Abstract
In this study, the protective effects of EGCG on L-3,4-dihydroxyphenylalanine (L-DOPA)-induced oxidative cell death in catecholaminergic PC12 cells, the in vitro model of Parkinson's disease, were investigated. Treatment with L-DOPA at concentrations higher than 150 µM caused cytotoxicity in PC12 cells, as determined using the 3-(4,5-dimetylthiazol-2-yl)-2,5-diphenyltetrazolium bromide (MTT) assay and flow cytometry detection. The apoptotic ratio was similar in cells treated with 100 µM EGCG plus 150 µM L-DOPA (5.02%) and the control (0.96%) (P > 0.05), and was lower than that of cells treated with L-DOPA only (32.24%, P < 0.05). The generation level of ROS (% of control) in cells treated with EGCG plus L-DOPA was lower than that in cells treated with L-DOPA only (123.90% vs 272.32%, P < 0.05). The optical density in production of TBARS in cells treated with L-DOPA only was higher than that in the control (0.27 ± 0.05 vs 0.08 ± 0.04, P < 0.05), and in cells treated with EGCG only (0.14 ± 0.02, P < 0.05), and EGCG plus L-DOPA (0.13 ± 0.02, P < 0.05). The intracellular level of GSH in cells treated with EGCG plus L-DOPA was higher than that in cells treated with L-DOPA only (233.25 ± 16.44 vs 119.23 ± 10.25, P < 0.05). These results suggest that EGCG protects against L-DOPA-induced oxidative apoptosis in PC12 cells, and might be a potent neuroprotective agent.
Parkinson's disease (PD) is a neurodegenerative disorder characterized by degeneration of dopaminergic neurons in the substantis nigra and dopamine depletion in the striatum [1]. The neuropathology associated with PD within and around the substantia nigra is thought to involve excess production of free radical, L-DOPA autoxidation [2,3], defects in expression of glutathione peroxidase, attenuated levels of reduced GSH [4], altered calcium homeostasis [5], elevation in brain iron content, excitotoxicity, and genetic defects in mitochondrial complex I activity [6]. In addition, enhanced levels of lipid peroxidation products have been reported in brains of PD patients [7]. Oxidative stress to doparminergic neurons is believed to be one of the causes of neurodegeneration in PD [8,9]. Antioxidants, as scavengers of ROS and free radicals, may play an important role in prevention of PD.
The catechins in green tea have attracted considerable attention in recent years for their potential for prevention of oxidative stress-related diseases, including neurodegenerative disease, cardiovascular disease, and cancer [10-13]. One of the most important catechins, epigallocatechin gallate (EGCG, Fig. 1), exerts protective effects, such as antioxidant, anticarcinogenic, antimutagenic, and antineurodegenerative effects [10,12,14-16]. EGCG has been reported to exhibit a potent antioxidant property because it possesses two triphenolic groups in its structure [17]. In addition, EGCG has been proven to be neuroprotective in both cell and animal models of PD [12,18,19]. After exposure of PC12 cells to hydrogen peroxid, EGCG inhibited many points of the apoptotic sequence, including release of caspase-3, and cytochrome c, cleavage of poly (ADP-ribose) polymerase, the glycogen synthase kinase-3 pathway, and modulated cell signaling by activation of the phophatidyl inositol-3 kinase/Akt pathway [20].
L-DOPA induces apoptosis in PC 12 cells and is most commonly used in animal models of PD [21,22]. L-DOPA, is the natural precursor of dopamine in catecholaminergic neurons and the most widely used medicine in treatment of PD, its tocixity in cell lines, including PC12 cells [2,3], cultured sympathetic neurons [23], and striatal neurons [24] has been reported. L-DOPA, through auto- and enzymatic oxidation, can generate a variety of cytotoxic oxygen radical species, including superoxide, hydrogen peroxide, semiquinones, and quinones [2,3,25]. Thus, as the major component of ROS, L-DOPA has been used extensively as an inducer of oxidative stress in in vitro models [2,3]. The sympathetic nerve pheochromocytoma cell line, PC12 cells, is a good model system for the study of oxidative stress in dopamine-containing cells as they exhibit several physiological properties of dopaminergic neurons [26].
Therefore, in this study, the protective effects of EGCG on L-DOPA-induced cytotoxicity in PC12 cells were investigated.
