, and β-actin. The blots were visualized with HRP-conjugated secondary antibodies and an ECL system (Pierce, Rockford, IL, USA).
NPDFs were plated on 60-mm cell culture plates. NF-κB luciferase reporter gene constructs (luc2P/NF-κB-RE/Hygro and hRluc/TK; Promega, Madison, WI, USA) were transiently transfected into NPDFs by using fetal bovine serum and antibiotic-free DMEM containing 5 µL of FuGENE transfection reagent (Promega). After 5 hours of incubation, the medium was replaced with DMEM containing 10% fetal bovine serum. After incubation for 24 hours, transfected NPDFs were treated with TGF-β1 (5 ng/mL), with or without delphinidin (20 µM), MAPK inhibitors (10 µM), and NF-κB inhibitor (1 µM) at 37℃ for 2 hours. Luciferase assays were performed to determine the firefly luciferase activity relative to the Renilla luciferase activity in the cell lysate using a luminometer (Promega).
For all results, data were obtained from at least 3 independent experiments. The statistical significance of differences between control and experimental data was analyzed by unpaired t tests or one-way analysis of variance, followed by Tukey's test (GraphPad Prism, version 5, Graph Pad Software, San Diego, CA, USA). Significance was established at the 95% confidence level. A P value of less than 0.05 was considered statistically significant.
To investigate the inhibitory effect of delphinidin on myofibroblast differentiation, NPDFs were treated with TGF-β1, with or without delphinidin, at different concentrations (0-20 µM). Delphinidin significantly inhibited the TGF-β1-induced mRNA expression of α-SMA, a marker for myofibroblasts (P<0.05, Fig. 1A). The inhibitory effect of delphinidin on TGF-β1-induced α-SMA protein production was confirmed by western blot analysis and immunofluorescent staining (Fig. 1B and C). The mRNA and protein expression levels of α-SMA were inhibited by delphinidin treatment in a dose-dependent manner.
Collagen and fibronectin are ECM components. Therefore, to examine the inhibitory effect of delphinidin on ECM accumulation, NPDFs were treated with TGF-β1, with or without delphinidin at different concentrations, and collagen and fibronectin levels were assessed. Delphinidin significantly inhibited TGF-β1-induced collagen type I and fibronectin mRNA expression (P<0.05, Fig. 2A). Furthermore, TGF-β1-induced fibronectin and collagen protein expression was suppressed by delphinidin treatment (Fig. 2B and C).
To verify whether the MAPK signaling pathway that includes ERK, p38, and JNK is involved with the expressions of α-SMA, fibronectin, and collagen, NPDFs were treated with TGF-β1, with or without delphinidin or MAPK inhibitors. We examined the activation of MAPK signal proteins by Western blot analysis and determined that TGF-β1 activated all 3 MAPKs, including ERK, p38 and JNK. Delphinidin inhibited the activation of the 3 MAPKs (P<0.05, Fig. 3A). In addition, the expressions of TGF-β1-induced α-SMA, fibronectin, and collagen were significantly inhibited by all 3 MAPK inhibitors (Fig. 3B and C).
To determine the role of NF-κB signaling pathways in the expressions of α-SMA, fibronectin, and collagen, NPDFs were treated with TGF-β1, with or without delphinidin, MAPK inhibitors, or NF-κB inhibitor (BAY-11). The protein expression level of p50, an NF-κB subunit, was inhibited by delphinidin and the NF-κB inhibitor (Fig. 4A). In addition, the NF-κB inhibitor suppressed TGF-β1-induced α-SMA, fibronectin and collagen production (Fig. 4B and C). The transcriptional activity of NF-κB was significantly inhibited by delphinidin, the NF-κB inhibitor, and MAPK inhibitors (Fig. 4D).
In this study, we showed that delphinidin suppressed myofibroblast differentiation and ECM accumulation in TGF-β1-stimulated NPDFs. Additionally, we determined that delphinidin inhibited the MAPK (ERK1/2, JNK, p38) and NF-κB pathways in TGF-β1-stimulated NPDFs.
Nasal polyps are part of chronic inflammatory disease that involves benign outgrowths from the mucous membranes of the nasal cavity or paranasal sinuses. One of the development processes of nasal polyps is myofibroblast differentiation from fibroblasts and ECM production.13 It has also been demonstrated that TGF-β1 induces myofibroblast differentiation in nasal polyps.14 Although there have been efforts to identify the etiologies of nasal polyps and pathophysiological mechanisms for nasal polyp formation, the mechanisms of nasal polyp development are still unclear.
