Abstract
Echovirus 30 is one of the major causes of meningitis in children and adults. The purpose of our current study was to investigate whether selected antiviral drugs could provide antiviral activity against echovirus 30. Using RD cells, we assessed the cytopathic effect of echovirus 30, including viral RNA levels as indicators of viral replication. The effects of gemcitabine were compared to rupintrivir, a well-known antiviral drug. To understand the activity gemcitabine exerts on the viral life cycle, time course and time-of-addition assays were implemented. The most effective compounds against echovirus 30 were gemcitabine and rupintrivir, as demonstrated by their concentration-dependent activity. Gemcitabine affects the early stages of echovirus 30 infection by disrupting viral replication. However, gemcitabine failed to directly inactivate echovirus 30 particles or impede viral uptake into the RD cells. Gemcitabine can be considered as a lead candidate in the development of echovirus 30 antiviral drugs, specifically in the early stages of echovirus 30 replication.
Human enteroviruses (EVs) are positive-sense, single-stranded, RNA viruses and belong to Picornaviridae (1). Sequence analysis of human enteroviruses further grouped them into four species (EV-A to D). Among these, six serotypes of EV-A (CAV2, 4, 6, 10, 16 and EV71) and four serotypes of EV-B (E6, 9, 30 and CAV9) have been detected in young children with sepsis-like illness across Edinburgh and the US (234).
Echovirus 30 (E30) is a key pathogen related with aseptic meningitis in humans (56). It is highly prevalent on a global scale (789). The transmission routes of E30 may be direct such as fecal-oral and respiratory routes or indirect, such as via contaminated water (10).
Primary infection with E30 leads to replication of the virus in the tissue around the gastrointestinal tract, with transient viremia following (11). Infectious diseases caused by pathogenic organisms such as viruses are still responsible for the majority of hospitalization and death worldwide. Although considerable advancement has been made by using various antibiotic agents, there remain big challenges in the effective treatment of various infections (1213). In addition to numerous side effects in the use of antiviral agents due to their effect on the whole body, the rapid emergence of drug resistance adds a serious problem to human health (1415). Moreover, new viruses are constantly emerging through evolution or other biological events, leading to a continuing challenge in the control and prevention of infectious diseases. Consequently, in addition to discovering novel antiviral drugs for infection control, new strategies need to be expanded to maximize efficacy of the existing available drugs (16).
Gemcitabine is widely utilized in the treatment of pancreatic, ovarian, and non-small cell lung cancers (17). To date, however, no reports have described the antiviral activity of gemcitabine against E30. In this study, we assess the antiviral activity of gemcitabine against E30, and further elucidate its mechanism of action.
E30 (ATCC, VR-692) was purchased from American Type Culture Collection (Manassas, VA, USA) and grown at 37℃ in RD cells (human rhabdomyosarcoma cell line). RD cells were cultured in minimal essential medium (MEM), supplemented with 10% fetal bovine serum (FBS) and 1% antibiotic-antimycotic solution. MEM, FBS, trypsin-EDTA, and antibiotic-antimycotic solution were purchased from Gibco BRL (Invitrogen Life Technologies, Karlsruhe, Germany) and tissue culture plates were purchased from Falcon (BD Biosciences, San Jose, CA, USA).
