Abstract
Purpose
Bacillus Calmette-Guérin (BCG) is known to suppress the asthmatic responses in a murine model of asthma and to induce dendritic cells (DCs) maturation. Mature DCs play a crucial role in the differentiation of regulatory T cells (Tregs), which are known to regulate allergic inflammatory responses. To investigate whether BCG regulates Tregs in a DCs-mediated manner, we analyzed in a murine model of asthma.
Methods
BALB/c mice were injected intraperitoneally with BCG or intravenously with BCG-stimulated DCs and then sensitized and challenged with ovalbumin (OVA). Mice were analysed for bronchial hyperresponsiveness (BHR), the influx of inflammatory cells in the bronchoalveolar lavage (BAL) fluid, and histopathological changes in the lung. To identify the mechanisms, IgE, IgG1 and IgG2a in the serum were analysed and the CD25+ Tregs in the mice were depleted with anti-CD25 monoclonal antibody (mAb).
Asthma is a chronic inflammatory disease that is characterized by infiltration of inflammatory cells such as eosinophils, airway hypersensitivity, and airflow obstruction.1,2 Th2 cells play a particularly crucial role in the development of asthma by secreting interleukin (IL)-4, IL-5, and IL-13: IL-4 causes naïve T cells to differentiate into Th2 cells while IL-5 induces eosinophil infiltration.3
The hygiene hypothesis proposes that westernized culture and its associated improvements in sanitation cause the microbial exposure of the population to be reduced. This decreases Th1 immune responses that are induced, the lack of which causes the immune system to become dominated by Th2 immune responses.4,5,6 It has been suggested that this skewing of the immune system has caused the incidence of allergic diseases such as asthma to rise rapidly in the last few decades.7,8,9 However, recent studies suggest that the incidence of Th1-related diseases such as type 1 diabetes mellitus and multiple sclerosis is also increasing along with Th2-related diseases such as asthma.10 This indicates that other mechanisms apart from Th1/Th2 immune responses participate in the modulation of allergic inflammatory responses. One of these mechanisms may involve regulatory T cells (Tregs): several studies report that Tregs regulate immune system by suppression of the Th1/Th2 responses.11,12,13 Tregs, which are widely considered to be crucial suppressor T cells, act by releasing the immunosuppressive cytokines IL-10 and transforming growth factor (TGF)-β14 and by cell-cell contact mechanism that is mediated cytotoxic lymphocyte associated antigen-4 (CTLA-4) expressed by CD4+CD25+ Tregs, which interacts with CD80 and/or CD86 on the surface of antigen-presenting cells (APCs).15
Bacillus Calmette-Guérin (BCG) is a mycobacterial vaccine organism that is used to prevent tuberculosis. It is known to modulate allergic inflammatory responses.16,17 For example, infants with a BCG scar have a higher anti-inflammatory response, namely their ratio of IL-10-producing T cells to IL-4-producing T cells is higher than the ratio of controls who lack a BCG scar.18 Significantly, analyses of mice who were immunised with BCG in the neonatal period revealed that their splenocytes had a higher frequency of CD4+CD25+ Tregs, expressed more Foxp3 and CTLA-4, and produced more IL-10 and TGF-β.19 Thus, BCG may be an example of the microbial exposure that is proposed in the hygiene hypothesis to prevent susceptibility to allergic inflammation.
Dendritic cells (DCs) are APCs that play an important role in modulating immune responses.20,21,22,23 DCs can be divided into immature and mature DCs. Contact with antigens can cause immature DCs to differentiate into mature DCs that express on their cell surfaces CD80, CD86, CD40, and major histocompatibility (MHC) class II markers.
Many studies have reported that depending on the stimuli, mature DCs can prime naïve T cells toward Tregs. Mature bone marrow-derived DCs (BMDCs) were able to induce the proliferation of CD4+CD25+ T cells in the presence of a polyclonal stimulus and in the absence of exogenous IL-2.24 Thymic stromal lymphopoietin-treated thymic DCs express CD80/86 and MHC class II and promote the conversion of CD4+CD25- thymocytes into CD4+CD25+Foxp3+ Tregs.25 Mature human monocytes-derived DCs upregulate the immune-inhibitory enzyme, indoleamine 2, 3-dioxygenase and expand CD4+CD25bright Foxp3+CD127neg Tregs.26
We confirmed previously that vaccination with BCG can suppress asthma-related inflammatory responses in a mouse model of asthma.27 The aim of the present study was to investigate the mechanism by which BCG vaccination inhibits the inflammatory allergic responses of this mouse model; to do so, the roles of DCs and Tregs were analysed.
