Abstract
Purpose
To evaluate whether alpha-lipoic acid (ALA) is effective at restoring the levels of nitric oxide synthase (NOS) expression and preventing ultrastructural changes in the bladder of rats with streptozotocin-induced diabetes.
Materials and Methods
Nine-week-old male Sprague-Dawley rats were used. The experimental groups included a control group (n=6), a diabetes group (n=6), and two groups of diabetic rats treated with intraperitoneal injections of ALA (n=12) at either 50 (ALA50) or 100mg/kg/day (ALA100) for 8 weeks after the induction of diabetes. Diabetic oxidative stress was determined based on evaluation of immunohistochemical staining for 8-hydroxy-2-deoxyguanosine (8-OHdG). The measurements of the levels of eNOS and nNOS expressions, as well as an assessment of the ultrastructural changes in detrusor smooth muscle cells were performed.
Results
The highest expression of 8-OHdG was observed in the diabetes group; whereas, the 8-OHdG expression in the ALA-treated groups was similar to that in the control group. Both eNOS and nNOS were constitutively expressed in the control group. The expression levels of both eNOS and nNOS proteins were higher in the diabetes group, which had experienced increased oxidative stress, than in the ALA50 and ALA100 groups. Compared with the control group, the diabetes group exhibited severe degeneration of the detrusor muscle cells. In the rats treated with ALA, the detrusor muscle cells showed mild to moderate degeneration. The mean numbers of mitochondria per smooth muscle cell in the control, diabetes, ALA50 and ALA100 groups were 12.6±1.5, 5.1±0.7, 18.3±0.7 and 19.3±1.3, respectively (p<0.01).
Conclusions
Our data suggest that diabetes enhanced the levels of eNOS and nNOS expressions in the bladder, and ALA inhibited the expressions of eNOS and nNOS. ALA had a protective effect against the degeneration of intracellular micro-organelles produced by diabetic oxidative damage in detrusor muscle cells. This study suggests that early treatment with ALA can reduce the damage caused by diabetic oxidative stress.
Lifestyle changes and advancements in medicine have greatly improved the health and longevity those with diabetes. A Korean study reported that 9.7% of the Korean population had diabetes in 2000.1 Diabetes and urologic complications, such as bladder dysfunction, sexual and erectile dysfunction, and urinary tract infections, are common health problems that markedly increase in prevalence and incidence with advancing age. Although greater than 50% of patients with diabetes may have bladder dysfunction,2 the cause of bladder dysfunction in diabetic patients is not clear.
One of the most important consequences of glucose toxicity in tissues susceptible to diabetic complications is enhanced oxidative stress-induced production of reactive oxygen species (ROS) combined with a down-regulation or insufficient up-regulation of antioxidant defense mechanisms.3 An imbalance between the production and scavenging of free radicals is primarily responsible for the cell and tissue damage associated with diabetes mellitus.
Alpha-lipoic acid (ALA), a disulfide compound found naturally in mitochondria, is the coenzyme for pyruvate dehydrogenase and a-ketoglutarate dehydrogenase. ALA has recently gained attention as a potent antioxidant, and its reduced form, dihydrolipoic acid (DHLA), reacts with ROS such as superoxide radicals, hydroxyl radicals, hypochlorous acid, peroxyl radicals, and singlet oxygen.4 Prolonged ALA supplementation of streptozotocin (STZ)-induced diabetic rats was associated with an attenuation of hyperglycemia, and ALA has been used as a therapy for diabetic neuropathy.5,6
The present study further examined the antioxidative effects of ALA and determined its effects on rats with 8 weeks of uncontrolled type I diabetes. The marker of oxidative stress was 8-hydroxy-2'-deoxyguanosine (8-OHdG) immunoreactivity. Nitric oxide synthase (NOS) expression levels, and ultrastructural changes in bladder cells were examed.
All animal procedures were approved by the Inje University Ethics Committee for Animal Experiments. The study was performed using male Sprague-Dawley rats weighing 200-230g (Orient Co., Korea). Diabetes was induced by a single injection of STZ (Sigma Co., USA) at a dose of 60mg/kg body weight. Blood samples for glucose measurements were taken from the tail vein.