Antiviral activity was determined by sulforhodamine B (SRB) staining using the cytopathic effect (CPE) reduction assay method, following viral infection (18). One day before infection, RD cells (at 2×104 cells/well) were seeded onto a 96-well plate (Falcon, BD biosciences, San Jose, CA, USA). The next day, culture medium was altered to 1% FBS and the virus suspension was added at a 50% tissue culture infective dose (TCID50). An appropriate concentration of the gemcitabine was added to the plate which was then cultured at 37℃ in a CO2 incubator for 2 days until the appropriate CPE was generated. To fix cells, ice-cold 70% acetone was added to the plate. After 30 min, the fixed cells were stained using SRB solution (0.4% (w/v) in 1% acetic acid solution. To solubilize bound SRB, 10 mM unbuffered Tris base solution was added to the plate. The absorbance was measured at 562 nm by a SpectraMax i3 microplate reader (Molecular Devices, Palo Alto, CA, USA) with a reference absorbance at 650 nm. Cell viability (%) was calculated for comparison, based on the optical density measured. In addition, images of SRB-stained cells were recorded using ImmunoSpot (CTL, ShakerHeights, OH, USA).
To test the effect of gemcitabine on the infectivity of E30 particles, E30 was pre-incubated with gemcitabine or rupintrivir at 4℃. After 1 h, RD cells were treated with pretreated or untreated E30 at 37℃ and following a further 1h, unbound virus was eliminated. After washing using 2x PBS, RD cells were incubated in medium provided with or without gemcitabine or rupintrivir at 37℃. Antiviral activity was measured using the SRB assay 2 days following (19).
RD cells infected with TCID50 E30 were harvested at various time points, including 4, 6, 8, 10, and 12 h post-infection, after which gemcitabine and rupintrivir were added. Total RNA was isolated at the indicated time points post-infection. The levels of E30 RNA were analyzed using real time-PCR.
Gemcitabine and rupintrivir were then added onto the cells at 10 µM or 2 µM, respectively, either before (−1 h), during (0 h), or after (1, 2, 4, 6, 8, 10 and 12 h) E30 infection. After 14 h, real-time (RT−) PCR analysis was conducted using Thunderbird SYBR qPCR Mix (Toyobo, Osaka, Japan).
Total RNA was extracted using the QIAamp viral RNA Mini kit (Qiagen, Valencia, CA, USA). The reverse transcription reaction was generated using an RNA 20µl reaction volume for 60 min at 42℃, containing RNase inhibitor, murine Maloney leukemia virus reverse transcriptase with 5×buffer, oligo(dT) 15 primer and a dNTP mixture (all from Promega, Madison, WI, USA) according to the manufacturer's instructions. Quantitative RT-PCR analysis was conducted and cDNA was amplified using the CFX96 optical reaction real-time PCR system (Bio-Rad, Hercules, CA, USA) with Thunderbird SYBR qPCR Mix (Toyobo, Osaka, Japan). The following primers were used: E30 5′-NCR-sense, 5′-TCC TCC GGC CCC TGA ATG-3′ and 5′-NCR-antisense, 5′-GAA ACA CGG ACA CCC AAA G-3′ (20).
We recently reported that gemcitabine has antiviral activity against human rhinoviruses (21). Here we confirmed the antiviral effect of gemcitabine on E30 by assessing the virus-induced cytopathic effect in RD cells using an SRB assay. E30 induced approximately 90% cell death in RD cells, and treatment of cells with 2 µM of gemcitabine significantly increased cell viability up to 80%. Furthermore, 10 µM of gemcitabine fully preserved RD cells from cytotoxicity induced by E30 infection. Rupintrivir (positive control) exhibited strong antiviral activity against E30 up to a concentration of 0.016 µM (Fig. 1A). We also assessed the cytotoxicity of gemcitabine and rupintrivir against RD cells. Gemcitabine and rupintrivir did not show cytotoxicity at 50 µM and 2 µM respectively (Fig. 1B). Collectively, these results suggest that gemcitabine possesses antiviral activity against E30 in vitro.
Based on the results produced from the E30 in vitro assay, we conducted time course experiments to analyze the mechanism of action of gemcitabine. Viral RNA from E30 was detected using RT-PCR at various times points (4, 6, 8, 10, and 12 h post-infection) and after gemcitabine (10 µM) and rupintrivir (2 µM) treatment of E30-infected cells. We could find E30 viral RNA as early as 8 h post-infection. Interestingly, gemcitabine was seen to block the proliferation of E30 in resemblance to rupintrivir (inhibitor of picornavirus 3C protease, Fig. 2). Based on the results of the time course experiments, we hypothesized that the antiviral effect of gemcitabine occurs relatively early in the infection process. To observe which step is influenced by gemcitabine, we performed a time-of-addition experiment. Gemcitabine (10 µM) and rupintrivir (2 µM) were added to the culture medium at −1, 0, 1, 2, 4, 6, 8, 10 and 12 h after virus infection. E30 RNA replication was analyzed at 14 h post-infection.
