Abstract
Interleukin-7 (IL-7) is an essential cytokine for T cells. However, IL-7 is not produced by T cells themselves such that T cells are dependent on extrinsic IL-7. In fact, in the absence of IL-7, T cell development in the thymus as well as survival of naive T cells in the periphery is severely impaired. Furthermore, modulating IL-7 availability in vivo either by genetic means or other experimental approaches determines the size, composition and function of the T cell pool. Consequently, understanding IL-7 expression is critical for understanding T cell immunity. Until most recently, however, the spatiotemporal expression of in vivo IL-7 has remained obscured. Shortage of such information was partly due to scarce expression of IL-7 itself but mainly due to the lack of adequate reagents to monitor IL-7 expression in vivo. This situation dramatically changed with a recent rush of four independent studies that describe the generation and characterization of IL-7 reporter mice, all utilizing bacterial artificial chromosome transgene technology. The emerging consensus of these studies confirmed thymic stromal cells as the major producers of IL-7 but also identified IL-7 reporter activities in various peripheral tissues including skin, intestine and lymph nodes. Strikingly, developmental and environmental cues actively modulated IL-7 reporter activities in vivo suggesting that IL-7 regulation might be a new mechanism of shaping T cell development and homeostasis. Collectively, the availability of these new tools opens up new venues to assess unanswered questions in IL-7 biology in T cells and beyond.
Originally described as a pre-B cell growth factor expressed in bone marrow stromal cells (1), interleukin-7 (IL-7) was subsequently identified as an essential and non-redundant cytokine in T cells (2,3). In fact, IL-7 plays a critical role in every aspect of T cell biology, including early T cell development in the thymus, CD4/CD8 lineage choice during positive selection as well as maintaining the survival and homeostasis of naïve and memory T cells in the periphery (2-6). Genetic deficiencies in IL-7 or in any component of the IL-7 receptor (IL-7R) complex, namely the IL-7R alpha (IL-7Rα) chain and the cytokine receptor shared common gamma (γc)-chain, result in severely impaired thymopoiesis and T cell survival. IL-7 deficiency is manifested in humans as T(-)B(+)NK(-) SCID (severe combined immunodeficiency) (7,8) and in mice as severe deficiency in both B and T cells (2,3,9). The requirement of IL-7 in T cell development and survival is chiefly due to its anti-apoptotic effects by upregulating expression of Bcl-2 and Mcl-1 (10,11), but also because of its trophic effects, such as inducing expression of glucose transporter-1 (12). In mature naïve T cells, IL-7 is a non-redundant survival cytokine that cannot be replaced by other pro-survival cytokines (4,5,13,14). This non-redundancy is also partly the case for memory T cell maintenance (15). Interestingly, antigen specific memory CD8 T cells are still largely dependent on IL-7 for cell survival but intermittent and basal homeostatic proliferation of these cells seems to be more dependent on IL-15 (4,16-18). This is in contrast to the bulk of memory phenotype CD8 T cells which are dependent on IL-15 but not IL-7 (19). The molecular basis for such different cytokine requirements is unclear. Nevertheless, it remains evident that IL-7 is a key molecule for maintaining the fitness and size of the peripheral T cell pool by providing essential survival signals.
IL-7 signaling is transduced by the IL-7R heterodimer, which is composed of the IL-7-specific IL-7R α-chain and the γc-chain, which is a shared component with other members of the γc-cytokine family, i.e. IL-2, IL-4, IL-7, IL-9, IL-15 and IL-21 (20). Ligand-induced heterodimerization of the IL-7Rα/γc-chains leads to juxtaposition and trans-phosphorylation of receptor-associated tyrosine kinases JAK1 and JAK3 (21). Activated JAKs then phosphorylate specific tyrosine residues in the IL-7Rα cytosolic domain that attract STAT5 (Signal Transducers and Activators of Transcription 5) and PI-3 kinases for JAK-mediated phosphorylation and further downstream signaling. Known downstream molecules of IL-7 signaling include among others Bcl-2, Bcl-XL, Mcl-1, Glut-1, Cdc25A, IL-7Rα, Runx3, and CD8 coreceptors, which are all essential for functional fitness and survival of T cells (6,10,12,22-25).
Notably, multiple studies have documented that IL-7 functions beyond serving as a pro-survival factor. For example, IL-7 provides specific differentiation signals in immature lymphocytes by opening up the TCRγ-chain and the immunoglobulin heavy chain locus for antigen receptor recombination (26,27). In this line, we recently showed that IL-7 is necessary to specify CD8 lineage differentiation during CD4/CD8 cell fate choice in the thymus by inducing expression of the transcription factor Runx3 (6). Additionally, IL-7 is involved in the development of secondary lymphoid tissues since impaired IL-7 signaling results in defects in lymph node organogenesis (28). Here, IL-7 seems to regulate the size of the fetal lymphoid tissue inducer (LTi) cell pool that interacts with stromal organizer cells to form lymphoid tissues (29). Thus, IL-7 is a critical player in cell lineage choice and differentiation during lymphocyte as well as lymphoid tissue development. Furthermore, IL-7 also controls homeostasis of several innate lymphoid cell subsets (30). Within the T cell compartment, IL-7 controls the TCR activation threshold of naïve CD8 T cells by tuning CD8 coreceptor expression levels to the self-specificity of the TCR. In this way, IL-7 maintains a self-reactive but not autoimmune T cell pool (31). Finally, IL-7 downregulates expression of its own receptor. IL-7 signaled cells express lower surface levels of IL-7R so that unsignaled cells outcompete IL-7 signaled T cells for remaining IL-7. We previously showed that maintaining this negative feedback loop is necessary for maximizing the size of the naïve T cell pool (32). Collectively, IL-7 is a multi-faceted pro-survival cytokine that governs the development, differentiation, and maintenance of T cells in a non-redundant manner.