Rats with blood glucose=300mg/dl were considered to be diabetic in 72h after STZ injection. The experimental groups included a control group (n=6, control), a diabetes group (n=6, diabetes), and two groups of diabetic rats treated with intraperitoneal injection of ALA (Thioctacid injection, Bukwang Pharmaceutical Co., Korea) at either 50 (n=6, ALA50) or 100 mg/kg/day (n=6, ALA100) for 8 weeks after the induction of diabetes.
The rats were sedated with ether and killed by cervical dislocation. After dissection of the bladder from the pelvic cavity, the bladder tissue was fixed overnight in 4% paraformaldehyde, washed in phosphate-buffered saline (PBS), and frozen in liquid nitrogen.
Frozen bladder tissue samples were homogenized in lysis buffer (50mM Tris-HCl, 150mM NaCl, 5mM EDTA, 1% NP-40, 1g/ml aprotinin). The lysate was incubated on ice for 10 min and centrifuged at 12,000rpm for 10 min at 4℃. The supernatant was collected, and the protein concentration was determined using Bradford reagent (Bio-Rad Laboratories, Hercules, USA). Lysate samples (50ug protein) were mixed with 5 SDS gel loading buffer and boiled for 5 min at 100℃. The proteins were separated by SDS-PAGE and transferred to a polyvinylidene-difluoride (PVDF) membrane. The membranes were blocked with 5% nonfat milk in Tris-buffered saline containing 0.1% Tween-20 (TBST) for 1 h at room temperature. The membranes were washed three times in TBST for 10 min each and then incubated overnight at 4℃ with rabbit polyclonal anti-NOS1,3 (SC-8309, 8311, Santa Cruz Biotechnology, USA) diluted 1:1,000 in TBST. The membranes were washed three times in TBST for 10 min each and then incubated with horseradish peroxidase (HRP)-conjugated secondary antibody (1:500) in 5% nonfat milk in TBST at room temperature for 1 h. After the membranes were washed three times in TBST for 10 min, the target protein was visualized using an electrochemiluminescence system (Amersham International PLC, USA), and the protein band intensity was quantified by densitometry.
Tissue specimens were embedded in optimal cutting temperature (OCT) compound and snap-frozen in pre-cooled (-70℃) isopentane. Five micrometer slices were taken from the frozen blocks and applied to histology slides for immunohistochemistry. The frozen sections were fixed in cold 4% PFA (4℃) for 10 min. Each section was then immersed in a primary antibody solution containing anti-8-OHdG monoclonal antibody (Trevigen, USA) diluted 1:200 and incubated overnight at 4℃. The sections were washed three times in TBST for 10 min; reacted with biotinylated rabbit anti-goat IgG (PK-6102, Vector Laboratories, USA) as a secondary antibody; washed three times in TBST for 10 min; treated with avidin-biotin complex (ABC) (Vector Laboratories, USA) for 30 min, and stained with DAB substrate. The sections were counterstained with hematoxylin, and the slides were dehydrated and mounted with Permount. The slides were examined by light microscopy (Olympus model CX31-P polarizing microscope, Olympus, Japan), and images were captured using an Optronics video system (Olympus, Japan) attached to the microscope.
The positive staining was quantitatively evaluated by manually counting the cells at a magnification of 400 using a 10×10 grid in the eyepiece. At least 400 cells in four different fields were counted. The mean percent immunoreactivity was determined from the number of positively stained cells divided by the total number of cells.7
The bladder was cut into small pieces (approximately 1mm3), and the pieces were fixed in 2.5% glutaraldehyde, post-fixed in 1% osmium tetroxide in 0.1M phosphate buffer, dehydrated in a graded series of ethanol, and embedded in polar Epon mix. Serial semi-thin sections were cut to approximately 0.5 micrometer, stained with 0.5% toluidine blue, and observed under light microscopy. Ultrathin sections were cut, stained with uranyl acetate and lead citrate, and examined using a JEM-1200EXII transmission electron microscope (JEOL Ltd., Japan) operated at 100kv, 61A. The examination, photography, and initial diagnosis of all biopsy specimens were performed by the primary examiner. The ultrastructure of muscle cells, especially those with degenerative changes, was examined, documented photographically, and compared by counting the number of mitochondria in five muscle cells per slide.