Treating cells with gemcitabine at -1 h in respect to E30 infection did not inhibit the virus. However, gemcitabine treatment 10 h post-infection effectively suppresses E30 viral infection. In contrast, viral RNA somewhat increased with gemcitabine treatment at 12 h post-infection. This result suggests that gemcitabine did not inhibit E30 replication at an early state of infection, but instead, inhibits the stage of viral infection which occurs at 10 h or earlier post-infection (Fig. 3).
The effects of gemcitabine on the infectivity of E30 particles were also investigated. The antiviral activity of E30 pre-incubated (−1 h) with gemcitabine and rupintrivir did not show significant results. In contrast, the sustained presence (0 h) of gemcitabine and rupintrivir during infection resulted in a marked increase in antiviral activity (Fig. 4). This result suggests that gemcitabine does not interact with E30 particles because pre-exposure of the virus to gemcitabine did not alter the infectivity of E30 particles.
Previously, it was reported that gemcitabine affects the intracellular levels of nucleotide triphosphate (NTP), especially decreasing CTP levels (22). Thus, we found that the addition of 50 µM ATP, GTP, CTP and UTP significantly reduced the cell viability of RD cells treated with gemcitabine after infection with E30 (Fig. 5A and 5D). We found that the addition of pyrimidine nucleotides, UTP and CTP significantly reduced the antiviral activity of gemcitabine (Fig. 5D). Thus, the addition of gemcitabine limits UTP and CTP levels and may therefore restrict RNA synthesis (Fig. 5C and 5D). Collectively, we found that the anti-viral activity of gemcitabine may be controlled by reducing the levels of CTP and UTP after having been converted into gemcitabine triphosphate.
Although vaccines are effective, immunity takes time to develop. Thus, they are of no use to the infected patient (23). Additionally, vaccines must be reformulated each year because of the antigenic drift and rendered ineffective when the formulation does not match the epidemic virus. For these reasons, some antivirals are sought to replace the vaccine by supplying long-continuous protection against both seasonal and pandemic viruses.
Gemcitabine is a nucleoside analog that inhibits cell growth by incorporating into DNA and preventing the action of DNA polymerases. It is approved for the treatment of multiple solid tumors (24). To date, however, the mechanism of action and effectiveness of gemcitabine against E30 have yet to be described. Therefore, we sought to examine whether gemcitabine possesses antiviral activity against E30 using RD cells. Gemcitabine did not show any cytotoxicity at concentrations of 0.4, 2, 10 and 50 µM. Treatment of cells with 10 µM of gemcitabine significantly increased the cell viability to 100%. Therefore, our results support the possibility of gemcitabine as a potential antiviral and therapeutic candidate.
Among the approaches used in determining the antiviral activity of gemcitabine, the time-of-addition assay identified inhibition of viral RNA replication as the mechanism of action. In our study, gemcitabine effectively inhibited the proliferation of echovirus 30, mirroring well-known rupintrivir, an inhibitor of picornavirus 3C protease. Gemcitabine does not suppress the entry of the virus into the cell, but instead inhibits the stage of the viral life cycle that occurs no more than 10h after infection. Therefore, we concluded that the antiviral effect of gemcitabine occurs relatively early during viral infection.