Despite playing such critical roles, however, there is remarkably little known about IL-7 expression and regulation in vivo. In fact, the identity of IL-7 producing cells in the thymus is not fully understood as well as the precise location of IL-7 expression in peripheral tissues remains veiled. Recently, much light was shed on the in vivo source and regulation of IL-7 expression by a series of four independent publications reporting the generation and characterization of IL-7 reporter mice. Using fluorescent proteins, Cre recombinase and other reporter proteins, IL-7 transcriptional activities were monitored in vivo and so depicted a spatiotemporal expression profile of IL-7 expression during ontogeny and steady state conditions. Here we review the experimental results and their findings in context of IL-7 mediated T cell development and peripheral homeostasis.
Constant IL-7 signaling is critical for mature T cells. Adoptive transfer of naïve T cells into an IL-7 deficient environment fail to induce homeostatic proliferation and transferred cells succumb to programmed cell death (4,5). Even as all T lineage cells are critically dependent on IL-7, no lymphocytes including T cells themselves produce IL-7 (33). Thus, T cells are dependent on exogenous IL-7 (4,5). Importantly, the anatomical locations where immature thymocytes and T cells encounter IL-7 for development and homeostasis are not entirely mapped. The cellular sources of IL-7 in these tissues are also still disputed. Previously, IL-7 message and proteins have been reported in various tissues including the thymus and secondary lymphoid organs such as spleen (34,35) and lymph nodes (36). Also, other tissues were implicated as sources of in vivo IL-7 which comprise the intestine (37), human liver (38), skin (39,40), murine ovaries (41), human brain (42), and human prostate (43). But the precise identities of IL-7 expressing cells remain still obscure.
While IL-7 is generally considered as a secreted cytokine, its expression levels in serum are extremely low suggesting that IL-7 in vivo is limiting and that IL-7 would be consumed locally. In fact, serum IL-7 was never convincingly detected in mice sera until most recently, when Mackall and colleagues were able to determine serum IL-7 in wild type mice at levels of 10 pg/ml (44). Scarce IL-7 in the circulation on the one hand suggests that IL-7 would be produced only at very low levels, but on the other hand there is also the possibility that IL-7 is sequestered from serum by being complexed to the extracellular matrix (ECM). Potentially, such immobilized IL-7 could be the actual bioactive form of IL-7 in vivo. Indeed, MHC class II+ thymic epithelial cells do express the ECM components fibronectin and heparin sulfate, and IL-7 signaling in immature thymocytes is likely mediated by an ECM-associated form of IL-7 (45). Moreover, IL-7 can also act as a heterodimer when bound to a variant β-chain of the hepatocyte growth factor (HGF), which is then known as the pre-pro-B cell growth-stimulating factor (PPBSF). Both heterodimerization of IL-7 and HGFβ and the biological activity of PPBSF are dependent on low levels of heparin sulfate-derived oligosaccharides. HGF is a cytokine homologous to plasminogen, and these data suggest that HGF β-chains could allow interaction of IL-7 with the stromal cell surface and thus deliver IL-7 signaling as a membrane bound cytokine (46). If this is indeed the case, then identifying the actual IL-7 producer cells in vivo would be even more critical to understand where and when IL-7 signaling is happening in T cells.
Without identifying IL-7 producers, however, it is difficult to understand whether and how IL-7 expression is controlled. Generally, IL-7 production in vivo is considered to be constitutive and unaffected by external signals, so that IL-7 availability is a limiting factor in determining the size of the peripheral T cell pool (47). On the other hand, there is a great body of evidence that IL-7 expression in vivo can be modulated. Serum IL-7 concentration is elevated in lymphopenic humans and other primates (48,49), and in the mouse, a novel IL-7R mediated IL-7 expression feedback loop was recently reported, further indicating that IL-7 production is actively regulated in vivo (44). Furthermore, IL-6 and TLR signaling in the liver have been shown to induce IL-7 expression in hepatocytes suggesting that expression of immunoregulatory IL-7 is broader and more dynamically regulated than currently presumed (50). Because of the lack of adequate reagents, however, the whole scope of IL-7 expression remained largely unaddressed.
Recently, four independent studies reported a total of five different IL-7 reporter mice whose detailed characterizations will be discussed below (51-54). All IL-7 reporter mice were generated by basically the same strategy employing BAC transgenesis and insertion of a reporter gene into the IL-7 locus. Individual cloning schematics are summarized in Fig. 1. In brief, IL-7 BAC transgenes were generated by insertion of a reporter gene immediately after the ATG start codon of IL-7 in exon 1, which effectively disrupts the BAC-encoded IL-7 gene (Fig. 1). Consequently, all IL-7 reporter BAC transgenes do not overexpress IL-7. The choices of the IL-7 reporter genes, however, were distinct between each study explaining possible variations among the reports (Table I).