Table 1 shows the initial and final mean body weights and blood glucose levels in the four groups. The blood glucose concentration was higher in the diabetic rats than in the control rats and was affected dose-dependently by ALA treatment. The final body weight was significantly lower in the diabetic and ALA-treated rats than in the rats. The final body weight was lower in the ALA-treated groups than in the untreated diabetes group, but the difference was not significant.
The quantitative evaluation of 8-OHdG expression based on immunoreactivity is shown in Fig. 1. Immunoreactivity of 8-OHdG was observed in 36.2±4.1, 73.7±4.1, 50.2±4.8, and 35.0±6.4% of bladder cells in the control, diabetes, ALA50, and ALA100 groups, respectively. The immunoreactivity pattern and distribution of 8-OHdG expression in bladder tissue sections is shown in Fig. 2. The 8-OHdG-positive cells were localized in the urothelium and smooth muscle cells.
As shown in Fig. 3, eNOS (Fig. 3A, B) and nNOS (Fig. 3A, C) were constitutively expressed in all the bladder tissues. The expression of both eNOS and nNOS was higher in the diabetes group than in the control, ALA50, and ALA100 groups (p<0.05). The expression of nNOS was decreased significantly and dose-dependently by ALA treatment (p<0.05).
As shown in Fig. 4, in the smooth muscle cells of the control rats, the nucleus was capped on one side by smooth endoplasmic reticulum and mitochondria, the sarcoplasm was packed with myofilaments and had evenly distributed dense bodies and scattered mitochondria. In contrast, the detrusor muscle cells of the diabetic rats showed severe degeneration, including disruption of the dense bodies, vacuolation of the sarcoplasm, irregular clumping of chromatin, and loss of electron-dense bands. In the diabetic rats treated with ALA, the detrusor muscle cells showed mild to moderate degeneration, exhibiting misaligned, irregularly scattered dense bodies, and the appearance of highly electron-dense patches in the sarcoplasm. The mean numbers of mitochondria per smooth muscle cell in the control, diabetes, ALA50, and ALA100 groups were 12.6±1.5, 5.1±0.7, 18.3±0.7, and 19.3±1.3, respectively (p<0.01).
In this study, prolonged supplementation of STZ-diabetic rats with ALA affected hyperglycemia, NOS expression, and bladder smooth muscle cell ultrastructure.
Although ALA treatment reduced hyperglycemia, it had no effect on body weight. Variable results have been reported for the effect of ALA on hyperglycemia. Some studies have demonstrated a hyperglycemic-reducing effect of ALA in STZ-diabetic rats, others have shown no reduction in hyperglycemia.8,9 In a study by Maritim, ALA was used at only 10 or 50mg/kg/day for 14 days. A dosage of 600mg/day in human is roughly 10mg/kg body weight, that is similar to the dose used in rats. Many studies have assessed different doses of ALA, ranging from 10-100mg/kg body weight for their experiments. Therefore, two dosage of ALA (50 and 100mg/kg body weight) were chosen for our experiments.8-10
Our study showed a reduction in hyperglycemia, and we suggest that this effect may be both dose and time dependent. ALA was shown to lower elevated glucose levels in animal models. ALA dose not protect pancreatic β-cells, affect insulin secretion. ALA increases glucose uptake in skeletal muscle and adipocytes, which was associated with a significant enhancement of glycogen synthesis or inhibits of gluconeogenesis from alanine and pyruvate.11,12
One consequence of diabetes that is associated with glucose toxicity is mediated through the oxidative stress that results from increased production of ROS. In living cells, ROS are continuously formed as a consequence of metabolic reactions. Under normal physiological conditions, endogenous oxidants and antioxidants are balanced. Excessive generation of oxidants or decreased production of antioxidants can produce an imbalance and can result in oxidative stress.