EGCG, RNase A, propidium iodide (PI), ethylenediaminetetraacetic acid (EDTA), 3-(4,5-dimetylthiazol-2-yl)-2,5-diphenyltetrazolium bromide (MTT), and poly-L-lysine were purchased from Sigma (St. Louis, MO, USA). All sera, antibiotics, and RPMI 1640 for cell culture were obtained from Gibco (Grand Island, NY, USA). All other chemicals were of reagent grade.
PC12 cells were routinely maintained in RPMI 1640 medium supplemented with 10% heat-inactivated donor horse serum and 5% heat-inactivated fetal calf serum plus 100 units/mL penicillin and 100 µg/mL streptomycin at 37℃. After 24 h, the cells were exposed to L-DOPA only or pretreated with EGCG for 30 min before treatment with DOPA.
MTT assay was performed as described previously with slight modification [27]. After a period of incubation, the viable cells could convert the soluble dye MTT to insoluble blue formazan crystals. PC12 cells were diluted to 1 × 105 cells/mL and plated at 100 µL per well in a 96-well microplate. PC12 cells were treated with various concentrations of catechin and/or L-DOPA (20-100 µM) for 24 h. MTT solution (final concentration 1 mg/mL) was added to the cells and the cultures were allowed to incubate at 37℃ for 4 h. After the supernatant was discarded by centrifuging, the pellets were dissolved in 100 µL isopropanol containing 0.8 M HCl, and the absorbance was then measured at 570 nm using a Bauty Diagnostic Microplate Reader (Molecular Devices, CA, USA). Cell viability was expressed as a percentage of the control value.
Flow cytometry was performed for determination of cell death. PC12 cells were harvested, washed with ice-cold PBS, fixed in ice-cold 70% ethanol, and stored at -20℃ until analysis. Cells were then washed in PBS and suspended in 1 mL staining solution (50 µg/mL PI, 0.05 mg/mL ribonuclease A and 0.1 mM EDTA, pH 7.4 in PBS), followed by incubation at room temperature for 30 min. Analysis of 10.000 events was performed on a FACScan flow cytometer and calculation of the percentage of apoptotic cells was based on the cumulative frequency curves of the appropriate DNA histograms. Flow cytometric diagram was recorded using a FACS vantage fluorescence-activated flow cytometer (Becton Dickinson, CA, USA).
An assay based on the conversion of non-fluorescent DCFH2-DA to a fluorescent species by intracellular ROS was used in this study. PC12 cells (per 100 µL in 96-well plates) were rinsed with Krebs Ringer buffer and loaded with 50 µM DCFH2-DA for 45 min at 37℃ according to the method of Gunasekar et al. [28], the cells were then washed twice with the same buffer and treated with varying concentrations of L-DOPA alone or pretreated with EGCG for 30 min. After exposure to the compounds, the cells were washed with Krebs Ringer solution and fluorescence intensity was measured at 475 nm excitation and 525 nm emission using a fluorescence spectrophotometer (Kotron AG, Swiss).
Formation of lipid peroxides during the lipid peroxidation process was analyzed by measurement of thiobarbituric acid-reacting substances (TBARS) in PC12 cells, as described previously [29]. Briefly, an equal volume of ice-cold 10% TBA was added to the cell suspension. A solution containing 0.335% of 2-TBA in 50% glacial acetic acid was added and samples were incubated at 100℃ for 30 min. Samples were cooled, and water-saturated butanol was added to the solution. After centrifugation at 2,000 rpm for 5 min, TBARS were determined by absorbance at 535 nm.
The intracellular total GSH content was measured using a commercially available colorimetric assay kit [30]. Cells were gently washed with PBS and resuspended with 10 mM HCl. After freezing and thawing, a solution of 5% sulfosalicylic acid was added at a final concentration of 1% and samples were centrifuged at 15,000 rpm for 10 min. Supernatants were incubated with a reaction mixture containing 5,5'-dithiobis (2-nitrobezoic acid), NADPH, and glutathione reductase in a 96-well plate. The absorbance was measured at 405 nm using a microplate reader (Molecular Devices, CA, USA).
All data were expressed as mean ± SEM of at least six experiments. Statistical analyses were performed using InStat Ver 3.06 (GraphPad Software, Inc.,). Statistical significance of the data was determined using ANOVA, followed by Tukey's, test, and values ≤ 0.05 were considered significant.