To realize the potential of gemcitabine as an antiviral candidate, its mechanism of activity must be understood. It is important to differentiate between viral inactivation (virucidal activity) from antiviral activity. Direct viral inactivation is an early event where the virus is inactivated before it infects the cells while antiviral activity involves killing the virus or the suppression of viral replication. It would be ideal for viral infection treatments to possess both virucidal and antiviral activities. In our study, gemcitabine did not directly interact with E30 particles as pre-exposure of the virus to gemcitabine did not alter the infectivity of E30 particles. Furthermore, addition of UTP and CTP significantly reduced the antiviral activity of gemcitabine by increasing the levels of pyrimidine nucleotides. The anti-echoviral activity of gemcitabine may be altered by reducing the levels of CTP and UTP after having being converted into gemcitabine triphosphate.
Thus, this study has revealed that gemcitabine could possess antiviral activity against E30. It does not directly inactivate E30 particles, but affects the initial stages of E30 infection by interfering with viral replication. The antiviral activity induced by gemcitabine treatment may be adjusted by reducing the levels of CTP and UTP after it has been converted into gemcitabine triphosphate. These results could be useful in the design of new inhibitors for use as anti-viral agents.
Figures and Tables
Figure 1
Gemcitabine-mediated antiviral activity against E30 in vitro. (A) RD cells were infected with the TCID50 (50 % cell culture infective dose) of E30, and treated with indicated concentrations of gemcitabine and rupintrivir. Cell viability was evaluated using an SRB assay, and results were determined based on absorbance at 524nm. Bar graphs show mean ± SD. Control (Ctrl) was neither infected nor treated, while vehicle (Veh) was infected with E30 but not treated with gemcitabine and rupintrivir. (B) The cytotoxicity of gemcitabine was evaluated using an SRB assay in RD cells. ***P<0.001, based on ANOVA with Bonferroni's multiple comparison test.
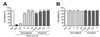
Figure 2
Time course of E30 infection. RD cells infected with the TCID50 of E30 were harvested at the indicated time points after addition of 10 µM gemcitabine, or 2 µM rupintrivir (post-infection). Total RNA was isolated and E30 RNA was analyzed using RT-qPCR. Real-time PCR analyses were performed to determine the effect of gemcitabine, or rupintrivir on E30 NCR gene expression levels. Each value is the result of mean ± S.D. of three independent experiments.
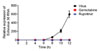
Figure 3
Time-of-addition experiment to test effect of gemcitabine on the E30 viral cycle. Gemcitabine (10 µM), or rupintrivir (2 µM) were added prior to, at the time of, or at indicated time points after viral infection of RD cells. The level of viral RNA expression was analyzed 14h post-infection. RD cells that were treated with drugs prior to viral infection were washed before infection. Each value is the result of mean ± S.D. of three independent experiments.
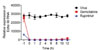
Figure 4
Effects of gemcitabine on the infectivity of E30 particles. E30 particles were incubated with 10 µM gemcitabine or 2 µM rupintrivir for 1h at 4℃. RD cells were then incubated with the treated or untreated virus for 1 h at 37℃. Unbound virus was removed by extensive washing, and incubation was continued with or without 10 µM gemcitabine or 2 µM rupintrivir, at 37℃. Antiviral activity was determined using RT-qPCR, 12 h post-infection. Preinc, pre-incubation of virus with the indicated drug without subsequent drug treatment of the infected cells; Inc., incubation of cells with the indicated drug after viral infection.
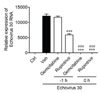
Figure 5
NTP inhibits the antiviral activity of gemcitabine in vitro. E30-infected RD cells were incubated with gemcitabine for 4 8 h at the indicated concentrations and (A) ATP, (B) GTP, (C) CTP, (D) UTP, and cell viability was determined. Data is expres sed as mean ± SD of the cell viability percentage values obtained from three independent experiments carried out in a triplic ate. ***P<0.001 for comparisons to the control, and #P<0.1, ##P<0.01, ###P<0.001 for comparisons between NTP treatment and NTP non-treatment, and at the corresponding dose of gemcitabine.
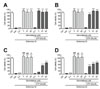
References
1. Fieldhouse JK, Wang X, Mallinson KA, Tsao RW, Gray GC. A systematic review of evidence that enteroviruses may be zoonotic. Emerg Microbes Infect. 2018; 7:164.


