Abstract
IL-9 is a known T cell growth factor with pleiotropic immunological functions, especially in parasite infection and colitis. However, its role in tumor growth is controversial. In this study, we generated tumor clones expressing the membrane-bound form of IL-9 (MB-IL-9) and investigated their influences on immune system. MB-IL-9 tumor clones showed reduced tumorigenicity but shortened survival accompanied with severe body weight loss in mice. MB-IL-9 expression on tumor cells had no effect on cell proliferation or major histocompatibility complex class I expression in vitro. MB-IL-9 tumor clones were effective in amplifying CD4+ and CD8+ T cells and increasing cytotoxic activity against CT26 cells in vivo. We also observed a prominent reduction in body weights and survival period of mice injected intraperitoneally with MB-IL-9 clones compared with control groups. Ratios of IL-17 to interferon (IFN)-γ in serum level and tumor mass were higher in mice implanted with MB-IL-9 tumor clones than those observed in mice implanted with control cells. These results indicate that the ectopic expression of the MB-IL-9 on tumor cells exerts an immune-stimulatory effect with toxicity. To exploit its benefits as a tumor vaccine, a strategy to control the toxicity of MB-IL-9 tumor clones should be developed.
References
1. Uyttenhove C, Simpson RJ, Van Snick J. Functional and structural characterization of P40, a mouse glycoprotein with T-cell growth factor activity. Proc Natl Acad Sci U S A. 1988; 85:6934–6938.


2. Schmitt E, Bopp T. Amazing IL-9: revealing a new function for an “old” cytokine. J Clin Invest. 2012; 122:3857–3859.


3. Renauld JC, van der Lugt N, Vink A, van Roon M, Godfraind C, Warnier G, Merz H, Feller A, Berns A, Van Snick J. Thymic lymphomas in interleukin 9 transgenic mice. Oncogene. 1994; 9:1327–1332.
4. Veldhoen M, Uyttenhove C, van Snick J, Helmby H, Westendorf A, Buer J, Martin B, Wilhelm C, Stockinger B. Transforming growth factor-beta ‘reprograms'the differentiation of T helper 2 cells and promotes an interleukin 9-producing subset. Nat Immunol. 2008; 9:1341–1346.


5. Dardalhon V, Awasthi A, Kwon H, Galileos G, Gao W, Sobel RA, Mitsdoerffer M, Strom TB, Elyaman W, Ho IC, et al. IL-4 inhibits TGF-beta-induced Foxp3+ T cells and, together with TGF-beta, generates IL-9+ IL-10+ Foxp3 (-) effector T cells. Nat Immunol. 2008; 9:1347–1355.


6. Beriou G, Bradshaw EM, Lozano E, Costantino CM, Hastings WD, Orban T, Elyaman W, Khoury SJ, Kuchroo VK, Baecher-Allan C, et al. TGF-beta induces IL-9 production from human Th17 cells. J Immunol. 2010; 185:46–54.
7. Lu LF, Lind EF, Gondek DC, Bennett KA, Gleeson MW, Pino-Lagos K, Scott ZA, Coyle AJ, Reed JL, Van Snick J, et al. Mast cells are essential intermediaries in regulatory T-cell tolerance. Nature. 2006; 442:997–1002.


8. Stassen M, Schmitt E, Bopp T. From interleukin-9 to T helper 9 cells. Ann N Y Acad Sci. 2012; 1247:56–68.


9. Chang HC, Sehra S, Goswami R, Yao W, Yu Q, Stritesky GL, Jabeen R, McKinley C, Ahyi AN, Han L, et al. The transcription factor PU.1 is required for the development of IL-9-producing T cells and allergic inflammation. Nat Immunol. 2010; 11:527–534.


10. Brüstle A, Heink S, Huber M, Rosenplänter C, Stadelmann C, Yu P, Arpaia E, Mak TW, Kamradt T, Lohoff M. The development of inflammatory T(h)-17 cells requires interferon-regulatory factor 4. Nat Immunol. 2007; 8:958–966.


11. Purwar R, Schlapbach C, Xiao S, Kang HS, Elyaman W, Jiang X, Jetten AM, Khoury SJ, Fuhlbrigge RC, Kuchroo VK, et al. Robust tumor immunity to melanoma mediated by interleukin-9-producing T cells. Nat Med. 2012; 18:1248–1253.


12. Kim IK, Kim BS, Koh CH, Seok JW, Park JS, Shin KS, Bae EA, Lee GE, Jeon H, Cho J, et al. Glucocorticoid-induced tumor necrosis factor receptor-related protein costimulation facilitates tumor regression by inducing IL-9-producing helper T cells. Nat Med. 2015; 21:1010–1017.


13. Parrot T, Allard M, Oger R, Benlalam H, Raingeard de la Bletiere D, Coutolleau A, Preisser L, Desfrancois J, Khammari A, Dreno B, et al. IL-9 promotes the survival and function of human melanoma-infiltrating CD4 (+) CD8 (+) double-positive T cells. Eur J Immunol. 2016; 46:1770–1782.