The first IL-7 reporter mouse was generated and reported by Di Santo and colleagues (51). The BAC reporter construct expressed a yellow fluorescence protein (YFP) cDNA downstream of the ATG translational start codon of IL-7 exon 1, thus inactivating the BAC IL-7 open reading frame. Consequently, endogenous IL-7 levels are not perturbed and transgenic mice did not show any significant differences in the cellular composition of primary and secondary lymphoid organs. IL-7 reporter activities were monitored either by flow cytometry for YFP expression or by immunohistochemistry using anti-YFP antibodies. Both fetal and adult thymic stromal cells were found to contain YFP (IL-7) positive cells which were further identified as CD45- MHCII+ thymic epithelial cells (TEC) in the fetal thymus and as either medullary or cortical TEC-specific markers bearing cells in the adult thymus. Interestingly, IL-7 reporter activity was not detected in either endothelial cells or fibroblasts, suggesting that only a subset of specialized TECs produce this cytokine. Of note, YFP expression was also not detected in peripheral lymphoid organs including the bone marrow, lymph node and spleen. This observation, however, does not agree with the general idea of peripheral IL-7 being necessary for T cell survival (4,5). A convincing explanation for this discrepancy, however, was provided when quantifying IL-7 mRNA transcripts in gp38+ fibroblastic reticular cells in the lymph node and comparing those to the IL-7 mRNA expression in YFP+ TECs. Fibroblastic reticular cells are an established source of peripheral IL-7 (36), but their IL-7 mRNA expression levels were found to be significantly lower than in YFP+ TECs. Therefore, IL-7 reporter activity in this particular reporter mouse identifies only cells with the highest levels of IL-7 transcription in vivo, which is clearly the case for thymic stromal cells as confirmed in subsequent studies by others.
Richie and colleagues chose to generate two different IL-7 reporter transgenes with either the human CD25 or the Cre recombinase cDNA inserted into nucleotide position 1 of the IL-7 exon 1 (52). The constructs were designed to replace the entire coding region of IL-7 exon 1 of the BAC clone RPCI-295A3 that contained the entire 43 kb IL-7 locus and its 5' and 3' flanking sequences (96 kb of the 5' sequence and 17 kb of the 3' of the Il7 locus) to include as much of the Il7 regulatory elements as possible. IL-7 reporter activities were monitored using either anti-human CD25 antibodies in IL-7.hCD25 transgenes or by crossing IL-7.Cre transgenic mice with Rosa26R (55) and R26YFP (56) reporter strains to induce expression of either β-galactosidase or YFP, respectively (52). Reporter gene expression was detected either by flow cytometry or in whole mount staining by X-gal and by anti-YFP antibodies. In these reporter strains, IL-7 expression faithfully reproduced the results from a previous study where in situ hybridization detected IL-7 expression in early thymic rudiments as early as at embryonic day 13.5 (57) and also in thymic stromal cells. Additionally, IL-7 expression was mapped to lymph nodes, bone marrow, liver, small intestine and skin. Importantly, however, IL-7 expressing cells were completely absent in the spleen.
Durum and colleagues generated an IL-7 reporter mouse, IL7PromECFP, by introducing an enhanced cyan fluorescence protein (ECFP) immediately after the translational initiation sequence of an IL-7 BAC clone (53). Since direct ECFP fluorescence was very weak in these mice, the reporter signal was further enhanced by an anti-ECFP antibody followed by fluorescent secondary antibodies. In two-photon microscopy, however, ECFP signals were sufficiently strong to perform intravital imaging. IL-7 reporter activities were detected in both cortex and medulla of the thymus, and further detailed analysis with antibodies for stromal cell subsets identified Ly51-positive cortical epithelial cells and EpCAM (epithelial cell adhesion molecule)-positive cells, in both the medulla and cortex, as the IL-7 producers. In agreement with the study by Alves et al. (51), fibroblasts, endothelial cells and also dendritic and myeloid derived cells in the thymus did not show IL-7 reporter expression. And as before, no ECFP-positive cells were detected in secondary lymphoid tissues and in no other additional organs including gut, lung and skin. Similarly to the YFP reporter mice (51), the IL7promECFP mouse is likely to report IL-7 expression only in IL-7 high expressing cells, and accordingly, ECFP-positive cells were found in the cervical thymus and also in the bone marrow. Collectively, because of the extremely low level of IL-7 transcription in peripheral tissues, monitoring reporter activity using IL-7 promoter dependent fluorescence signals turned out to be ineffective outside of the thymus. Rather, linking enzymatic activities downstream of IL-7 so that they can either accumulate or be enhanced by secondary reporter activity could provide greater sensitivity in reporting IL-7 expression in vivo.