Antioxidants seem to be effective in the treatment of chronic liver damage and fibrosis.15 Polyphenols, naturally occurring antioxidants in fruits, vegetables, and plant-derived beverages such as tea and wine, have been associated with a variety of beneficial properties.16 Among polyphenols, anthocyanins abundantly present in pigmented fruits and vegetables have attracted the attention of scientists over the last decade, as they appear to possess potent activity against various pathological conditions. The anthocyanidin delphinidin has been shown to possesses antioxidant, anti-inflammatory, antimutagenic, and antiangiogenic properties.17 In a previous report, delphinidin was shown to inhibit liver and cardiac fibrosis by promoting ECM degradation.12 Thus, delphinidin may be a useful therapeutic agent for nasal polyps.
Myofibroblasts are an activated phenotype of fibroblasts and produce large amounts of ECM components, such as fibronectin and collagen. Myofibroblasts are induced by TGF-β1, which induces ECM deposition.18 Previous studies suggest that the differentiation of fibroblasts into myofibroblasts and ECM accumulation may be important factors in the pathogenesis of nasal polyp formation.19 Myofibroblasts, which express α-SMA, can be transdifferentiated from fibroblasts in vitro by exposure to the fibrogenic cytokine, TGF-β1.20 TGF-β1 is thought to be an important cytokine for myofibroblast differentiation and the induction of pulmonary fibrosis in vivo,21 and has been shown to cause ECM deposition.22 We showed that TGF-β1 significantly induced the expression of α-SMA as well as the production of fibronectin and collagen in NPDFs. Delphinidin inhibited the expression of α-SMA, fibronectin, and collagen in a dose-dependent manner. These results suggest that delphinidin suppresses TGF-β1-induced myofibroblast differentiation and ECM accumulation in NPDFs.
TGF-β signaling is initiated by binding to 2 cell membrane receptors (types I and II). After binding of TGF-β1 to the receptor, Smad proteins, which are primary substrates and regulate the canonical TGF-β1 signaling pathway, are phosphorylated and activate transcription for TGF-β1 signal activation.23 The MAPK pathways have been reported as Smad-independent TGF-β1 signaling pathways.24 The MAPK pathways consist of many phosphorylation cascades, each of which modulates different signaling events, either alone or in combination. ERK, JNK, and p38 are known to participate in TGF-β signaling cascades.25 Through Western blot analysis, we determined that TGF-β1 activated the MAPK signaling pathways, including ERK1/2, p38, and JNK phosphorylation. However, delphinidin significantly inhibited all 3 MAPK pathways and the MAPK inhibitors suppressed the protein expressions of α-SMA, fibronectin, and collagen. These results indicate that delphinidin inhibits TGF-β1-induced myofibroblast differentiation and ECM accumulation via the MAPK signaling pathways in NPDFs.
MAPK activation regulates phosphorylation of the downstream transcription factor NF-κB, which leads to activation of the NF-κB signaling pathway. TGF-β1 induces the activation of MAPK signaling, and the activated MAPK promotes nuclear translocation and DNA binding of NF-κB.26 NF-κB consists of subunits, including p65 and p50. We demonstrated that TGF-β1 induced the expression of NF-κB and that a specific inhibitor of NF-κB suppressed the protein expressions of α-SMA, fibronectin, and collagen in TGF-β1-induced NPDFs. Moreover, the transcriptional activity of NF-κB increased in response to TGF-β1 treatment. However, this activity decreased upon treatment with NF-κB and MAPK inhibitors in TGF-β1-induced NPDFs. These results suggest that TGF-β1 may activate MAPK signaling and that NF-κB this the downstream effector of MAPK signaling in TGF-β1-induced NPDFs. The results of the present study provide evidence that delphinidin inhibits TGF-β1-induced myofibroblast differentiation and ECM accumulation in NPDFs through the MAPK/ NF-κB pathway (Fig. 5).
In conclusion, we showed that delphinidin inhibits TGF-β1-induced myofibroblast differentiation and ECM accumulation through the MAPK signaling pathway and NF-κB activation in NPDFs. Additional clinical and basic studies are needed to investigate the feasibility of delphinidin as a therapeutic agent for nasal polyps.