14. Fang Y, Chen X, Bai Q, Qin C, Mohamud AO, Zhu Z, Ball TW, Ruth CM, Newcomer DR, Herrick EJ, et al. IL-9 inhibits HTB-72 melanoma cell growth through upregulation of p21 and TRAIL. J Surg Oncol. 2015; 111:969–974.


15. Lu Y, Hong B, Li H, Zheng Y, Zhang M, Wang S, Qian J, Yi Q. Tumor-specific IL-9-producing CD8+ Tc9 cells are superior effector than type-I cytotoxic Tc1 cells for adoptive immunotherapy of cancers. Proc Natl Acad Sci U S A. 2014; 111:2265–2270.


16. Lu Y, Hong S, Li H, Park J, Hong B, Wang L, Zheng Y, Liu Z, Xu J, He J, et al. Th9 cells promote antitumor immune responses in vivo. J Clin Invest. 2012; 122:4160–4171.
17. Liu JQ, Li XY, Yu HQ, Yang G, Liu ZQ, Geng XR, Wang S, Mo LH, Zeng L, Zhao M, et al. Tumor-specific Th2 responses inhibit growth of CT26 colon-cancer cells in mice via converting intratumor regulatory T cells to Th9 cells. Sci Rep. 2015; 5:10665.
18. Rivera Vargas T, Humblin E, Végran F, Ghiringhelli F, Apetoh L. TH9 cells in anti-tumor immunity. Semin Immunopathol. 2017; 39:39–46.


19. Huang Y, Cao Y, Zhang S, Gao F. Association between low expression levels of interleukin-9 and colon cancer progression. Exp Ther Med. 2015; 10:942–946.


20. Zhao Y, Chu X, Chen J, Wang Y, Gao S, Jiang Y, Zhu X, Tan G, Zhao W, Yi H, et al. Dectin-1-activated dendritic cells trigger potent antitumour immunity through the induction of Th9 cells. Nat Commun. 2016; 7:12368.


21. Nakatsukasa H, Zhang D, Maruyama T, Chen H, Cui K, Ishikawa M, Deng L, Zanvit P, Tu E, Jin W, et al. The DNA-binding inhibitor Id3 regulates IL-9 production in CD4 (+) T cells. Nat Immunol. 2015; 16:1077–1084.


22. Grimbaldeston MA, Chen CC, Piliponsky AM, Tsai M, Tam SY, Galli SJ. Mast cell-deficient W-sash c-kit mutant Kit W-sh/W-sh mice as a model for investigating mast cell biology in vivo. Am J Pathol. 2005; 167:835–848.


23. Abdul-Wahid A, Cydzik M, Prodeus A, Alwash M, Stanojcic M, Thompson M, Huang EH, Shively JE, Gray-Owen SD, Gariepy J. Induction of antigen-specific TH 9 immunity accompanied by mast cell activation blocks tumor cell engraftment. Int J Cancer. 2016; 139:841–853.


24. Hoelzinger DB, Dominguez AL, Cohen PA, Gendler SJ. Inhibition of adaptive immunity by IL9 can be disrupted to achieve rapid T-cell sensitization and rejection of progressive tumor challenges. Cancer Res. 2014; 74:6845–6855.


25. Lv X, Feng L, Ge X, Lu K, Wang X. Interleukin-9 promotes cell survival and drug resistance in diffuse large B-cell lymphoma. J Exp Clin Cancer Res. 2016; 35:106.


26. Zhang J, Wang WD, Geng QR, Wang L, Chen XQ, Liu CC, Lv Y. Serum levels of interleukin-9 correlate with negative prognostic factors in extranodal NK/T-cell lymphoma. PLoS One. 2014; 9:e94637.


27. Knoops L, Renauld JC. IL-9 and its receptor: from signal transduction to tumorigenesis. Growth Factors. 2004; 22:207–215.


28. Fischer M, Bijman M, Molin D, Cormont F, Uyttenhove C, van Snick J, Sundstrom C, Enblad G, Nilsson G. Increased serum levels of interleukin-9 correlate to negative prognostic factors in Hodgkin's lymphoma. Leukemia. 2003; 17:2513–2516.


29. Chen N, Lu K, Li P, Lv X, Wang X. Overexpression of IL-9 induced by STAT6 activation promotes the pathogenesis of chronic lymphocytic leukemia. Int J Clin Exp Pathol. 2014; 7:2319–2323.
30. Elyaman W, Bradshaw EM, Uyttenhove C, Dardalhon V, Awasthi A, Imitola J, Bettelli E, Oukka M, van Snick J, Renauld JC, et al. IL-9 induces differentiation of TH17 cells and enhances function of FoxP3+ natural regulatory T cells. Proc Natl Acad Sci U S A. 2009; 106:12885–12890.


32. Do Thi VA, Park SM, Lee H, Kim YS. The membrane-bound form of IL-17A promotes the growth and tumorigenicity of colon cancer cells. Mol Cells. 2016; 39:536–542.