Schuler and colleagues have employed precisely such an approach when they established a new IL-7 reporter line (54). Here, they subcloned four genes linked to each other as a single reporter construct into an IL-7 BAC transgene. The four reporter genes were interlinked with P2A peptide sequences that allow translation of polycistronic mRNA in mammalian cells (58). This resulted in a transgenic mouse line (IL-7GCDL) that simultaneously expressed enhanced green fluorescent protein (EGFP) (G), Cre recombinase (C), the human diphtheria toxin receptor (D), and click beetle green luciferase 99 (L). Again, the reporter construct replaced the IL-7 ATG initiation site so that BAC-encoded IL-7 expression was abolished. Reporter activity was then determined either by injection of luciferin and measuring in vivo bioluminescence or by anti-GFP antibodies. Similar to ECFP and YFP reporter expression (51,53), peripheral lymphoid tissues expressed GFP mRNA but failed to exhibit any significant amounts of GFP as assessed by flow cytometry or by fluorescence microscopy. These results reaffirm that fluorescence proteins are not optimal reagents for detecting the low promoter activity of IL-7 in vivo. Importantly, in IL-7GCDL mice, even Cre-mediated activation of GFP reporter genes was still insufficient to accurately report IL-7 expression when compared to in vivo bioluminescence assays. Specifically, while luciferase activity was detected in the thymus, skin, small intestine, and to a lower level in lymph nodes, fluorescent protein expression was not detectable in any of these organs except for the thymus. Notably, even with bioluminescence assays, only low or undetectable levels of IL-7 expression were found in the heart, kidney, liver, and spleen (54).
The where and when of IL-7 expression in vivo has been a hotly contended issue as much as the question of whether IL-7 expression is actively regulated or constitutively expressed (49,59). The current series of IL-7 reporter mice are now providing insights into the anatomical distribution and in vivo signals that control IL-7 expression during development and homeostasis. This is especially important since, in a striking demonstration, Durum and colleagues documented that the conventional methods of immunohistochemistry and in situ hybridization are quite inadequate to monitor IL-7 expression in mice (53). Using Cre-recombinase expressing reporter mice that mark cells which have expressed and/or are currently expressing IL-7, and also using fluorescent proteins or luciferase expressing reporter mice that report the actual IL-7 expression levels in situ, there is now an emerging consensus on IL-7 expression in vivo (Table II).
The thymus, because of IL-7 producing thymic stromal cells, is likely to be the organ with the highest level of IL-7 expression. Specifically, Alves et al. (51), describe a distribution of IL-7 producing cells that closely matches observations from Zamisch et al. (57) in that IL-7+ cells are mostly concentrated around the corticomedullary junction of an adult thymus. The phenotypes of IL-7+ cells were heterogeneous in that they bear markers for both cortical and medullary TECs but they lacked markers of hematopoietic cells, endothelial cells and fibroblasts. Mazzucchelli et al. (53) further extended this observation by detailed morphological analysis and found that in the cortex, IL-7+ cells appear to envelop thymocytes in a "basket-like" shape, while in the medulla, an extensive network of processes extended from IL-7+ cells that made contact with thymocytes. Ontogenically, IL-7 reporter expression was first detectable in E12.5 fetal thymuses (60) and fully expressed throughout the thymus at E13.5 (57). Interestingly, IL-7+ TEC frequency steadily declined with increasing numbers of thymocytes and thymic maturation, and was augmented when thymocyte development was intrinsically blocked, suggesting a potential negative feedback of thymocytes on IL-7+ TECs (60). Indeed, IL-7+ TEC frequencies were significantly increased in immunocompromised mice such as in Rag2-/-Il2rg-/- mice whereas bone marrow transfer of either Tcra-/- or WT cells markedly reduced their frequencies. These data suggest that thymocyte-derived signals modulate IL-7 expression by remodeling the thymic stroma, and it uncovers a novel thymocyte-TEC cross-talk mechanism in the development of T cells (60).
Outside of the thymus, identification of IL-7 reporter positive cells remains somewhat controversial. All reporter mice using fluorescent proteins to monitor IL-7 expression fail to detect IL-7 expression in any other peripheral organ than the bone marrow (51,53). Moreover, even mice in which IL-7 expression was mapped by Cre or luciferase activity did not completely agree on the tissue distribution of IL-7 producer cells (52,54). Nevertheless, it seems that every study agrees that IL-7 expression was below detectable levels in spleen and kidney, while the skin and intestine showed strong signals of IL-7 reporter expression. Investigating the role of IL-7 in these organs will be clearly the subject of future studies.
However, harnessing the power of newly generated IL-7 reporter mice, a series of questions have been already addressed. As mentioned above, thymic IL-7 expression was developmentally regulated and not fixed. In fact, frequency of IL-7+ TECs declined with age, and there was a marked reduction of IL-7+ cell frequency in 5~7 weeks aged mice compared to neonatal and 1~2 weeks old mice (51). Furthermore, in addition to IL-7, the thymic stromal lymphopoietin (TSLP) also utilizes the IL-7Rα and STAT5 for signaling in T cells, and TSLP has been proposed to be a partially redundant pro-survival cytokine in immature thymocytes (61). However, using IL-7 reporter mice, IL-7 and TSLP expression was now shown to occupy different cellular and anatomical niches suggesting that IL-7 plays a distinct role to TSLP in thymopoiesis. Such analyses at single cell levels were difficult to do before the availability of IL-7 reporter mice.