Female BALB/c mice were purchased from Orient Bio (Seongnam, Korea) and cared for and used in accordance with the guidelines of the Institutional Animal Care and Use Committee (IACUC) at Asan Medical Center and Ulsan University College of Medicine. The mice were housed in specific pathogen-free facility.
The mice (6 weeks of age, 5 mice/group) were sensitized by 2 intraperitoneal injections with 10 µg ovalbumin (OVA; chicken egg albumin grade V, Sigma Chemical Co., St. Louis, USA) dissolved in 100 µL of phosphate-buffered saline (PBS) adsorbed to an equal volume of alum solution (Alumimject, Pierce, IL, USA) on days 0 and 7. On days 14-16, all mice were challenged daily for 30 minutes via an ultrasonic sprayer (Nescosonic UN-511, Alfresa, Osaka, Japan) with aerosolized 1% OVA (50 µg in 50 µL saline). The mice were divided into 5 groups of 5 mice each.
Freeze-dried living BCG vaccine was obtained from Japan BCG laboratory (Tokyo, Japan). The mice were injected intraperitoneally with BCG Tokyo 172 strain 5 days before the first sensitization (day -5) at a dose of 1×106 CFU/200 µL.
BMDCs were generated from BALB/c (6-8-weeks-old). Briefly, bone marrow cells were cultured in Iscove's Modified Dulbecco's Medium (IMDM) supplemented with 10% fetal bovine serum (FBS), 1% L-glutamine, antibiotic-antimycotic, and 10 ng/mL recombinant murine Granulocyte-macrophage colony-stimulating factor (GM-CSF) (R&D systems, Minneapolis, MN, USA). The culture media were replaced with fresh media containing GM-CSF on days 3 and 6. On day 6, BMDCs were collected and stained for CD11c. then, the CD11c+ cells were sorted by using autoMACS (Miltenyi Biotec) and prepared at 1×106 cells/10 mL, and stimulated with BCG (1×107 CFU) for 48 hours in vitro. The mice were injected intravenously with BCG-stimulated DCs or non-stimulated DCs (1×106/200 µL) 5 days before the first sensitization (day -5).
The administration of anti-CD25 Abs is a commonly used method to deplete Tregs in vivo.28 To investigate the mechanism by which Tregs participate in the allergy-suppressive effects of vaccination with BCG or BCG-stimulated DCs, the OVA-sensitized mice were injected intraperitoneally with 250 µg of anti-CD25 monoclonal antibody (mAb) (clone PC61, eBioscience, San Diego, USA) 1 day before the first challenge with 1% OVA.
Twenty-four hours after the final OVA challenge, BHR was measured in conscious unrestrained mice by using a barometric whole-body plethysmograph (one chamber plethysmography, All Medicus, Anyang, Korea), as described previously.29 Briefly, after inhalation of saline and each concentration of methacholine (MeCh), enhanced pause (Penh) was measured at 10-seconds intervals for 3 minutes, averaged, and expressed as absolute values.
After measurement of BHR, mice were anaesthetized. BAL was performed as described previously.29 Briefly, the trachea was immediately exposed after anesthesia. Then, BAL was performed through a catheter inserted into the exposed trachea following instillation of normal saline (2 mL) at 37℃. The BAL fluid was centrifuged at 2,000 rpm for 5min at 4℃. After discarding the supernatant, the pellet was resuspended in 100 µL PBS. Total BAL cell counts were performed using a haemacytometer. To count the different BAL fluid cells, cytospin slides were prepared, stained with Wright stain, and the different cell types were identified on the basis of their standard morphology under a light microscope. Five sections per slide were evaluated.
Serum was collected upon sacrifice 24 hours after last 1% OVA challenge and the serum immunoglobulins were quantified by sandwich ELISA as described previously.29 Briefly, serum was separated from the blood clot by centrifugation at 2,500 rpm for 10 minutes at 4℃. Absorbance of Total IgE, OVA-specific IgE, IgG1 and IgG2a was measured using ELISA reader. The optical density was measured at 450 nm.