Treatment of PC12 cells with 50, 100, 150, and 200 µM of EGCG for 24 h resulted in no significant reduction of cell viability (Fig. 2). L-DOPA-induced cytotoxicity was also determined by the percentage of MTT reduction assay after culture with increasing concentrations (50-150 µM) of L-DOPA for up to 24 h. Treatment with L-DOPA resulted in a concentration-dependent decrease in cell viability, whereas pretreatment with EGCG at 50 and 100 µM for 30 min prevented the loss of viability (Fig. 3). Cell viability of PC12 cells treated with 150 µM L-DOPA for 24 h was apprpximately 62.49 ± 4.21% of untreated control value, whereas the cell viability of cells pretreated with 100 µM EGCG for 30 min before exposure to L-DOPA was up to 101.21 ± 5.63%. Results of the MTT assay indicated that treatment with L-DOPA resulted in reduced viability of PC12 cells, which was prevented by pretreatment with EGCG.
Apoptotic cells were quantified in cells pretreated with EGCG prior to delivery of L-DOPA using flow cytometric detection. When a number of apoptotic cells were stained with a DNA-specific fluorochrome, such as propidium iodide, a special DNA peak (usually called subG1 peak or apoptotic peak) appeared. This peak is thought to be one of the characteristics of apoptosis. Treatment with EGCG (100 µM) and L-DOPA (150 µM) together for 24 h resulted in a marked decrease the percentage of apoptotic cells, compared with cells treated with L-DOPA alone (Fig. 4). The ratios of apoptotic cells in the subG1 phase were 0.96 ± 0.22%, 2.34 ± 0.64%, and 32.24 ± 4.21% in the control, EGCG, and L-DOPA treated cells, respectively. However, the apoptotic ratio was reduced by 5.02 ± 1.17% in cells pretreated with 100 µM EGCG before exposure to L-DOPA. The protective effect of EGCG on L-DOPA-induced cytotoxicity as determined by flow cytometry was similar to that determined by MTT assay.
To investigate involvement of oxygen radicals in L-DOPA-induced apoptosis of PC12 cells, accumulation of ROS after exposure to L-DOPA was measured utilizing a conversion of DCF-DA to DCF. An increase (2-fold) in ROS production was observed after treatment of cells with L-DOPA, which was showed a significant decrease (1.5-fold) after pretreatment with EGCG (P < 0.05) (Fig. 5). EGCG were inhibitory in the presence of L-DOPA, indicating the ROS scavenging activity of EGCG under oxidative stress conditions.
The resultant oxidative stress was also determined by measuring the level of lipid peroxidation. To estimate the preventive effect of EGCG, PC12 cells were treated with L-DOPA for 3 h, and the level of lipid peroxide was measured by TBARS assay (Fig. 6). Production of TBARS in PC12 cells treated with L-DOPA (150 µM) showed a significant increase, to 301 ± 10% (P < 0.05 over control). Pretreatment with EGCG (100 µM) inhibited production of TBARS by 102 ± 9% compared to L-DOPA alone.
Because the data from MTT, ROS, and TBARS suggested protection against oxidative stress, total GSH was investigated in cells pretreated with EGCG. PC12 cells treated with 150 µM L-DOPA for 24 h showed a significant decrease in the intracellular level of GSH relative to the level observed in control cells (2-fold, P < 0.05) (Fig. 7). However, pretreatment with EGCG at 100 µM before exposure to L-DOPA resulted in a marked increase in the level of GSH compared to that of L-DOPA alone in PC12 cells (1.7-fold, P < 0.05).
Oxidative stress is believed to play important role in a number of neurodegenerative disorders, including PD, stroke, and Alzheimer's disease. Neurodegenerative disorders are a class of diseases linked to elevation of ROS and apoptosis [31]. Intracellular accumulation of ROS will quickly exhaust reducing agents such as GSH. Many cellular compounds are easily oxidized, and lipid peroxides have been used as a marker for oxidative stress [32,33]. Finding of the current study demonstrated that exposure to L-DOPA-induced oxidative stress, characterized by a number of potentially detrimental changes in intracellular ROS, lipids, and GSH in PC12 cells. In addition, ROS cause DNA damage by directly attacking DNA, ultimately contributing to cell death [4]. We demonstrated that exposure of PC12 cells to L-DOPA resulted in a significant increase in ROS, triggered DNA damage, as examined by use of a DNA sensitive dye with flow cytometry, showed a significant subG1 peak in the histograms, and produced neurotoxicity as evaluated by MTT assay.