33. Horiuchi T, Mitoma H, Harashima S, Tsukamoto H, Shimoda T. Transmembrane TNF-alpha: structure, function and interaction with anti-TNF agents. Rheumatology (Oxford). 2010; 49:1215–1228.


34. Väyrynen JP, Kantola T, Väyrynen SA, Klintrup K, Bloigu R, Karhu T, Mäkelä J, Herzig KH, Karttunen TJ, Tuomisto A, et al. The relationships between serum cytokine levels and tumor infiltrating immune cells and their clinical significance in colorectal cancer. Int J Cancer. 2016; 139:112–121.


35. Leung S, Liu X, Fang L, Chen X, Guo T, Zhang J. The cytokine milieu in the interplay of pathogenic Th1/Th17 cells and regulatory T cells in autoimmune disease. Cell Mol Immunol. 2010; 7:182–189.


36. Lundy SK, Fox DA. Reduced Fas ligand-expressing splenic CD5+ B lymphocytes in severe collagen-induced arthritis. Arthritis Res Ther. 2009; 11:R128.


37. Chang MR, Lee WH, Choi JW, Park SO, Paik SG, Kim YS. Antitumor immunity induced by tumor cells engineered to express a membrane-bound form of IL-2. Exp Mol Med. 2005; 37:240–249.


38. Kim HJ, Park SM, Lee H, Kim YS. Membrane-bound p35 Subunit of IL-12 on tumor cells is functionally equivalent to membrane-bound heterodimeric single chain IL-12 for induction of anti-tumor immunity. Immune Netw. 2016; 16:305–310.