Figures and Tables
Fig. 1
Effect of delphinidin on myofibroblast differentiation (α-SMA) induced by TGF-β1 in NPDFs. NPDFs were treated with TGF-β1 (5 ng/mL), with or without delphinidin (Del, 0-20 µM). The mRNA expression level of α-SMA was determined by RT-PCR (A). Protein production of α-SMA was measured by Western blot (B) and visualized by immunofluorescence staining with an antibody to α-SMA (C). Values are the means ±SEM of the 3 independent experiments. Images were acquired by confocal laser scanning microscopy. *P<0.05 vs control; †P<0.05 vs TGF-β1. Scale bar=50 µm.
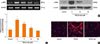
Fig. 2
Effect of delphinidin on extracellular matrix production induced by TGF-β1 in NPDFs. NPDFs were treated with TGF-β1 (5 ng/mL), with or without delphinidin (Del, 0-20 µM). The mRNA expression levels of collagen type I and fibronectin were determined by RT-PCR (A). The protein expression level of fibronectin was measured by Western blot (B), and the total amount of collagen was measured by Sircol assay (C). Values are the means ±SEM of the 3 independent experiments. *P<0.05 vs control; †P<0.05 vs TGF-β1.
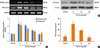
Fig. 3
Effect of delphinidin on TGFβ1-induced MAPK signaling pathways in NPDFs. NPDFs were stimulated with TGF-β1 (5 ng/mL), with or without delphinidin (20 µM) and MAPK inhibitors, including those for ERK (U0126, 10 µM), p38 (SB203580, 10 µM), and JNK (SP600125, 10 µM). (A) The phosphorylation of MAPK signaling molecules, including p-ERK, p-JNK, and p-p38, was measured by Western blot. (B) The protein expression levels of α-SMA and fibronectin were determined by Western blot. (C) The total amount of collagen was measured by collagen assay. Values are the means ±SEM of the 3 independent experiments. *P<0.05 vs control; †P<0.05 vs TGF-β1.
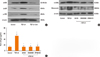
Fig. 4
Effect of delphinidin on TGFβ1-induced NF-κB signaling in NPDFs. NPDFs were stimulated with TGF-β1 (5 ng/mL), with or without delphinidin (Del, 20 µM), NF-κB inhibitor (BAY-11, 1 µM) or MAPK inhibitors. (A) p50 protein production was determined by Western blot. (B) The protein expressions of Fibronectin and α-SMA were examined by Western blot. (C) The total amount of collagen was measured by Sircol assay. (D) The transcriptional activity of NF-κB was measured by luciferase assay. Values are the means ±SEM of the 3 independent experiments. *P<0.05 vs control; †P<0.05 vs TGF-β1.
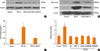
ACKNOWLEDGMENTS
This research was supported by Basic Science Research Program through the National Research Foundation of Korea (NRF) funded by the Ministry of Education (2013R1A1A2059716).
References
1. Pawankar R. Nasal polyposis: an update: editorial review. Curr Opin Allergy Clin Immunol. 2003; 3:1–6.
2. Chaaban MR, Walsh EM, Woodworth BA. Epidemiology and differential diagnosis of nasal polyps. Am J Rhinol Allergy. 2013; 27:473–478.
3. Rinia AB, Kostamo K, Ebbens FA, van Drunen CM, Fokkens WJ. Nasal polyposis: a cellular-based approach to answering questions. Allergy. 2007; 62:348–358.
4. Kendall RT, Feghali-Bostwick CA. Fibroblasts in fibrosis: novel roles and mediators. Front Pharmacol. 2014; 5:123.
5. Haruna S, Nakanishi M, Otori N, Moriyama H. Histopathological features of nasal polyps with asthma association: an immunohistochemical study. Am J Rhinol. 2004; 18:165–172.
6. Serpero L, Petecchia L, Sabatini F, Giuliani M, Silvestri M, Di Blasi P, et al. The effect of transforming growth factor (TGF)-beta1 and (TGF)-beta2 on nasal polyp fibroblast activities involved upper airway remodeling: modulation by fluticasone propionate. Immunol Lett. 2006; 105:61–67.
7. Little SC, Early SB, Woodard CR, Shonka DC Jr, Han JK, Borish L, et al. Dual action of TGF-beta1 on nasal-polyp derived fibroblasts. Laryngoscope. 2008; 118:320–324.
8. Van Bruaene N, Derycke L, Perez-Novo CA, Gevaert P, Holtappels G, De Ruyck N, et al. TGF-beta signaling and collagen deposition in chronic rhinosinusitis. J Allergy Clin Immunol. 2009; 124:253–259. 259.e1–259.e2.