Another interesting observation from IL-7 reporter mice is the reduced frequency of IL-7 expressing TECs by TCRβ-selected thymocytes (60). This finding is in agreement with a previous study by Zamisch et al. (57). There, the authors observed that introduction of a TCRβ transgene into RAG2-deficient mice largely restored thymic cellularity and also the generation of DP thymocytes but that it paradoxically resulted in diminished frequencies of IL-7+ TECs. Similarly, IL-7+ TECs are retained in the thymus of mice with profound and early block in thymocyte development (Rag2-/-Il2rg-/-) and reconstitution of alymphoid reporter thymus with Tcra-/- hematopoietic progenitors diminishes the frequency of IL-7+ TECs (60). Together, these data revealed that thymocytes negatively regulate thymic IL-7 production, but the biological significance of this feedback mechanism still needs to be addressed.
With the ability to visualize IL-7 producing cells, it is now possible to track their biological activities in vivo and in real time. A compelling study by Durum and colleagues (53) assessed the interactions of T cells with ECFP+ IL-7 producing stromal cells using intravital microscopy. Central memory T cells (TCM) are known to home to the bone marrow, and it had been suggested that IL-7 signaling by bone marrow resident stromal cells could be involved (62). Indeed, two photon microscopy of CFSE-labeled and intravenously injected TCMs displayed a preferential interaction with IL-7+ producing cells in the bone marrow, but subsequent studies showed that such preferential encounters was independent of IL-7 or the chemokine CXCL12 (53). Thus, while the exact identity of IL-7 producing cells in the bone marrow is unknown and also why TCM preferentially interact with these cells is unclear, these data confirm the power of IL-7-reporter mice in assessing unresolved questions on IL-7 biology in vivo.
Finally, IL-7 expression was shown to be expressed by intestinal epithelial cells (IEC) in the gut (37). IL-7 reporter mice confirmed this observation by displaying strong bioluminescence in the intestine and more specifically in EpCAM+ IECs. Since in vitro data suggested that IL-7 expression in IEC is regulated by IFN-γ (63), IL-7 reporter activity was assessed in Ifng-/- mice and a significant reduction in IL-7 expression was found in the colon (54). Interestingly, intestinal IFN-γ expression itself was dependent on an intact commensal microflora. These observations suggest a novel regulatory mechanism for mucosal IL-7 expression: IFN-γ expression is induced by the microflora in the gut, and IFN-γ directly upregulates IL-7 expression in IEC which then supports the survival of intestinal T cells. These findings provide a powerful clue in understanding the role of the commensal microbiota in the cytokine-mediated homeostasis of the mucosal immune system.
The availability of new IL-7 reporter mice provided new information on the in vivo expression and regulation of IL-7. These studies confirmed thymic stroma as a site of high IL-7 expression levels. These studies also enabled the identification and precise phenotyping of IL-7 producing cells in the thymus as thymic epithelial cells whose development and maintenance were actively regulated during ontogeny and aging. In peripheral tissues, IL-7 producing cells were identified in a number of different tissues including lymph node, skin and intestine, but reporter activity also allowed the exclusion of spleen as an IL-7+ site. Importantly, IL-7 expression by IECs in the intestine was found to be dependent on the commensal microflora and regulated by IFN-γ, which suggest that IL-7 expression can be actively modulated and is locally controlled. The new information gained from these IL-7 reporter mice greatly improved our understanding on IL-7 function by illuminating the source of in vivo IL-7. We anticipate that these new tools will provide us with further insights in future studies.
Figures and Tables
Figure 1
Schematic representation of the BAC constructs for IL-7 reporter mice. Endogenous wildtype IL-7 locus is shown at the top. Alves et al. (51) generated BAC transgenic mice encoding the yellow fluorescent protein (YFP) under the control of the IL-7 promoter. The YFP gene was inserted by homologous recombination downstream of the ATG transcriptional start codon of exon 1 of the IL-7 locus. Repass et al. (52) generated two BAC transgenic mice where IL-7 regulatory elements drive either Cre recombinase (IL-7.Cre) or human CD25 (IL-7.hCD25) reporter expression. Mazzucchelli et al. (53) generated BAC transgenic mice encoding the enhanced cyan fluorescence protein (ECFP) under the control of the IL-7 promoter (IL7promECFP). The exon 1 sequence, encoding the signal peptide was replaced with the Ecfp cDNA sequence starting after the ATG start site. Shalapour et al. (54) generated BAC transgenic mice simultaneously expressing enhanced green fluorescent protein (G), recombinase Cre (C), the human diphtheria toxin receptor (D) and click beetle green luciferase 99 (L) under control of the IL-7 promoter (IL-7GCDL). E1~E5: Exon 1~Exon 5. UTR: Untranslated region.
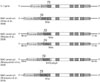
ACKNOWLEDGEMENTS
We thank Drs. James Di Santo and Alfred Singer for helpful discussion and review of the manuscript. This study was supported by the Intramural Research Program of the US National Institutes of Health, National Cancer Institute, Center for Cancer Research.
References
1. Namen AE, Schmierer AE, March CJ, Overell RW, Park LS, Urdal DL, Mochizuki DY. B cell precursor growth-promoting activity. Purification and characterization of a growth factor active on lymphocyte precursors. J Exp Med. 1988. 167:988–1002.