To histologically evaluate the lung tissues, lung samples were prepared as described previously.30 Inflammation was scored by 2 independent blinded investigators. The degree of peribronchial and perivascular inflammation was evaluated on a subjective scale of 0-3 as described elsewhere.30 Briefly, a value of 0 meant that no inflammation was detectable, a value of 1, occasional cuffing with inflammatory cells, a value of 2, most bronchi or vessels were surrounded by thin layer (1 to 5 cells thick) of inflammatory cells, and a value of 3, most bronchi or vessels were surrounded by a thick layer (more than 5 cells thick) of inflammatory cells. The cellular infiltration in 5 randomly selected fields was assessed under a Zeiss Axiophot microscope (Carl Zeiss Inc., Thornwood, USA) at 100×.
The MeCh-BHR in response to aerosolized MeCh was measured and the cells in the BAL fluid were identified and counted. Mean Penh value at maximum MeCh dose of the BCG-stimulated DCs mice was half those of mice, which had only been sensitized and challenged with OVA (OVA mice) (P<0.05, Fig. 1A). By contrast, the mean Penh values at maximum MeCh dose of the mice who had received non-stimulated DCs before OVA sensitization and challenge were equivalent to those of the OVA mice. In terms of the BAL fluid analyses, the mice receiving the BCG-stimulated DCs had significantly fewer infiltrating cells overall than the OVA mice (P<0.01, Fig. 1B), whereas the mice receiving non-stimulated DCs were similar to the OVA mice. With respect to specific cell types in the BAL fluid, the mice receiving the BCG-stimulated DCs had significantly fewer eosinophils than the OVA mice and the mice receiving non-stimulated DCs (P<0.01, Fig. 1B).
Histological analysis of the lungs of the mice described above revealed that the recruitment to the lungs of peribronchial and perivascular inflammatory cells was low in mice who had received the BCG-stimulated DCs when compared to the OVA mice (Fig. 1C). In contrast, the recruitment of the mice that received the non-stimulated DCs was equivalent to that of the OVA mice (Fig. 1C).
To quantify the infiltration of inflammatory cells, peribronchial and perivascular histopathological inflammation scores were determined. Compared to the OVA mice, the inflammation score was low in the mice that had received BCG-stimulated DCs (P<0.01, Fig. 1D). By contrast, the scores of the mice that had received the non-stimulated DCs were similar to those of the OVA mice (Fig. 1D).
The levels of total IgE, OVA-specific IgE, OVA-specific IgG1, and OVA-specific IgG2a in the sera of each group were measured. The OVA mice had elevated levels of total IgE and OVA-specific IgE. Both measures were significantly lower in the mice that received the BCG-stimulated DCs but not in the mice that received the non-stimulated DCs (P<0.05, P<0.01, respectively, Fig. 2). Compared to the OVA mice, the mice that received the BCG-stimulated DCs also tended to have lower OVA-specific IgG2a levels and their OVA-specific IgG1 levels were significantly lower (P<0.05, Fig. 2). By contrast, the OVA-specific IgG1 and OVA-specific IgG2a levels of the mice that received the non-stimulated DCs were similar to those of the OVA mice (Fig. 2).
BHR analysis revealed that the mean Penh values at maximum MeCh dose of the CD25-depleted mice were significantly higher than those of the BCG-DCs-injected mice (P<0.05, Fig 3A). BAL fluid analysis revealed that the anti-CD25 mAb treatment also significantly increase the total cell numbers and eosinophils relative to the mice that treated the BCG-stimulated DCs (P<0.01, respectively, Fig. 3B).
There was significantly more recruitment of inflammatory cells to the lungs in the anti-CD25 mAb treated mice than in the BCG-stimulated DCs-treated mice (P<0.01, Fig. 3C and D).
Many studies in human and experimental work in animals showed that BCG can modulate the allergic inflammatory responses.16,17,18 However, the mechanism(s) by which this occurs remained unclear. In the present study, we asked whether this mechanism is associated with Tregs that are stimulated by BCG-stimulated DCs.
The present study shows that BCG vaccination and the transfer of BCG-stimulated DCs both inhibited asthmatic responses, including BHR, serum IgE production, and the numbers of inflammatory cells in the BAL fluid and lungs. In addition, treatment with anti-CD25 mAb prior to challenge abrogated these effects. These findings suggest that BCG inhibits allergic inflammatory responses by inducing Tregs, which is mediated by DCs.