Food-derived antioxidants such as phytochemicals and vitamins have recently received increasing attention, because they are known to function as chemoprotective and chemopreventive agents against oxidative stress [9]. EGCG, the major polyphenol isolated from green tea, was shown in several studies to possess neuroprotective effects against a variety of toxic insults and neuronal injuries [12,18,19]. Thus, in order to investigate the mechanism underlying EGCG induced protection against L-DOPA associated oxidative damage in PC12 cells, we measured generations of ROS, GSH content, and lipid peroxidation. One of the possible protective mechanisms of EGCG might be its direct scavenging of ROS produced by L-DOPA. In the current study, an increase in intracellular levels of ROS was observed in L-DOPA-treated PC12 cells, which were decreased by EGCG, suggesting that a radical scavenging effect of EGCG may be a significant contributor to the neuroprotective effect. In relation to this, Levites et al. [34-36], reported that EGCG protected neuronal cells against the cytotoxic effect of 6-hydroxydopamine (OHDA), N-methyl-4-phenyl-1,2,3,6-tetrahydropyridine, and amyloid β peptide.
Increase of free radicals also leads to production of lipid peroxidation, a major cause of cell membrane damage, which eventually leads to degeneration of cells. Dietary polyphenols, such as catechins, are known to protect against oxidation of biomolecules by oxidant species, and by prevention of lipid peroxidation [15,16]. In this study, using EGCG, we observed marked inhibition of L-DOPA-induced lipid peroxidation in PC12 cells. EGCG has also been shown to protect against lipid peroxidation by initiators of oxidative stress, such as 6-OHDA [12], hydrogen peroxide [20], and 3-hydroxykynurenine [37]. Previous study has shown that pretreatment with EGCG results in attenuation of the increase in the Bax to Bcl-2 ratio, a marker of apoptosis, which is increased by oxidative stress in PC12 cells [38]. In addition, in the central nervous system, oral administration of green tea polyphenols or flavonoid-related compounds has preventive effects on accumulation of iron-salt-induced lipid peroxide in the brain [19], age-related accumulation of neurotoxic lipid peroxides in rat brain, and ischemia-reperfusion-induced neural death in Mongolian gerbils [39].
GSH is a critical intracellular antioxidant, and its radicalscavenging ability is the primary mechanism for elimination of excess ROS under physiological conditions [31]. There is evidence that depletion of cellular GSH in cerebellar granule neurons and PC12 cells results in increased generation of ROS, disruption of the mitochondrial transmembrane potential, and rapid loss of mitochondrial function [40,41]. The toxic effect of L-DOPA on neuronal cells appears to be mediated by formation and reduction of intracellular GSH contents [4]. Results of this study indicate that depletion of GSH may play an important role in the cytotoxic effect of L-DOPA. In this study, pretreatment of PC12 cells with EGCG prevented the marked decline of intracellular GSH levels that normally occurs after exposure to L-DOPA. The result again suggests an oxidative stress-dependent mechanism and further demonstrates regulation of an endogenous antioxidant mechanism by EGCG. Raza and John [42] also reported that alteration in GSH metabolism can be achieved through administration of polyphenols, particularly EGCG.
One possible mechanism underlying the effectiveness of EGCG against oxidative stress induced by L-DOPA involved its polyphenolic structure, since plant-derived polyphenolic compounds are known to be potent antioxidant and free radical scavengers [43]. Thus, catechins have been studied with regard to their structure-antioxidant activity relationship [16]. The structure-antioxidant activity relationship of EGCG (Fig. 1) has been demonstrated in many studies, where the active site of green tea polyphenols reacted directly with oxygen free radicals at the ortho-hydroxyl group in the B ring and galloyl moitery in the C ring in the chemical structure of EGCG [16]. In addition, the galloyl group was very important for the antioxidant properties of EGCG against lipid peroxidation in synaptosomes [15,16]. Oral administration of EGCG alone also resulted in elevated activity of superoxide dismutase and catalase in striatum [34]. Since L-DOPA treatment has been shown to increase GSSG levels with depletion of reduced GSH [4], critical involvement of the galloyl group in L-DOPA cytotoxicity is possible. In addition, using in vitro and in vivo models, recent studies have suggested the protective effects of tea catechins on neuronal damage induced by free radical attack [12,15,19].