In the past, indicators of lipid peroxidation, such as malondialdehyde, oxidized LDL, and MDA-modified LDL, have been used as markers of oxidative stress. In recent years, 8-OHdG has emerged as a marker of oxidative stress in diabetes.13,14 The strong immunoreactivity of 8-OHdG in the urothelium and smooth muscle cells of diabetic rats in the present study demonstrated that diabetes-induced oxidative stress had developed in these cells. The immunoreactivity of 8-OHdG was decreased significantly in the ALA-treated groups compared with that in the diabetes group, suggesting that ALA can neutralize or restore diabetes-induced oxidative damage.
Nitric oxide (NO) has gained recognition as an important neurotransmitter and cell mediator in the lower urinary tract. NO is synthesized by nitric oxide synthase (NOS). Hyperglycemia increase eNOS protein expression.15
Our results showed an increase of eNOS protein expression in the bladder tissue of diabetic rats and a decrease in the expression of eNOS after ALA supplementation. In addition to its direct scavenging activity, ALA may modulate endogenous NO bioactivity owing to its known ability to increase intracellular levels of glutathione.16 By improving the intracellular redox state, ALA may protect tetrahydrobiopterin, an essential cofactor for eNOS from oxidation, thereby enhancing the capacity for NO production in endothelial cells.17 Expressions of eNOS may be tissue specific. Increase of eNOS expression is observed in heart and aorta, meanwhile eNOS expression decrease in corpus cavernosum.18-20
It is well known that autonomic neuropathy occurs as a complications of diabetes including bladder dysfunction, impotence. nNOS is detected in bladder and may be tissue-specific.21 Our results showed an increase in nNOS protein expression in the bladder tissue of diabetic rats and a decrease in the expression of nNOS after ALA supplementation. nNOS expression in duodenum longitudinal muscle and penis decreased compared to male control and, in retina nNOS expression was higher than control.22-24 Some investigator has reported ALA treatment restores nNOS expression similar to control in ileum and penis.23
With regard to smooth muscle change, we evaluated the ultrastructure of the detrusor muscle. The features characterizing disruptive degeneration were based on a study by Elbadawi.25 The ultrastructural hallmark of smooth muscle change is a degeneration pattern, which is divided according to the severity and extent of degeneration. Here, the features of the detrusor muscle cells of the ALA-treated rats reflected mild to moderate degeneration, and the features of the cells of diabetic rats indicated severe degeneration. There was also a difference in the number of mitochondria between the diabetic and ALA-treated rats.
In cultured bovine aortic endothelial cells, hyperglycemia resulted in an overproduction of superoxide anion in mitochondria.26 Suicide of the mitochondria (mitoptosis) is initiated by ROS, and sometimes the disappearance of mitochondria is observed prior to cell death.27 ALA supplementation enhances the activities of mitochondrial enzymes and the antioxidant status in cells, thereby protecting the function of the mitochondria.10
ALA provides protection against the progression of bladder dysfunction in streptozotocin-induced diabetes. This study demonstrates the presence of oxidative stress in the bladder tissue of diabetic rats, and this oxidative stress is decreased by antioxidant supplementation. ALA restored the expression levels of eNOS and nNOS and inhibited the degeneration of bladder smooth muscle cells.
Figures and Tables
Fig. 1
The expressions of 8-hydroxy-2'-deoxyguanosine in the urothelium and muscle cells of the bladder. Data are given as the means and SEM for the percentage of cells showing 8-hydroxy 2'-deoxyguanosine immunoreactivity. *p<0.01, †p=0.078. ALA50: diabetic rats treated with alpha-lipoic acid at 50mg/kg/day, ALA100: diabetic rats treated with alpha-lipoic acid at 100mg/kg/day. ALA: alpha-lipoic acid.