The first of these experiments involved injecting mice with BCG-stimulated DCs, as this would reveal whether the inhibitory effect of BCG is associated with DCs. Indeed, compared to the OVA mice and the mice that received non-stimulated DCs, the transfer of BCG-stimulated DCs significantly suppressed the asthmatic responses, including BHR, the numbers of inflammatory cells in the BAL fluid, and the pulmonary inflammation in the lung tissue. This effect of BCG has also been observed by other studies.31,32,33 These results suggest that the BCG-induced maturation of DCs plays an important role in regulating allergic immune responses.
Our second experiment involved the administration of anti-CD25 mAb to determine whether the inhibitory effect of the BCG-stimulated DCs is associated with Tregs. Cell-surface CD25 is a well-known marker for Tregs.34 Tregs depletion by CD25 mAb treatment indeed enhanced BHR and measures of airway inflammation, namely the total cell and eosinophil counts in the BAL fluid and the infiltration of inflammatory cells in the lung. Thus, it appears that the asthma-inhibiting effects of BCG are associated with Tregs. These observations are consistent with those of other studies that have used mouse models.35,36,37 For example, BCG-primed DCs were shown to enhance the expression of Foxp3+ Tregs, IL-10, and inducible costimulator.35 However, interestingly, BCG-primed DCs have also been shown to upregulate Th1-associated neutrophilic inflammation; this was more effective when the Pasteur strain was used instead of the Copenhagen strain.35 In the present study, the Tokyo strain of BCG was used and neutrophil increases were not observed. Thus, this difference may be related to the type of BCG strain that is used. Supporting this is a study that found considerable differences in the immunogenicity of various BCG strains, namely their ability to modulate T-cell proliferation and cytokine production.38
This study demonstrated that matured DCs by BCG in vitro could suppress allergic inflammation in mouse model of asthma with adoptive transfer. In light of these, our study provides a new perspective of the mechanisms by which the allergic inflammation in a mouse model of asthma can be modulated.
Further studies are needed to refine several aspects of this study. CD25 is not a specific marker of Tregs and it may be expressed by activated T and B cells. Therefore, the use of anti-CD25 may not be sufficient to explain the mechanism of Tregs.39 And we did not investigate the expression of Tregs in the airway or thymus or regional lymph node and the functional cytokine production. However, BCG treatment is likely to elevate Foxp3+ Tregs which is a master transcription factor of Tregs in vivo and in vitro.35,36 In addition, recent study has reported that DCs stimulated by BCG release IL-10 and induce differentiation of naïve T cells into Tregs.39
BCG is also recognized as a strong Th1 immune response inducer.41,42 A study has reported that the interaction between BCG and toll-like receptor (TLR) 2 on DCs may affect the differentiation of naïve T cell mainly to Th1-like cells with the help of IL-10, IL-12, and so forth.43 In addition, recently, some studies have reported that IFN-γ is essential to suppress allergic responses.44 It would be worth to perform the study of BCG-DCs on damping allergic inflammation in IFN-γ- or TLR2-deficient mice.
In summary, the present study showed that BCG treatment inhibited the allergic responses of a mouse model of asthma; the study with BCG-stimulated DCs was consistent with these observations. In addition, anti-CD25 mAb treatment reversed the effects of BCG/BCG-DCs. These results suggest that the inhibitory effect of BCG in our mouse model of asthma is mediated by DCs, which induce Tregs.
Figures and Tables
Fig. 1
Effect of treatment with Bacillus Calmette-Guerin (BCG) vaccine-stimulated DCs on the ovalbumin (OVA)-induced allergic asthma of mouse model. OVA: mice sensitized with intraperitoneal injections of OVA on days 0 and 7 and then challenged with aerosolized OVA on days 14-16; Saline: mice sensitized and challenged with saline following the same time schedule described for OVA mice; BGC-DC: mice that were injected intravenously with BCG vaccine-stimulated DCs on day -5 and then sensitized and challenged with OVA; Non-DC: mice that were injected intravenously with unstimulated DCs on day -5 and then sensitized and challenged with OVA; BCG: mice injected intraperitoneally with BCG vaccine emulsified in alum and then sensitized and challenged with OVA (A) Brochial hyperresponsiveness (BHR), *P<0.05, **P<0.01 compared to the OVA mice; †P<0.01 compared to the BCG-DC-treated mice. (B) Differential cell counts in the Bronchoalvoelar lavage (BAL) fluid. (C) Lung pathology. (D) Peribronchial and perivascular lung inflammation scores. The values are the means±SD of the results from 5 mice per group. *P<0.05, **P<0.01.