In summary, L-DOPA-induced apoptosis in PC12 cells through induction of oxidative stress, which could be attenuated by treatment with EGCG. EGCG may decrease the L-DOPA-induced death of PC12 cells through a scavenging action on ROS, inhibition of lipid peroxidation, and increase in GSH level. Therefore, the current data indicate that EGCG might be a potent neuroprotective agent against diseases such as PD. However, conduct of more epidemiological and clinical studies needed in order to fully elucidate their efficacy.
Figures and Tables
Fig. 2
Effects of EGCG on viability of PC12 cells. PC12 cells were incubated for 24 h in media containing various concentrations of EGCG (50-200 µM). Each value was calculated as a percentage of the MTT assay in control PC12 cells. The results represent the mean ± SEM of six experiments.
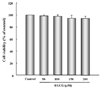
Fig. 3
Effects of EGCG on L-DOPA-induced decrease in viability of PC12 cells. After 24 h in culture, PC12 cells were exposed to 50-150 µM L-DOPA or preincubated with 50-100 µM EGCG for 30 min before treatment with L-DOPA. Cell viability was assessed using the MTT method and the results represent the mean ± SEM of six experiments. *P < 0.05 compared with the untreated control group; #P < 0.05 compared with L-DOPA damaged cell groups (ANOVA followed by Tukey's test).
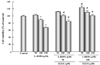
Fig. 4
Flow cytometric histograms of PC 12 cells after exposure to 150 µM L-DOPA alone for 24 h or preincubated with 100 µM EGCG for 30 min before treatment with L-DOPA. (A) After incubation, cells were harvested and stained with propidium iodide. Relative DNA content was analyzed by flow cytometry. The X-axis represents DNA content and the Y-axis represents the number of cells. (B) The results shown in present the mean ± SEM of six experiments. *P < 0.05 compared with the untreated control group; #P < 0.05 compared with L-DOPA damaged cell groups (ANOVA followed by Tukey's test).
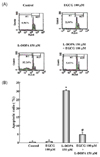
Fig. 5
Effect of EGCG on L-DOPA-induced intracellular accumulation of ROS in PC12 cells loaded with DCFH2-DA. PC12 cells in culture after 1 h were exposed to 150 µM L-DOPA or preincubated with 100 µM EGCG for 30 min before treatment with L-DOPA. Formation of peroxides, detected using 2',7'-dichloroluorescin oxidation and fluorescence, was monitored on a Multi-Well Plate Reader. The results were expressed as a relative percentage of DCF fluorescence. Values are expressed as the mean ± SEM of triplicate determinations in six distinct experiments. *P < 0.05 compared with the untreated control group; #P < 0.05 compared with L-DOPA damaged cell groups (ANOVA followed by Tukey's test).
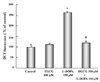
Fig. 6
Effect of EGCG on L-DOPA-induced TBARS formation in PC12 cells. PC12 cells in culture were exposed to 150 µM L-DOPA or preincubated with 100 µM EGCG for 30 min before treatment with L-DOPA. After exposure for 24 h, the increase in TBARS formation was evaluated. The results represent the mean ± SEM of six experiments. *P < 0.05 compared with the untreated control group; #P < 0.05 compared with L-DOPA damaged cell groups (ANOVA followed by Tukey's test).
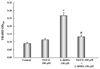
Fig. 7
Decrease in intracellular GSH content by L-DOPA and its inhibition by EGCG. PC12 cells in culture for 24 h were exposed to 150 µM L-DOPA or preincubated with 100 µM EGCG for 30 min before treatment with L-DOPA. After incubation, the total intracellular GSH content was measured using DTNB, as described in the Materials and Methods section. The results represent the mean ± SEM of six experiments. *P < 0.05 compared with the untreated control group; #P < 0.05 compared with L-DOPA damaged cell groups (ANOVA followed by Tukey's test).
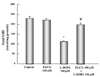
References
2. Lee JJ, Kim YM, Yin SY, Park HD, Kang MH, Hong JT, Lee MK. Aggravation of L-DOPA-induced neurotoxicity by tetrahydropapaveroline in PC12 cells. Biochem Pharmacol. 2003; 66:1787–1795.