2. Peschon JJ, Morrissey PJ, Grabstein KH, Ramsdell FJ, Maraskovsky E, Gliniak BC, Park LS, Ziegler SF, Williams DE, Ware CB, Meyer JD, Davison BL. Early lymphocyte expansion is severely impaired in interleukin 7 receptor-deficient mice. J Exp Med. 1994. 180:1955–1960.


3. von Freeden-Jeffry U, Vieira P, Lucian LA, McNeil T, Burdach SE, Murray R. Lymphopenia in interleukin (IL)-7 gene-deleted mice identifies IL-7 as a nonredundant cytokine. J Exp Med. 1995. 181:1519–1526.


4. Schluns KS, Kieper WC, Jameson SC, Lefrançois L. Interleukin-7 mediates the homeostasis of naïve and memory CD8 T cells in vivo. Nat Immunol. 2000. 1:426–432.


5. Tan JT, Dudl E, LeRoy E, Murray R, Sprent J, Weinberg KI, Surh CD. IL-7 is critical for homeostatic proliferation and survival of naive T cells. Proc Natl Acad Sci U S A. 2001. 98:8732–8737.


6. Park JH, Adoro S, Guinter T, Erman B, Alag AS, Catalfamo M, Kimura MY, Cui Y, Lucas PJ, Gress RE, Kubo M, Hennighausen L, Feigenbaum L, Singer A. Signaling by intrathymic cytokines, not T cell antigen receptors, specifies CD8 lineage choice and promotes the differentiation of cytotoxic-lineage T cells. Nat Immunol. 2010. 11:257–264.


7. Puel A, Ziegler SF, Buckley RH, Leonard WJ. Defective IL7R expression in T(-)B(+)NK(+) severe combined immunodeficiency. Nat Genet. 1998. 20:394–397.


8. Noguchi M, Yi H, Rosenblatt HM, Filipovich AH, Adelstein S, Modi WS, McBride OW, Leonard WJ. Interleukin-2 receptor gamma chain mutation results in X-linked severe combined immunodeficiency in humans. Cell. 1993. 73:147–157.


9. Witte PL, Burrows PD, Kincade PW, Cooper MD. Characterization of B lymphocyte lineage progenitor cells from mice with severe combined immune deficiency disease (SCID) made possible by long term culture. J Immunol. 1987. 138:2698–2705.
10. Akashi K, Kondo M, von Freeden-Jeffry U, Murray R, Weissman IL. Bcl-2 rescues T lymphopoiesis in interleukin-7 receptor-deficient mice. Cell. 1997. 89:1033–1041.


11. Opferman JT, Letai A, Beard C, Sorcinelli MD, Ong CC, Korsmeyer SJ. Development and maintenance of B and T lymphocytes requires antiapoptotic MCL-1. Nature. 2003. 426:671–676.


12. Wofford JA, Wieman HL, Jacobs SR, Zhao Y, Rathmell JC. IL-7 promotes Glut1 trafficking and glucose uptake via STAT5-mediated activation of Akt to support T-cell survival. Blood. 2008. 111:2101–2111.