Figure 1.
Generation of CT26 tumor clones expressing the membrane-bound form of IL-9. (A) The chimeric MB-IL-9 cDNA comprised the cDNA encoding the CY (from −75 to −45) and TM (from −44 to −24) of murine TNF-α and IL-9 cDNA without signal sequence (54–435). A spacer sequence (Gly-Gly-Ils) was inserted between TNF-α and IL-9. (B) MB-IL-9 transfectants were analyzed for IL-9 expression using RT-PCR. Two positive clones of the membrane-bound form (MB-1 and MB-7 clones) were chosen for further experiments. One million cells of MB-IL-9 tumor clones were cultured for 24 h and culture supernatants (C) and cells (D) were separated to quantitate MB-IL-9 protein expression by ELISA. A representative result was presented from more than 3 independent experiments. (E) The expression of IL-9 on cell surface was analyzed by flow cytometry analysis using polyclonal anti-IL-9 antibody. The statistical significance of the differences between mock-vector group vs. MB-IL-9 groups were analyzed by 1-way ANOVA. CY, cytoplasmic domain; TM, transmembrane domain; SS, signal sequence; Medium, complete RPMI medium; P, wild-type CT26; M, mock vector-transfected clone; NS, not significant. ** p<0.01.
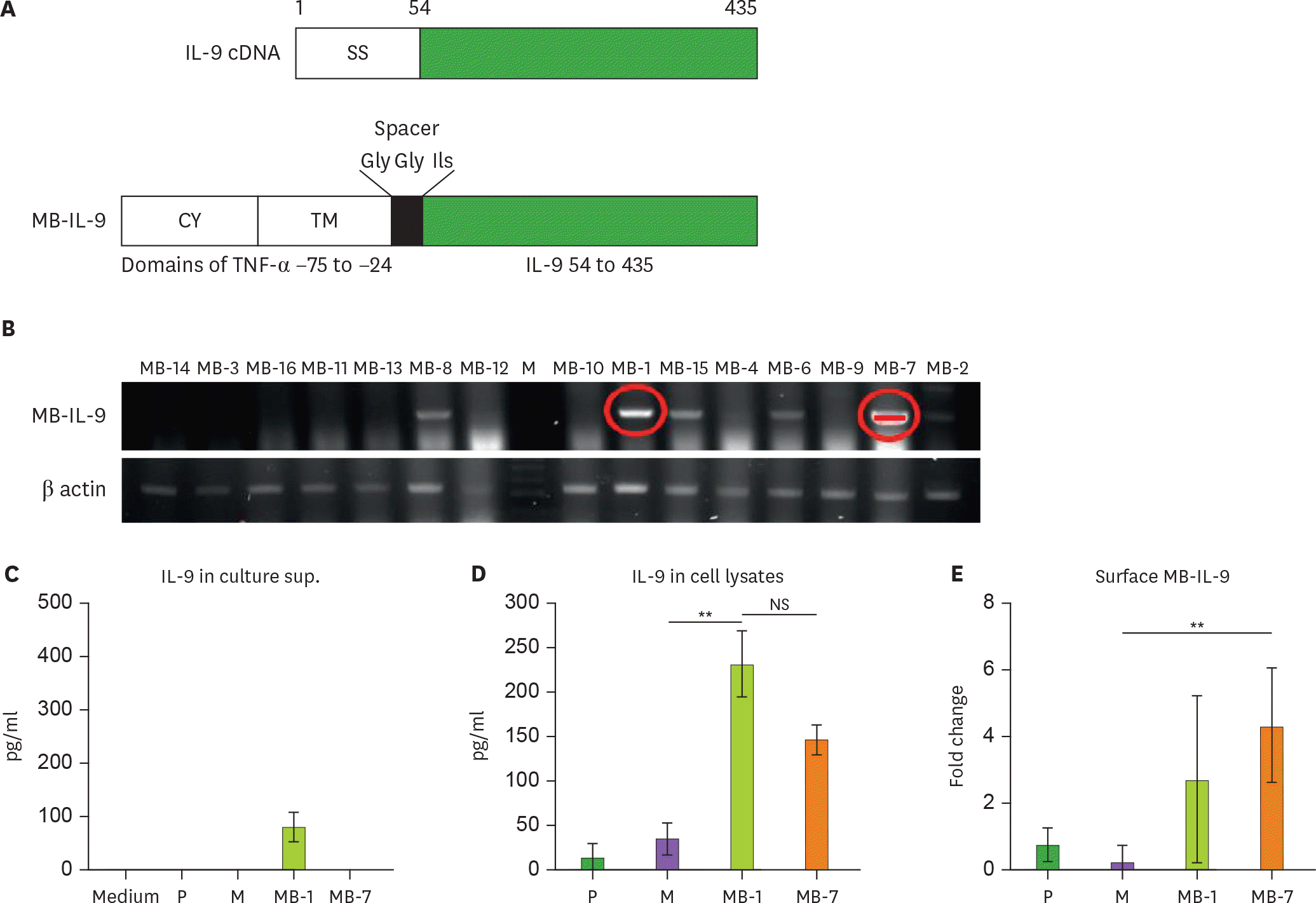
Figure 2.
Tumor growth and survival of mice implanted with MB-IL-9 tumor clones. (A) BALB/c mice (n=5) were s.c. injected with indicated number (1, 0.5, or 0.1 million) of MB-IL-9 tumor clones (MB-1 and MB-7 clones) and tumor growth monitored. Comparable tumor volume measured at the indicated dates after injection was presented. The statistical significance of the differences between mock-vector group vs. MB-IL-9 groups were analyzed by 1-way ANOVA. (B) One of mice from each group was randomly chosen and sacrificed at indicated dates to visualize tumor mass. The weight of isolated tumor mass was also measured. (C) Survival of mice in each experiment was estimated. Mean survival (days) was indicated insets. Two independent survival experiments were repeated in case of 0.5 and 0.1 million cell injections. One of representative results was presented. (D) Body weights of mice injected with 0.5 million cells of MB-IL-9 tumor clones were simultaneously measured. A group of mice (n=5) with same age was used as control for body weight change. P, wild-type CT26; M, mock vector-transfected clone; NS; not significant. * p<0.05. (continued to the next page)