3. Migheli R, Godani C, Sciola L, Delogu MR, Serra PA, Zangani D, De Natale G, Miele E, Desole MS. Enhancing effect of manganese on L-DOPA-induced apoptosis in PC12 cells: role of oxidative stress. J Neurochem. 1999; 73:1155–1163.
4. Spencer JP, Jenner P, Halliwell B. Superoxide-dependent depletion of reduced glutathione by L-DOPA and dopamine. Relevance to Parkinson's disease. Neuroreport. 1995; 6:1480–1484.


5. Hirsch EC. Biochemistry of Parkinson's disease with special reference to the dopaminergic systems. Mol Neurobiol. 1994; 9:135–142.


6. Mizuno Y, Ikebe S, Hattori N, Nakagawa-Hattori Y, Mochizuki H, Tanaka M, Ozawa T. Role of mitochondria in the etiology and pathogenesis of Parkinson's disease. Biochim Biophys Acta. 1995; 1271:265–274.


7. Dexter DT, Carter CJ, Wells FR, Javoy-Agid F, Agid Y, Lees A, Jenner P, Marsden CD. Basal lipid peroxidation in substantia nigra is increased in Parkinson's disease. J Neurochem. 1989; 52:381–389.


8. Chung KK, Dawson VL, Dawson TM. New insights into Parkinson's disease. J Neurol. 2003; 250:Suppl 3. III15–III24.


9. Heo HJ, Choi SJ, Choi SG, Shin DH, Lee JM, Lee CY. Effects of banana, orange, and apple on oxidative stress-induced neurotoxicity in PC12 cells. J Food Sci. 2008; 73:H28–H32.


10. Bastianetto S, Zheng WH, Quirion R. The Ginkgo biloba extract (EGb 761) protects and rescues hippocampal cells against nitric oxide-induced toxicity: involvement of its flavonoid constituents and protein kinase C. J Neurochem. 2000; 74:2268–2277.


11. Bordoni A, Hrelia S, Angeloni C, Giordano E, Guarnieri C, Caldarera CM, Biagi PL. Green tea protection of hypoxia/reoxygenation injury in cultured cardiac cells. J Nutr Biochem. 2002; 13:103–111.