13. Vivien L, Benoist C, Mathis D. T lymphocytes need IL-7 but not IL-4 or IL-6 to survive in vivo. Int Immunol. 2001. 13:763–768.


14. Rathmell JC, Farkash EA, Gao W, Thompson CB. IL-7 enhances the survival and maintains the size of naive T cells. J Immunol. 2001. 167:6869–6876.


16. Ku CC, Murakami M, Sakamoto A, Kappler J, Marrack P. Control of homeostasis of CD8+ memory T cells by opposing cytokines. Science. 2000. 288:675–678.


17. Tan JT, Ernst B, Kieper WC, LeRoy E, Sprent J, Surh CD. Interleukin (IL)-15 and IL-7 jointly regulate homeostatic proliferation of memory phenotype CD8+ cells but are not required for memory phenotype CD4+ cells. J Exp Med. 2002. 195:1523–1532.


18. Kieper WC, Tan JT, Bondi-Boyd B, Gapin L, Sprent J, Ceredig R, Surh CD. Overexpression of interleukin (IL)-7 leads to IL-15-independent generation of memory phenotype CD8+ T cells. J Exp Med. 2002. 195:1533–1539.


19. Schluns KS, Williams K, Ma A, Zheng XX, Lefrançois L. Cutting edge: requirement for IL-15 in the generation of primary and memory antigen-specific CD8 T cells. J Immunol. 2002. 168:4827–4831.


20. Rochman Y, Spolski R, Leonard WJ. New insights into the regulation of T cells by gamma(c) family cytokines. Nat Rev Immunol. 2009. 9:480–490.


21. Witthuhn BA, Silvennoinen O, Miura O, Lai KS, Cwik C, Liu ET, Ihle JN. Involvement of the Jak-3 Janus kinase in signalling by interleukins 2 and 4 in lymphoid and myeloid cells. Nature. 1994. 370:153–157.


22. Maraskovsky E, O'Reilly LA, Teepe M, Corcoran LM, Peschon JJ, Strasser A. Bcl-2 can rescue T lymphocyte development in interleukin-7 receptor-deficient mice but not in mutant rag-1-/- mice. Cell. 1997. 89:1011–1019.


23. Chehtane M, Khaled AR. Interleukin-7 mediates glucose utilization in lymphocytes through transcriptional regulation of the hexokinase II gene. Am J Physiol Cell Physiol. 2010. 298:C1560–C1571.


24. Khaled AR, Bulavin DV, Kittipatarin C, Li WQ, Alvarez M, Kim K, Young HA, Fornace AJ, Durum SK. Cytokine-driven cell cycling is mediated through Cdc25A. J Cell Biol. 2005. 169:755–763.


25. Jiang Q, Li WQ, Aiello FB, Mazzucchelli R, Asefa B, Khaled AR, Durum SK. Cell biology of IL-7, a key lymphotrophin. Cytokine Growth Factor Rev. 2005. 16:513–533.


26. Chowdhury D, Sen R. Transient IL-7/IL-7R signaling provides a mechanism for feedback inhibition of immunoglobulin heavy chain gene rearrangements. Immunity. 2003. 18:229–241.


27. Maki K, Sunaga S, Ikuta K. The V-J recombination of T cell receptor-gamma genes is blocked in interleukin-7 receptor-deficient mice. J Exp Med. 1996. 184:2423–2427.
28. Meier D, Bornmann C, Chappaz S, Schmutz S, Otten LA, Ceredig R, Acha-Orbea H, Finke D. Ectopic lymphoid-organ development occurs through interleukin 7-mediated enhanced survival of lymphoid-tissue-inducer cells. Immunity. 2007. 26:643–654.


29. Chappaz S, Finke D. The IL-7 signaling pathway regulates lymph node development independent of peripheral lymphocytes. J Immunol. 2010. 184:3562–3569.


30. Spits H, Di Santo JP. The expanding family of innate lymphoid cells: regulators and effectors of immunity and tissue remodeling. Nat Immunol. 2011. 12:21–27.


31. Park JH, Adoro S, Lucas PJ, Sarafova SD, Alag AS, Doan LL, Erman B, Liu X, Ellmeier W, Bosselut R, Feigenbaum L, Singer A. 'Coreceptor tuning': cytokine signals transcriptionally tailor CD8 coreceptor expression to the self-specificity of the TCR. Nat Immunol. 2007. 8:1049–1059.


32. Park JH, Yu Q, Erman B, Appelbaum JS, Montoya-Durango D, Grimes HL, Singer A. Suppression of IL7Ralpha transcription by IL-7 and other prosurvival cytokines: a novel mechanism for maximizing IL-7-dependent T cell survival. Immunity. 2004. 21:289–302.