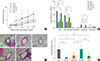
Fig. 2
Effect of treatment with BCG vaccine-stimulated DCs on the serum levels of immunoglobulins in mouse model of allergic asthma. The plot legends are as described in Fig. 1. BCG vaccine-stimulated DC transfer inhibited total IgE, OVA-specific IgE and OVA- specific IgG1 levels compared to OVA mice. The values are the means±SD of the results from 5 mice per group. *P<0.05, **P<0.01.
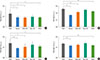
Fig. 3
Effect of anti-CD25 monoclonal antibody (mAb) treatment on the allergic asthma of mice injected with BCG vaccine-stimulated DCs and then sensitized and challenged with OVA. The mice were injected intraperitoneally with anti-CD25 mAb before challenge with OVA. The plot legends are as described in Fig. 1 except that BCG-DC+ anti-CD25 indicate mice that received BCG-DCs and the anti-CD25 mAb. (A) BHR, *P<0.05, **P<0.01 compared to the OVA mice; †P<0.05 compared to the BCG-DC-treated mice. (B) Differential cell counts in the BAL fluid. (C) Lung pathology. (D) Peribronchial and perivascular lung inflammation scores. The values shown are the means±SD of the results from 5 mice per group. *P<0.05, **P<0.01.
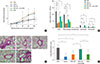
ACKNOWLEDGMENTS
All authors declare there are no conflicts of interest. This study was supported by a grant from the Korea Healthcare Technology R&D Project of the Ministry for Health, Welfare and Family Affairs, Republic of Korea (A084144).
References
1. Walker C, Bode E, Boer L, Hansel TT, Blaser K, Virchow JC Jr. Allergic and nonallergic asthmatics have distinct patterns of T-cell activation and cytokine production in peripheral blood and bronchoalveolar lavage. Am Rev Respir Dis. 1992; 146:109–115.
2. Lee SH, Park JS, Park CS. The search for genetic variants and epigenetics related to asthma. Allergy Asthma Immunol Res. 2011; 3:236–244.
3. Wang JM, Rambaldi A, Biondi A, Chen ZG, Sanderson CJ, Mantovani A. Recombinant human interleukin 5 is a selective eosinophil chemoattractant. Eur J Immunol. 1989; 19:701–705.
4. Strachan DP. Hay fever, hygiene, and household size. BMJ. 1989; 299:1259–1260.
5. Riedler J, Braun-Fahrlnder C, Eder W, Schreuer M, Waser M, Maisch S, Carr D, Schierl R, Nowak D, von Mutius E. ALEX Study Team. Exposure to farming in early life and development of asthma and allergy: a cross-sectional survey. Lancet. 2001; 358:1129–1133.
6. Ege MJ, Mayer M, Normand AC, Genuneit J, Cookson WO, Braun-Fahrlnder C, Heederik D, Piarroux R, von Mutius E. GABRIELA Transregio 22 Study Group. Exposure to environmental microorganisms and childhood asthma. N Engl J Med. 2011; 364:701–709.
7. Eder W, Ege MJ, von Mutius E. The asthma epidemic. N Engl J Med. 2006; 355:2226–2235.
8. Hong SJ, Ahn KM, Lee SY, Kim KE. The prevalences of asthma and allergic diseases in Korean children. Korean J Pediatr. 2008; 51:343–350.
9. Lee SI. Prevalence of childhood asthma in Korea: international study of asthma and allergies in childhood. Allergy Asthma Immunol Res. 2010; 2:61–64.
10. Bach JF. The effect of infections on susceptibility to autoimmune and allergic diseases. N Engl J Med. 2002; 347:911–920.
11. Seroogy CM, Gern JE. The role of T regulatory cells in asthma. J Allergy Clin Immunol. 2005; 116:996–999.
12. Curotto de Lafaille MA, Kutchukhidze N, Shen S, Ding Y, Yee H, Lafaille JJ. Adaptive Foxp3+ regulatory T cell-dependent and -independent control of allergic inflammation. Immunity. 2008; 29:114–126.