12. Nie G, Jin C, Cao Y, Shen S, Zhao B. Distinct effects of tea catechins on 6-hydroxydopamine-induced apoptosis in PC12 cells. Arch Biochem Biophys. 2002; 397:84–90.


13. Song DU, Jung YD, Chay KO, Chung MA, Lee KH, Yang SY, Shin BA, Ahn BW. Effect of drinking green tea on age-associated accumulation of Maillard-type fluorescence and carbonyl groups in rat aortic and skin collagen. Arch Biochem Biophys. 2002; 397:424–429.


14. Dong Z, Ma W, Huang C, Yang CS. Inhibition of tumor promoter-induced activator protein 1 activation and cell transformation by tea polyphenols, (-)-epigallocatechin gallate, and theaflavins. Cancer Res. 1997; 57:4414–4419.
15. Guo Q, Zhao B, Li M, Shen S, Xin W. Studies on protective mechanisms of four components of green tea polyphenols against lipid peroxidation in synaptosomes. Biochim Biophys Acta. 1996; 1304:210–222.


16. Guo Q, Zhao B, Shen S, Hou J, Hu J, Xin W. ESR study on the structure-antioxidant activity relationship of tea catechins and their epimers. Biochim Biophys Acta. 1999; 1427:13–23.


17. Jin CF, Shen SR Sr, Zhao BL. Different effects of five catechins on 6-hydroxydopamine-induced apoptosis in PC12 cells. J Agric Food Chem. 2001; 49:6033–6038.


18. Levites Y, Youdim MB, Maor G, Mandel S. Attenuation of 6-hydroxydopamine (6-OHDA)-induced nuclear factor-kappaB (NF-kappaB) activation and cell death by tea extracts in neuronal cultures. Biochem Pharmacol. 2002; 63:21–29.


19. Yoneda T, Hiramatsu M, Sakamoto M, Togasaki K, Komatsu M, Yamaguchi K. Antioxidant effects of "beta catechin". Biochem Mol Biol Int. 1995; 35:995–1008.
20. Koh SH, Kim SH, Kwon H, Park Y, Kim KS, Song CW, Kim J, Kim MH, Yu HJ, Henkel JS, Jung HK. Epigallocatechin gallate protects nerve growth factor differentiated PC12 cells from oxidative-radical-stress-induced apoptosis through its effect on phosphoinositide 3-kinase/Akt and glycogen synthase kinase-3. Brain Res Mol Brain Res. 2003; 118:72–81.


21. Casarejos MJ, Solano RM, Menéndez J, Rodríguez-Navarro JA, Correa C, García de Yébenes J, Mena MA. Differential effects of L-DOPA on monoamine metabolism, cell survival and glutathione production in midbrain neuronal-enriched cultures from parkin knockout and wild-type mice. J Neurochem. 2005; 94:1005–1014.


22. Walkinshaw G, Waters CM. Induction of apoptosis in catecholaminergic PC12 cells by L-DOPA. Implications for the treatment of Parkinson's disease. J Clin Invest. 1995; 95:2458–2464.


23. Boyce S, Rupniak NM, Steventon MJ, Iversen SD. Nigrostriatal damage is required for induction of dyskinesias by L-DOPA in squirrel monkeys. Clin Neuropharmacol. 1990; 13:448–458.


24. Cheng N, Maeda T, Kume T, Kaneko S, Kochiyama H, Akaike A, Goshima Y, Misu Y. Differential neurotoxicity induced by L-DOPA and dopamine in cultured striatal neurons. Brain Res. 1996; 743:278–283.


25. Basma AN, Morris EJ, Nicklas WJ, Geller HM. L-DOPA cytotoxicity to PC12 cells in culture is via its autoxidation. J Neurochem. 1995; 64:825–832.


26. Greene LA, Tischler AS. Establishment of a noradrenergic clonal line of rat adrenal pheochromocytoma cells which respond to nerve growth factor. Proc Natl Acad Sci U S A. 1976; 73:2424–2428.