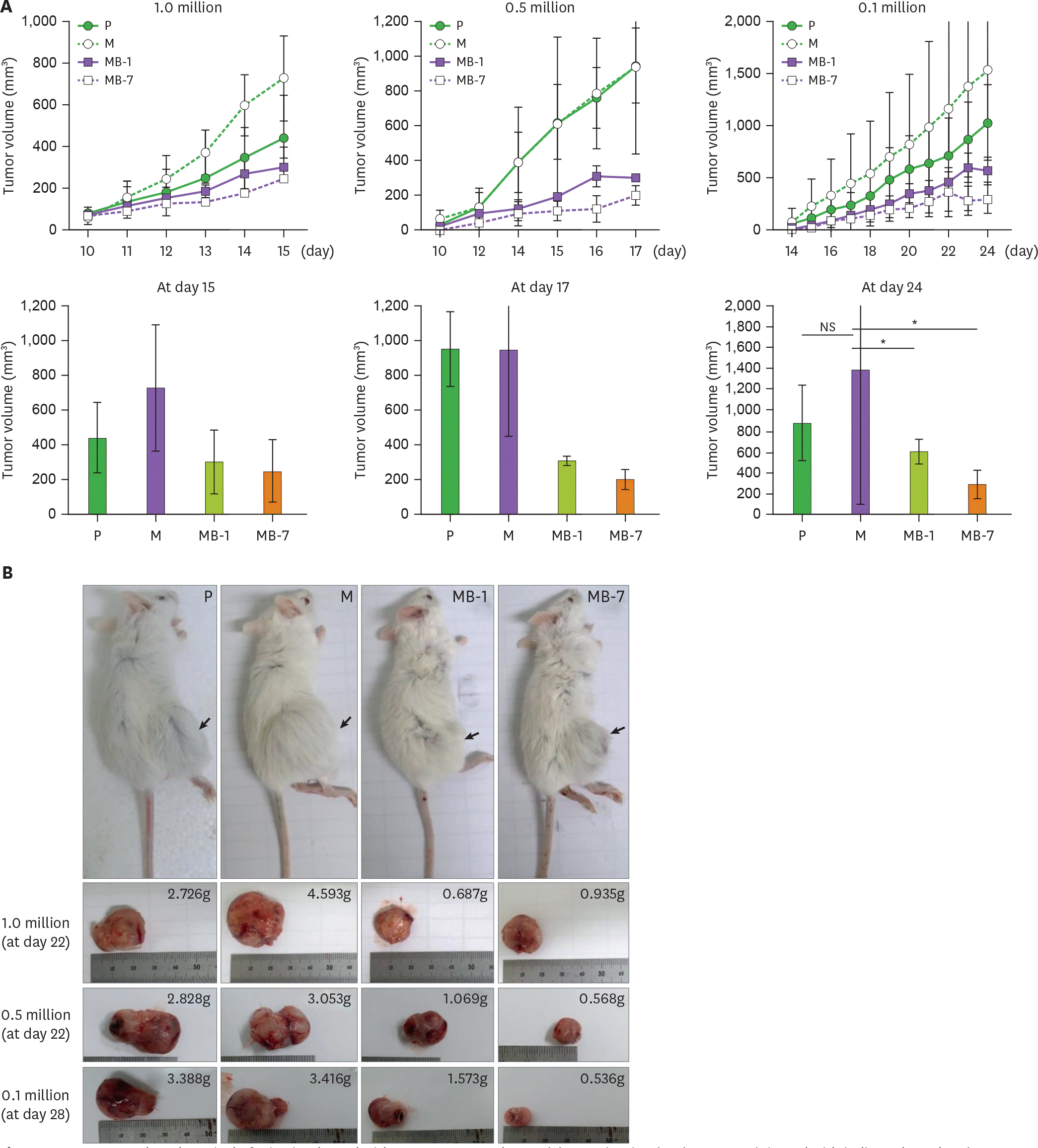
Figure 3.
Effects of MB-IL-9 tumor clones on T lymphocytes subsets in spleens. (A) Two mice per group were implanted with MB-IL-9 tumor clones or control cells (1 million cells), and one of mice per group was sacrificed at day 15 to analyze CD4+ and CD8+ T cell subsets. (B) The other mouse of each group was also sacrificed at day 17 for the same analysis, and average proportions of T cell subsets were presented. P, wild-type CT26; M, mock vector-transfected clone. The p-values were * p<0.05, ** p<0.01, *** p<0.001. Difference alphabets mean follow as; a average±standard deviation from tumor bearing mice, b statistical significance was assessed by 1-way ANOVA to evaluate differences in T lymphocytes between MB-IL-9 groups and mock controls.
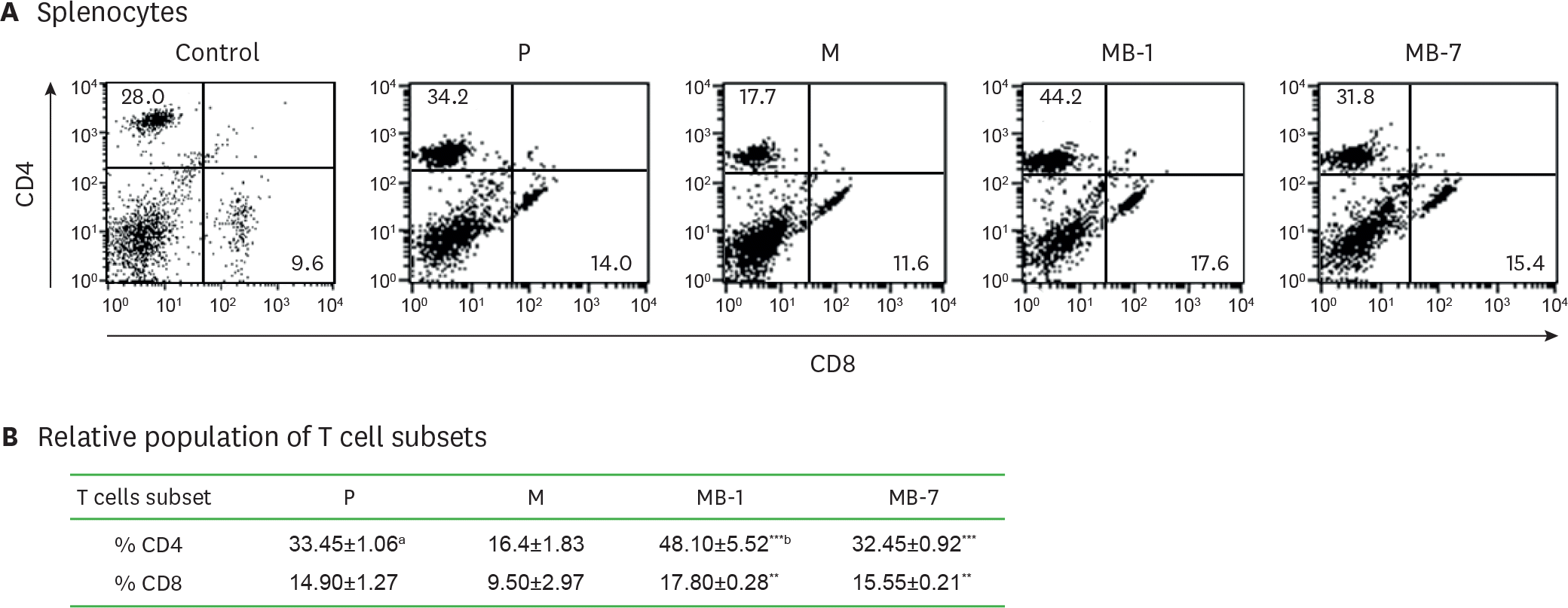
Figure 4.