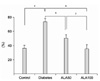
Fig. 2
Immunostaining of 8-hydroxy-2'-deoxyguanosine (8-OHdG) in the bladder. The black arrows indicate the nuclei of the urothelium and smooth muscle cells expressing immunoreactive 8-OHdG. The arrowheads indicate negative findings for 8-OHdG. A: control, B: diabetes, C: ALA50, D: ALA100 groups (×200). ALA: alpha-lipoic acid.
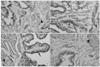
Fig. 3
The expressions of eNOS and nNOS proteins. A: Western blot analyses of eNOS and nNOS protein expression levels, B, C: quantification of data, corrected for beta-actin, in each sample. *: p<0.05. eNOS: endothelial nitric oxide synthase, nNOS: neuronal nitric oxide synthase.
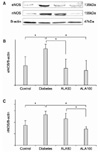
Fig. 4
Cylindrical profiles of longitudinally sectioned detrusor smooth muscle cells. The black arrows indicate dense bodies, and open arrows electron-dense bands. The asterisk indicates vacuolation. Many mitochondria are seen in the smooth muscle cells of the control (A), whereas few are seen in the diabetes group (B), ALA treatment restored the number of mitochondria in the smooth muscle cells (C, D). A: control, B: diabetes, C: ALA50, D: ALA100 groups (final magnification, ×4,000). ALA: alpha-lipoic acid.
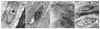
Table 1
Body weights and blood glucose levels
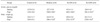
Data are expressed as mean±SEM. *the blood glucose level was significantly increased in the diabetes, ALA50, and ALA100 groups compared with the control group (p<0.05), †the blood glucose level was higher in the ALA50 group than in the ALA100 group (p<0.05), ‡the body weight was significantly decreased in the diabetes, ALA50, and ALA100 groups compared with the control group (p<0.05). ALA: alpha-lipoic acid
References
1. Kim SA, Park WS, Ohrr HC, Kang HY, Lee DH, Yi SW, et al. Prevalence and management status of diabetes mellitus in Korea. Korean J Med. 2005. 68:10–17.
2. Brown JS, Wessells H, Chancellor MB, Howards SS, Stamm WE, Stapleton AE, et al. Urologic complications of diabetes. Diabetes Care. 2005. 28:177–185.
3. Wolff SP. Diabetes mellitus and free radicals. Free radicals, transition metals and oxidative stress in the aetiology of diabetes mellitus and complications. Br Med Bull. 1993. 49:642–645.
4. Sies H, Stahl W. Vitamins E and C, beta-carotene, and other carotenoids as antioxidants. Am J Clin Nutr. 1995. 62(6):Suppl. 1315S–1321S.
5. Reed LJ, DeBusk BG, Gunsalus IC, Hornberger CS Jr. Crystalline alpha-lipoic acid: a catalytic agent associated with pyruvate dehydrogenase. Science. 1951. 27:93–94.
6. Packer L, Witt EH, Tritsckler HJ. Alpha-lipoic acid as a biological antioxidant. Free Radic Biol Med. 1995. 19:227–250.
7. Berman JR, McCarthy MM, Kyprianou N. Effect of estrogen withdrawal on nitric oxide synthase expression and apoptosis in the rat vagina. Urology. 1998. 51:650–656.
8. Khamaisi M, Potashnik R, Tirosh A, Demshchak E, Rudich A, Tritschler H, et al. Lipoic acid reduces glycemia and increases muscle GLUT4 content in streptozotocin-diabetic rats. Metabolism. 1997. 46:763–768.
9. Maritim AC, Sanders RA, Watkins JB 3rd. Effects of alpha-lipoic acid on biomarkers of oxidative stress in streptozotocin-induced diabetic rats. J Nutr Biochem. 2003. 14:288–294.
10. Arivazhagan P, Ramanathan K, Panneerselvam C. Effect of DL-alpha-lipoic acid on mitochondrial enzymes in aged rats. Chem Biol Interact. 2001. 138:189–198.
11. Jacob S, Streeper RS, Fogt DL, Hokama JY, Tritschler HJ, Dietze GJ, et al. The antioxidant alpha-lipoic acid enhances insulin-stimulated glucose metabolism in insulin-resistant rat skeletal muscle. Diabetes. 1996. 45:1024–1029.