13. Robinson DS, Larch M, Durham SR. Tregs and allergic disease. J Clin Invest. 2004; 114:1389–1397.
14. Kwon HK, Lee CG, So JS, Chae CS, Hwang JS, Sahoo A, Nam JH, Rhee JH, Hwang KC, Im SH. Generation of regulatory dendritic cells and CD4+Foxp3+ T cells by probiotics administration suppresses immune disorders. Proc Natl Acad Sci U S A. 2010; 107:2159–2164.
15. Read S, Malmstrm V, Powrie F. Cytotoxic T lymphocyte-associated antigen 4 plays an essential role in the function of CD25(+)CD4(+) regulatory cells that control intestinal inflammation. J Exp Med. 2000; 192:295–302.
16. Hopfenspirger MT, Agrawal DK. Airway hyperresponsiveness, late allergic response, and eosinophilia are reversed with mycobacterial antigens in ovalbumin-presensitized mice. J Immunol. 2002; 168:2516–2522.
17. Erb KJ, Holloway JW, Sobeck A, Moll H, Le Gros G. Infection of mice with Mycobacterium bovis-Bacillus Calmette-Guérin (BCG) suppresses allergen-induced airway eosinophilia. J Exp Med. 1998; 187:561–569.
18. Jason J, Archibald LK, Nwanyanwu OC, Kazembe PN, Chatt JA, Norton E, Dobbie H, Jarvis WR. Clinical and immune impact of Mycobacterium bovis BCG vaccination scarring. Infect Immun. 2002; 70:6188–6195.
19. Li Q, Shen HH. Neonatal bacillus Calmette-Guérin vaccination inhibits de novo allergic inflammatory response in mice via alteration of CD4+CD25+ T-regulatory cells. Acta Pharmacol Sin. 2009; 30:125–133.
20. Hammad H, Lambrecht BN. Dendritic cells and epithelial cells: linking innate and adaptive immunity in asthma. Nat Rev Immunol. 2008; 8:193–204.
21. Geijtenbeek TB, Torensma R, van Vliet SJ, van Duijnhoven GC, Adema GJ, van Kooyk Y, Figdor CG. Identification of DC-SIGN, a novel dendritic cell-specific ICAM-3 receptor that supports primary immune responses. Cell. 2000; 100:575–585.
22. Steinman RM, Hawiger D, Nussenzweig MC. Tolerogenic dendritic cells. Annu Rev Immunol. 2003; 21:685–711.
23. Banchereau J, Steinman RM. Dendritic cells and the control of immunity. Nature. 1998; 392:245–252.
24. Brinster C, Shevach EM. Bone marrow-derived dendritic cells reverse the anergic state of CD4+CD25+ T cells without reversing their suppressive function. J Immunol. 2005; 175:7332–7340.
25. Watanabe N, Wang YH, Lee HK, Ito T, Wang YH, Cao W, Liu YJ. Hassall's corpuscles instruct dendritic cells to induce CD4+CD25+ regulatory T cells in human thymus. Nature. 2005; 436:1181–1185.
26. Chung DJ, Rossi M, Romano E, Ghith J, Yuan J, Munn DH, Young JW. Indoleamine 2,3-dioxygenase-expressing mature human monocyte-derived dendritic cells expand potent autologous regulatory T cells. Blood. 2009; 114:555–563.
27. Kim YJ, Kim HJ, Kang MJ, Kwon JW, Seo JH, Kim BJ, Yu J, Hong SJ. Effects of Bacillus Calmette-Guérin (BCG) vaccination according to time point of administration on asthma in a murine model. Korean J Asthma Allergy Clin Immunol. 2010; 30:307–313.
28. Choi MS, Park S, Choi T, Lee G, Haam KK, Hong MC, Min BI, Bae H. Bee venom ameliorates ovalbumin induced allergic asthma via modulating CD4+CD25+ regulatory T cells in mice. Cytokine. 2013; 61:256–265.
29. Yu J, Jang SO, Kim BJ, Song YH, Kwon JW, Kang MJ, Choi WA, Jung HD, Hong SJ. The effects of Lactobacillus rhamnosus on the prevention of asthma in a murine model. Allergy Asthma Immunol Res. 2010; 2:199–205.