Cytotoxic activity of spleen cells immunized with MB-IL-9 tumor clones. (A) Splenocytes were isolated from mice pre-immunized with MMC-inactivated CT26 cells and stimulated in vitro for 56 h with MMC-inactivated MB-IL-9 clones at different ratios (spleen cells: stimulators=10:1 or 50:1). The morphology of cells in the mixed cell culture was recorded under a microscope. A representative result was shown from 3 independent experiments. (B) Spleen cells activated in vivo and in vitro were assayed for cytotoxic activity against wild-type CT26 cells. The activated spleen cells were harvested and incubated for 3.5 h with CFSE-stained wild-type CT26 cells at 3 different E:T ratios (1;1, 5:1, 50:1) in triplicate, followed by their treatment with PI before flow cytometry analysis. The ratio of PI+ cells among CFSE+ population was estimated cytotoxic activity of splenocytes. A representative result at 50:1 ratio was presented from 2 independent experiments. The p-value between M and MB-1 or MB-7 was less than 0.07 or 0.08, respectively. P, wild-type CT26; M, mock vector-transfected clone.
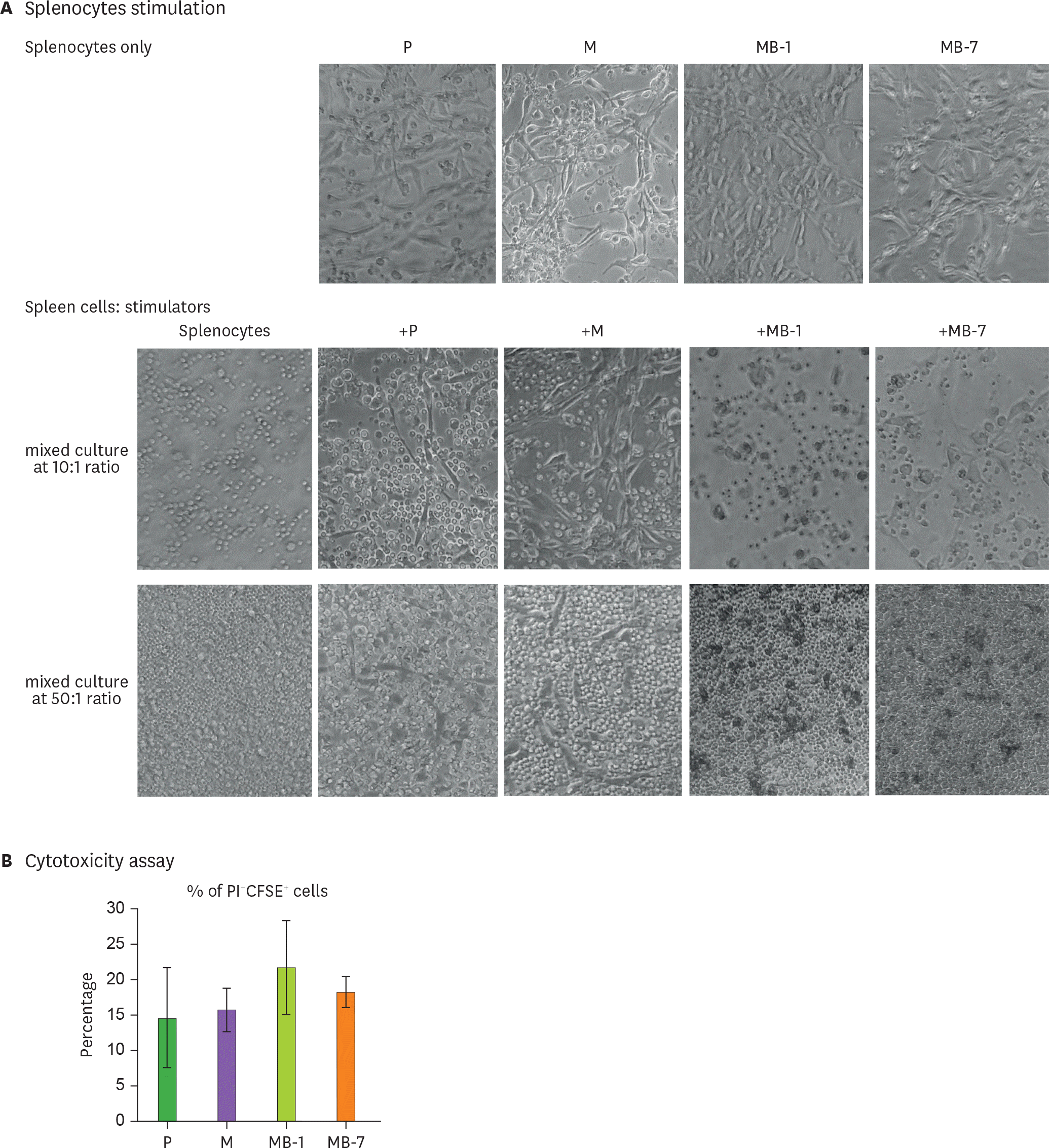
Figure 5.