12. Khamaisi M, Rudich A, Potashnik R, Tritschler HJ, Gutman A, Bashan N. Lipoic acid acutely induces hypoglycemia in fasting nondiabetic and diabetic rats. Metabolism. 1999. 48:504–510.
13. Leinonen J, Lehtimaki T, Toyokuni S, Okada K, Tanaka T, Hiai H, et al. New biomarker evidence of oxidative DNA damage in patients with non-insulin-dependent diabetes mellitus. FEBS Lett. 1997. 417:150–152.
14. Ihara Y, Toyokuni S, Uchida K, Odaka H, Tanaka T, Ikeda H, et al. Hyperglycemia causes oxidative stress in pancreatic beta-cells of GK rats, a model of type 2 diabetes. Diabetes. 1999. 48:927–932.
15. Cosentino F, Hishikawa K, Katusic ZS, Luscher TF. High glucose increases nitric oxide synthase expression and superoxide anion generation in human aortic endothelial cells. Circulation. 1997. 96:25–28.
16. Sen CK, Roy S, Han D, Packer L. Regulation of cellular thiols in human lymphocytes by alpha-lipoic acid: a flow cytometric analysis. Free Radic Biol Med. 1997. 22:1241–1257.
17. Heitzer T, Krohn K, Albers S, Meinertz T. Tetrahydrobiopterin improves endothelium-dependent vasodilation by increasing nitric oxide activity in patients with Type II diabetes mellitus. Diabetologia. 2000. 43:1435–1438.
18. Minamiyama Y, Bito Y, Takemura S, Takahashi Y, Kodai S, Mizuguchi S, et al. Calorie restriction improves cardiovascular risk factors via reduction of mitochondrial reactive oxygen species in type II diabetic rats. J Pharmacol Exp Ther. 2007. 320:535–543.
19. Jesmin S, Zaedi S, Maeda S, Yamaguchi I, Goto K, Miyauchi T. Effects of a selective endothelin a receptor antagonist on the expressions of iNOS and eNOS in the heart of early streptozotocin-induced diabetic rats. Exp Biol Med (Maywood). 2006. 231:925–931.
20. Park HJ, Park BK, Park NC. Penile expression of hypoxiainducible factor-1alpha, vascular endothelial growth factor, and nitric oxide synthase in the type II diabetic rat. Korean J Androl. 2005. 23:144–152.
21. Oh BR, Kim HJ, Ahn KY, Kwon DD, Ryu SB, Park YI. Change of nitric oxide synthase gene and protein expressions after bacillus calmette-guerin instillation into rat bladder. Korean J Urol. 2001. 42:413–419.
22. Surendran S, Kondapaka SB. Altered expression of neuronal nitric oxide synthase in the duodenum longitudinal musclemyenteric plexus of obesity induced diabetes mouse: implications on enteric neurodegeneration. Biochem Biophys Res Commun. 2005. 338:919–922.
23. Shotton HR, Clarke S, Lincoln J. The effectiveness of treatments of diabetic autonomic neuropathy is not the same in autonomic nerves supplying different organs. Diabetes. 2003. 52:157–164.
24. Park JW, Park SJ, Park SH, Kim KY, Chung JW, Chun MH, et al. Up-regulated expression of neuronal nitric oxide synthase in experimental diabetic retina. Neurobiol Dis. 2006. 21:43–49.
25. Elbadawi A. Functional pathology of urinary bladder muscularis: the new frontier in diagnostic uropathology. Semin Diagn Pathol. 1993. 10:314–354.
26. Nishikawa T, Edelstein D, Du XL, Yamagishi S, Matsumura T, Kaneda Y, et al. Normalizing mitochondrial superoxide production blocks three pathways of hyperglycaemic damage. Nature. 2000. 404:787–790.
27. Skulachev VP. Bioenergetic aspects of apoptosis, necrosis and mitoptosis. Apoptosis. 2006. 11:473–485.