2. Harvala H, Calvert J, Van Nguyen D, Clasper L, Gadsby N, Molyneaux P, et al. Comparison of diagnostic clinical samples and environmental sampling for enterovirus and parechovirus surveillance in Scotland, 2010 to 2012. Euro surveill. 2014; 19:20772.


3. Maguire HC, Atkinson P, Sharland M, Bendig J. Enterovirus infections in England and Wales: laboratory surveillance data: 1975 to 1994. Commun Dis Public Health. 1999; 2:122–125.
4. Sharp J, Harrison CJ, Puckett K, Selvaraju SB, Penaranda S, Nix WA, et al. Characteristics of young infants in whom human parechovirus, enterovirus or neither were detected in cerebrospinal fluid during sepsis evaluations. Pediatr Infect Dis J. 2013; 32:213–216.


5. Peigue-Lafeuille H, Croquez N, Laurichesse H, Clavelou P, Aumaître O, Schmidt J, et al. Enterovirus meningitis in adults in 1999-2000 and evaluation of clinical management. J Med Virol. 2002; 67:47–53.


6. Amvrosieva TV, Titov LP, Mulders M, Hovi T, Dyakonova OV, Votyakov VI, et al. Viral water contamination as the cause of aseptic meningitis outbreak in Belarus. Cent Eur J Public Health. 2001; 9:154–157.
7. Drebot MA, Nguan CY, Campbell JJ, Lee SH, Forward KR. Molecular epidemiology of enterovirus outbreaks in Canada during 1991-1992: identification of echovirus 30 and coxsackievirus B1 strains by amplicon sequencing. J Med Virol. 1994; 44:340–347.


8. Lévêque N, Jacques J, Renois F, Antona D, Abely M, Chomel JJ, et al. Phylogenetic analysis of Echovirus 30 isolated during the 2005 outbreak in France reveals existence of multiple lineages and suggests frequent recombination events. J Clin Virol. 2010; 48:137–141.


9. Khetsuriani N, Lamonte-Fowlkes A, Oberst S, Pallansch MA. Enterovirus surveillance--United States, 1970-2005. MMWR Surveill Summ. 2006; 55:1–20.
10. Palacios G, Casas I, Cisterna D, Trallero G, Tenorio A, Freire C. Molecular epidemiology of echovirus 30: temporal circulation and prevalence of single lineages. J Virol. 2002; 76:4940–4949.


11. McWilliam Leitch EC, Bendig J, Cabrerizo M, Cardosa J, Hyypiä T, Ivanova OE, et al. Transmission networks and population turnover of echovirus 30. J Virol. 2009; 83:2109–2118.


12. Metcalf CJE, Lessler J. Opportunities and challenges in modeling emerging infectious diseases. Science. 2017; 357:149–152.


13. De Rycker M, Baragaña B, Duce SL, Gilbert IH. Challenges and recent progress in drug discovery for tropical diseases. Nature. 2018; 559:498–506.


14. Baker S, Thomson N, Weill FX, Holt KE. Genomic insights into the emergence and spread of antimicrobial-resistant bacterial pathogens. Science. 2018; 360:733–738.


15. Meylan S, Andrews IW, Collins JJ. Targeting Antibiotic Tolerance, Pathogen by Pathogen. Cell. 2018; 172:1228–1238.


16. Willing BP, Russell SL, Finlay BB. Shifting the balance: antibiotic effects on host-microbiota mutualism. Nat Rev Microbiol. 2011; 9:233–243.


17. Baig J, Shokouh-Amiri M, Chan J, Chowdhery R, Danthurthy S, Venepalli NK. The Spectrum of Pulmonary Toxicity in Pancreatic Cancer Patients Receiving Gemcitabine Combination Chemotherapy. Case Rep Oncol. 2019; 12:506–512.


18. Song J, Yeo SG, Hong EH, Lee BR, Kim JW, Kim J, et al. Antiviral Activity of Hederasaponin B from Hedera helix against Enterovirus 71 Subgenotypes C3 and C4a. Biomol Ther. 2014; 22:41–46.


19. Choi HJ, Kim JH, Lee CH, Ahn YJ, Song JH, Baek SH, et al. Antiviral activity of quercetin 7-rhamnoside against porcine epidemic diarrhea virus. Antiviral Res. 2009; 81:77–81.


20. Kwon BE, Song JH, Song HH, Kang JW, Hwang SN, Rhee KJ, et al. Antiviral Activity of Oroxylin A against Coxsackievirus B3 Alleviates Virus-Induced Acute Pancreatic Damage in Mice. PloS one. 2016; 11:e0155784.


21. Song JH, Kim SR, Heo EY, Lee JY, Kim DE, Cho S, et al. Antiviral activity of gemcitabine against human rhinovirus in vitro and in vivo. Antiviral Res. 2017; 145:6–13.


22. Heinemann V, Schulz L, Issels RD, Plunkett W. Gemcitabine: a modulator of intracellular nucleotide and deoxynucleotide metabolism. Semin Oncol. 1995; 22:11–18.