30. Tournoy KG, Kips JC, Schou C, Pauwels RA. Airway eosinophilia is not a requirement for allergen-induced airway hyperresponsiveness. Clin Exp Allergy. 2000; 30:79–85.
31. Soualhine H, Deghmane AE, Sun J, Mak K, Talal A, Av-Gay Y, Hmama Z. Mycobacterium bovis bacillus Calmette-Guérin secreting active cathepsin S stimulates expression of mature MHC class II molecules and antigen presentation in human macrophages. J Immunol. 2007; 179:5137–5145.
32. Liu E, Law HK, Lau YL. BCG promotes cord blood monocyte-derived dendritic cell maturation with nuclear Rel-B up-regulation and cytosolic I kappa B alpha and beta degradation. Pediatr Res. 2003; 54:105–112.
33. Demangel C, Bean AG, Martin E, Feng CG, Kamath AT, Britton WJ. Protection against aerosol Mycobacterium tuberculosis infection using Mycobacterium bovis Bacillus Calmette Guérin-infected dendritic cells. Eur J Immunol. 1999; 29:1972–1979.
34. Han Y, Guo Q, Zhang M, Chen Z, Cao X. CD69+ CD4+ CD25- T cells, a new subset of regulatory T cells, suppress T cell proliferation through membrane-bound TGF-beta 1. J Immunol. 2009; 182:111–120.
35. Ahrens B, Gruber C, Rha RD, Freund T, Quarcoo D, Awagyan A, Hutloff A, Dittrich AM, Wahn U, Hamelmann E. BCG priming of dendritic cells enhances T regulatory and Th1 function and suppresses allergen-induced Th2 function in vitro and in vivo. Int Arch Allergy Immunol. 2009; 150:210–220.
36. Lagranderie M, Abolhassani M, Vanoirbeek JA, Lima C, Balazuc AM, Vargaftig BB, Marchal G. Mycobacterium bovis bacillus Calmette-Guérin killed by extended freeze-drying targets plasmacytoid dendritic cells to regulate lung inflammation. J Immunol. 2010; 184:1062–1070.
37. Lewkowich IP, Herman NS, Schleifer KW, Dance MP, Chen BL, Dienger KM, Sproles AA, Shah JS, Khl J, Belkaid Y, Wills-Karp M. CD4+CD25+ T cells protect against experimentally induced asthma and alter pulmonary dendritic cell phenotype and function. J Exp Med. 2005; 202:1549–1561.
38. Lagranderie MR, Balazuc AM, Deriaud E, Leclerc CD, Gheorghiu M. Comparison of immune responses of mice immunized with five different Mycobacterium bovis BCG vaccine strains. Infect Immun. 1996; 64:1–9.
39. Fehérvari Z, Sakaguchi S. Development and function of CD25+CD4+ regulatory T cells. Curr Opin Immunol. 2004; 16:203–208.
40. Gao X, Bai H, Cheng J, Fan Y, Wang S, Jiao L, Xiu N, Yang X. CD8α+ and CD8α- DC subsets from BCG-infected mice inhibit allergic Th2-cell responses by enhancing Th1-cell and Treg-cell activity respectively. Eur J Immunol. 2012; 42:165–175.
41. Orme IM, Andersen P, Boom WH. T cell response to Mycobacterium tuberculosis. J Infect Dis. 1993; 167:1481–1497.
42. Christ AP, Rodriguez D, Bortolatto J, Borducchi E, Keller A, Mucida D, Silva JS, Leite LC, Russo M. Enhancement of Th1 lung immunity induced by recombinant Mycobacterium bovis Bacillus Calmette-Guérin attenuates airway allergic disease. Am J Respir Cell Mol Biol. 2010; 43:243–252.
43. Barlan I, Bahceciler NN, Akdis M, Akdis CA. Bacillus Calmette-Guérin, Mycobacterium bovis, as an immunomodulator in atopic diseases. Immunol Allergy Clin North Am. 2006; 26:365–377.
44. Fonseca DM, Paula MO, Wowk PF, Campos LW, Gembre AF, Turato WM, Ramos SG, Dias-Baruffi M, Barboza R, Gomes E, Horn C, Marchal G, Arruda LK, Russo M, Bonato VL. IFN-γ-mediated efficacy of allergen-free immunotherapy using mycobacterial antigens and CpG-ODN. Immunol Cell Biol. 2011; 89:777–785.