Toxicity of MB-IL-9 tumor clones in vivo. BALB/c mice (n=5) were i.p. injected with one million MB-IL-9 tumor clones. Body weights (A) and survival (B) of mice were monitored daily. (C) At day 12, one of mice from each group was randomly chosen to sacrifice and the presence of lumps of tumor cells in the abdominal cavity examined. Arrows indicate the lumps of tumor cells. P, wild-type CT26; M, mock vector-transfected clone.
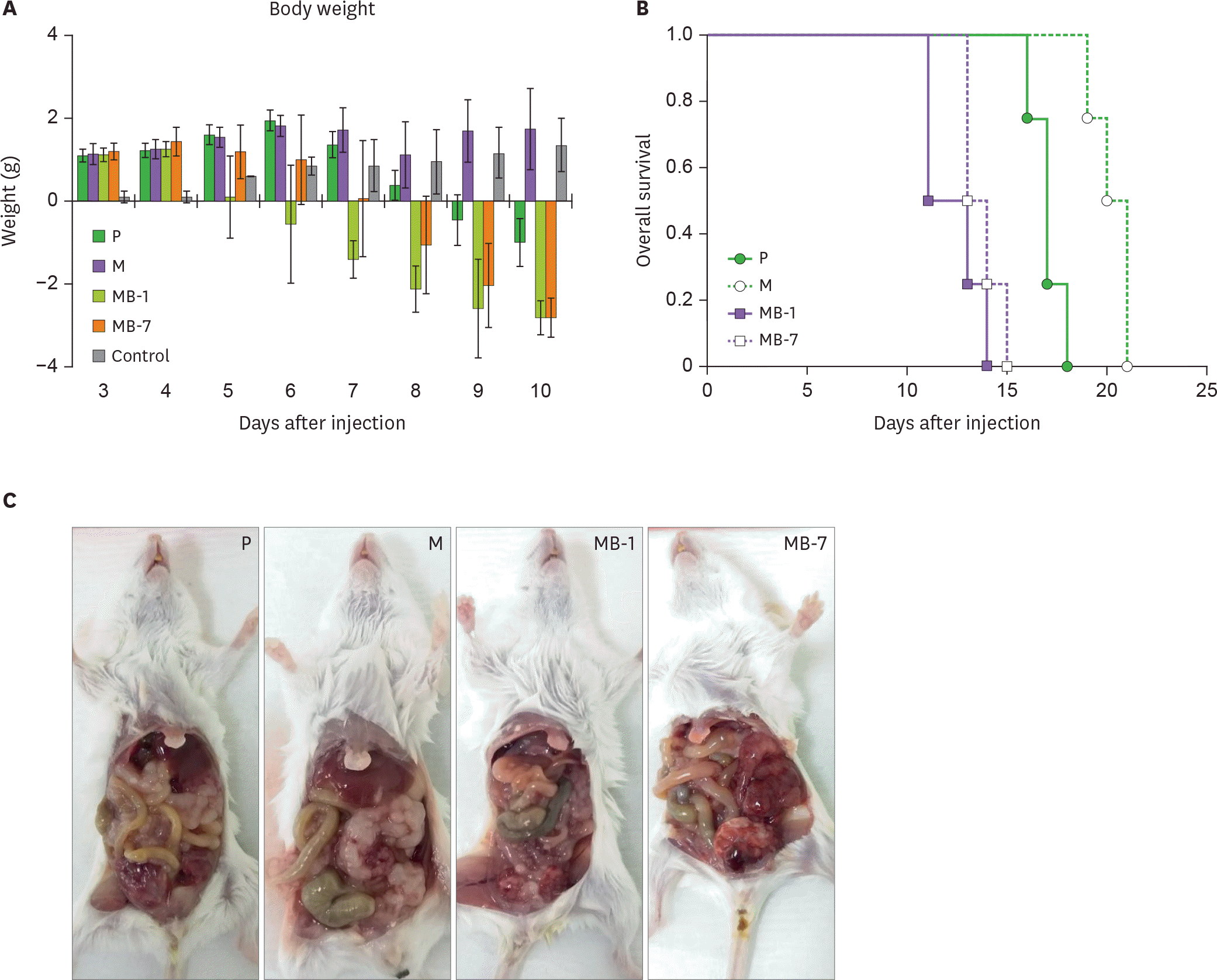
Figure 6.
Levels of cytokines in the serum and tumor mass. At day 21 after the s.c. injection of 0.5 million MB-IL-9 clones per mouse, blood was collected from the eye artery (A) and tumor mass isolated (B) to quantitate various cytokines. To analyze cytokines in tumor mass, 0.5 g of tumor mass from each group was lysed using a lysing buffer. The IL-17/IFN-γ ratio was calculated and presented at the lowest panel (C). Cytokines were quantitated by ELISA. The statistical significance of the differences between mock-vector group vs. MB-IL-9 groups were analyzed by 1-way ANOVA. Control; tumor free mice, P; wild-type CT26, M; mock vector-transfected clone. * p<0.05, ** p<0.01.
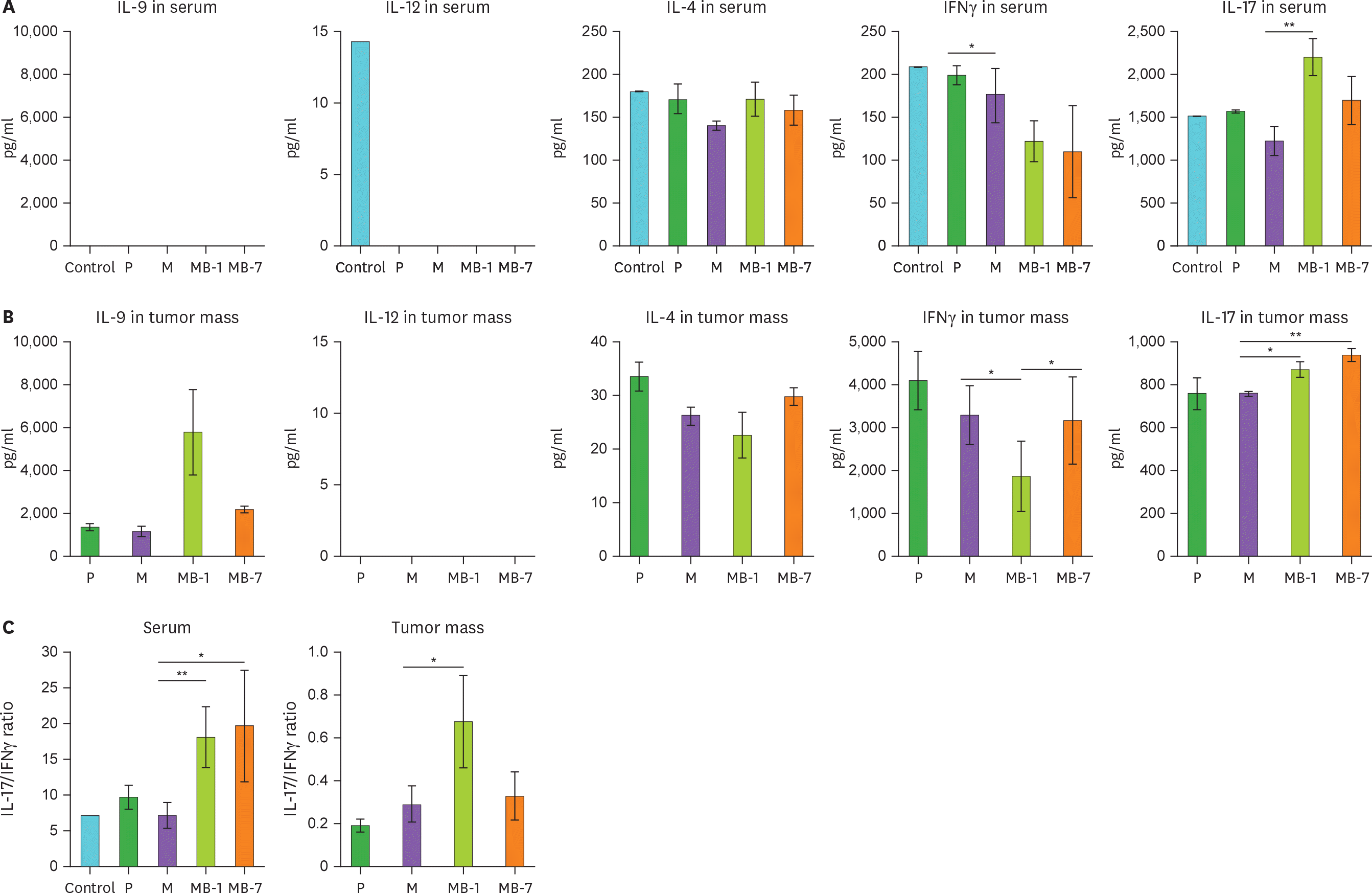