27. Mosmann T. Rapid colorimetric assay for cellular growth and survival: application to proliferation and cytotoxicity assays. J Immunol Methods. 1983; 65:55–63.


28. Gunasekar PG, Kanthasamy AG, Borowitz JL, Isom GE. NMDA receptor activation produces concurrent generation of nitric oxide and reactive oxygen species: implication for cell death. J Neurochem. 1995; 65:2016–2021.


29. Draper HH, Hadley M. Malondialdehyde determination as index of lipid peroxidation. Methods Enzymol. 1990; 186:421–431.
30. Griffith OW. Determination of glutathione and glutathione disulfide using glutathione reductase and 2-vinylpyridine. Anal Biochem. 1980; 106:207–212.


31. Raza H, John A. In vitro effects of tea polyphenols on redox metabolism, oxidative stress, and apoptosis in PC12 cells. Ann N Y Acad Sci. 2008; 1138:358–365.


32. Watanabe H, Kobayashi A, Yamamoto T, Suzuki S, Hayashi H, Yamazaki N. Alterations of human erythrocyte membrane fluidity by oxygen-derived free radicals and calcium. Free Radic Biol Med. 1990; 8:507–514.


33. Zeevalk GD, Bernard LP, Albers DS, Mirochnitchenko O, Nicklas WJ, Sonsalla PK. Energy stress-induced dopamine loss in glutathione peroxidase-overexpressing transgenic mice and in glutathione-depleted mesencephalic cultures. J Neurochem. 1997; 68:426–429.


34. Levites Y, Weinreb O, Maor G, Youdim MB, Mandel S. Green tea polyphenol (-)-epigallocatechin-3-gallate prevents N-methyl-4-phenyl-1,2,3,6-tetrahydropyridine-induced dopaminergic neurodegeneration. J Neurochem. 2001; 78:1073–1082.


35. Levites Y, Youdim MB, Maor G, Mandel S. Attenuation of 6-hydroxydopamine (6-OHDA)-induced nuclear factor-kappaB (NF-kappaB) activation and cell death by tea extracts in neuronal cultures. Biochem Pharmacol. 2002; 63:21–29.


36. Levites Y, Amit T, Mandel S, Youdim MB. Neuroprotection and neurorescue against Abeta toxicity and PKC-dependent release of nonamyloidogenic soluble precursor protein by green tea polyphenol (-)-epigallocatechin-3-gallate. FASEB J. 2003; 17:952–954.
37. Jeong JH, Kim HJ, Lee TJ, Kim MK, Park ES, Choi BS. Epigallocatechin 3-gallate attenuates neuronal damage induced by 3-hydroxykynurenine. Toxicology. 2004; 195:53–60.


38. Jung JY, Mo HC, Yang KH, Jeong YJ, Yoo HG, Choi NK, Oh WM, Oh HK, Kim SH, Lee JH, Kim HJ, Kim WJ. Inhibition by epigallocatechin gallate of CoCl2-induced apoptosis in rat PC12 cells. Life Sci. 2007; 80:1355–1363.


39. Inanami O, Watanabe Y, Syuto B, Nakano M, Tsuji M, Kuwabara M. Oral administration of (-)catechin protects against ischemia-reperfusion-induced neuronal death in the gerbil. Free Radic Res. 1998; 29:359–365.


40. Seyfried J, Soldner F, Schulz JB, Klockgether T, Kovar KA, Wullner U. Differential effects of L-buthionine sulfoximine and ethacrynic acid on glutathione levels and mitochondrial function in PC12 cells. Neurosci Lett. 1999; 264:1–4.


41. Wüllner U, Löschmann PA, Schulz JB, Schmid A, Dringen R, Eblen F, Turski L, Klockgether T. Glutathione depletion potentiates MPTP and MPP+ toxicity in nigral dopaminergic neurones. Neuroreport. 1996; 7:921–923.