9. Park HH, Park IH, Cho JS, Lee YM, Lee HM. The effect of macrolides on myofibroblast differentiation and collagen production in nasal polyp-derived fibroblasts. Am J Rhinol Allergy. 2010; 24:348–353.
10. Afaq F, Syed DN, Malik A, Hadi N, Sarfaraz S, Kweon MH, et al. Delphinidin, an anthocyanidin in pigmented fruits and vegetables, protects human HaCaT keratinocytes and mouse skin against UVB-mediated oxidative stress and apoptosis. J Invest Dermatol. 2007; 127:222–232.
11. Seong AR, Yoo JY, Choi K, Lee MH, Lee YH, Lee J, et al. Delphinidin, a specific inhibitor of histone acetyltransferase, suppresses inflammatory signaling via prevention of NF-κB acetylation in fibroblast-like synoviocyte MH7A cells. Biochem Biophys Res Commun. 2011; 410:581–586.
12. Domitrović R, Jakovac H. Antifibrotic activity of anthocyanidin delphinidin in carbon tetrachloride-induced hepatotoxicity in mice. Toxicology. 2010; 272:1–10.
13. Meng J, Zhou P, Liu Y, Liu F, Yi X, Liu S, et al. The development of nasal polyp disease involves early nasal mucosal inflammation and remodelling. PLoS One. 2013; 8:e82373.
14. Watelet JB, Claeys C, Perez-Novo C, Gevaert P, Van Cauwenberge P, Bachert C. Transforming growth factor beta1 in nasal remodeling: differences between chronic rhinosinusitis and nasal polyposis. Am J Rhinol. 2004; 18:267–272.
15. Czaja AJ. Hepatic inflammation and progressive liver fibrosis in chronic liver disease. World J Gastroenterol. 2014; 20:2515–2532.
16. Havsteen BH. The biochemistry and medical significance of the flavonoids. Pharmacol Ther. 2002; 96:67–202.
17. Noda Y, Kaneyuki T, Mori A, Packer L. Antioxidant activities of pomegranate fruit extract and its anthocyanidins: delphinidin, cyanidin, and pelargonidin. J Agric Food Chem. 2002; 50:166–171.
18. Cho JS, Moon YM, Park IH, Um JY, Moon JH, Park SJ, et al. Epigenetic regulation of myofibroblast differentiation and extracellular matrix production in nasal polyp-derived fibroblasts. Clin Exp Allergy. 2012; 42:872–882.
19. Cho JS, Moon YM, Um JY, Moon JH, Park IH, Lee HM. Inhibitory effect of ginsenoside Rg1 on extracellular matrix production via extracellular signal-regulated protein kinase/activator protein 1 pathway in nasal polyp-derived fibroblasts. Exp Biol Med (Maywood). 2012; 237:663–669.
20. Harris WT, Kelly DR, Zhou Y, Wang D, MacEwen M, Hagood JS, et al. Myofibroblast differentiation and enhanced TGF-B signaling in cystic fibrosis lung disease. PLoS One. 2013; 8:e70196.
21. Kolb M, Bonniaud P, Galt T, Sime PJ, Kelly MM, Margetts PJ, et al. Differences in the fibrogenic response after transfer of active transforming growth factor-beta1 gene to lungs of "fibrosis-prone" and "fibrosis-resistant" mouse strains. Am J Respir Cell Mol Biol. 2002; 27:141–150.
22. Barbaro V, Ferrari S, Fasolo A, Ponzin D, Di Iorio E. Reconstruction of a human hemicornea through natural scaffolds compatible with the growth of corneal epithelial stem cells and stromal keratocytes. Mol Vis. 2009; 15:2084–2093.
23. Blobe GC, Schiemann WP, Lodish HF. Role of transforming growth factor beta in human disease. N Engl J Med. 2000; 342:1350–1358.
24. Moustakas A, Heldin CH. Non-Smad TGF-beta signals. J Cell Sci. 2005; 118:3573–3584.
25. Samarakoon R, Overstreet JM, Higgins PJ. TGF-β signaling in tissue fibrosis: redox controls, target genes and therapeutic opportunities. Cell Signal. 2013; 25:264–268.
26. Kubiczkova L, Sedlarikova L, Hajek R, Sevcikova S. TGF-β-an excellent servant but a bad master. J Transl Med. 2012; 10:183.