33. Guimond M, Fry TJ, Mackall CL. Cytokine signals in T-cell homeostasis. J Immunother. 2005. 28:289–294.


34. Namen AE, Lupton S, Hjerrild K, Wignall J, Mochizuki DY, Schmierer A, Mosley B, March CJ, Urdal D, Gillis S. Stimulation of B-cell progenitors by cloned murine interleukin-7. Nature. 1988. 333:571–573.


35. Goodwin RG, Lupton S, Schmierer A, Hjerrild KJ, Jerzy R, Clevenger W, Gillis S, Cosman D, Namen AE. Human interleukin 7: molecular cloning and growth factor activity on human and murine B-lineage cells. Proc Natl Acad Sci U S A. 1989. 86:302–306.


36. Link A, Vogt TK, Favre S, Britschgi MR, Acha-Orbea H, Hinz B, Cyster JG, Luther SA. Fibroblastic reticular cells in lymph nodes regulate the homeostasis of naive T cells. Nat Immunol. 2007. 8:1255–1265.


37. Watanabe M, Ueno Y, Yajima T, Iwao Y, Tsuchiya M, Ishikawa H, Aiso S, Hibi T, Ishii H. Interleukin 7 is produced by human intestinal epithelial cells and regulates the proliferation of intestinal mucosal lymphocytes. J Clin Invest. 1995. 95:2945–2953.


38. Golden-Mason L, Kelly AM, Traynor O, McEntee G, Kelly J, Hegarty JE, O'Farrelly C. Expression of interleukin 7 (IL-7) mRNA and protein in the normal adult human liver: implications for extrathymic T cell development. Cytokine. 2001. 14:143–151.


39. Heufler C, Topar G, Grasseger A, Stanzl U, Koch F, Romani N, Namen AE, Schuler G. Interleukin 7 is produced by murine and human keratinocytes. J Exp Med. 1993. 178:1109–1114.


40. Matsue H, Bergstresser PR, Takashima A. Keratinocyte-derived IL-7 serves as a growth factor for dendritic epidermal T cells in mice. J Immunol. 1993. 151:6012–6019.
41. Cheng Y, Yata A, Klein C, Cho JH, Deguchi M, Hsueh AJ. Oocyte-Expressed Interleukin 7 Suppresses Granulosa Cell Apoptosis and Promotes Oocyte Maturation in Rats. Biol Reprod. 2010. [Epub ahead of print].


42. Moors M, Vudattu NK, Abel J, Krämer U, Rane L, Ulfig N, Ceccatelli S, Seyfert-Margolies V, Fritsche E, Maeurer MJ. Interleukin-7 (IL-7) and IL-7 splice variants affect differentiation of human neural progenitor cells. Genes Immun. 2010. 11:11–20.


43. Di Carlo E, D'Antuono T, Pompa P, Giuliani R, Rosini S, Stuppia L, Musiani P, Sorrentino C. The lack of epithelial interleukin-7 and BAFF/BLyS gene expression in prostate cancer as a possible mechanism of tumor escape from immunosurveillance. Clin Cancer Res. 2009. 15:2979–2987.


44. Guimond M, Veenstra RG, Grindler DJ, Zhang H, Cui Y, Murphy RD, Kim SY, Na R, Hennighausen L, Kurtulus S, Erman B, Matzinger P, Merchant MS, Mackall CL. Interleukin 7 signaling in dendritic cells regulates the homeostatic proliferation and niche size of CD4+ T cells. Nat Immunol. 2009. 10:149–157.


45. Banwell CM, Partington KM, Jenkinson EJ, Anderson G. Studies on the role of IL-7 presentation by mesenchymal fibroblasts during early thymocyte development. Eur J Immunol. 2000. 30:2125–2129.


46. Lai L, Goldschneider I. Cutting edge: Identification of a hybrid cytokine consisting of IL-7 and the beta-chain of the hepatocyte growth factor/scatter factor. J Immunol. 2001. 167:3550–3554.


47. Fry TJ, Mackall CL. The many faces of IL-7: from lymphopoiesis to peripheral T cell maintenance. J Immunol. 2005. 174:6571–6576.


48. Muthukumar A, Wozniakowski A, Gauduin MC, Paiardini M, McClure HM, Johnson RP, Silvestri G, Sodora DL. Elevated interleukin-7 levels not sufficient to maintain T-cell homeostasis during simian immunodeficiency virus-induced disease progression. Blood. 2004. 103:973–979.


49. Napolitano LA, Grant RM, Deeks SG, Schmidt D, De Rosa SC, Herzenberg LA, Herndier BG, Andersson J, McCune JM. Increased production of IL-7 accompanies HIV-1-mediated T-cell depletion: implications for T-cell homeostasis. Nat Med. 2001. 7:73–79.


50. Sawa Y, Arima Y, Ogura H, Kitabayashi C, Jiang JJ, Fukushima T, Kamimura D, Hirano T, Murakami M. Hepatic interleukin-7 expression regulates T cell responses. Immunity. 2009. 30:447–457.


51. Alves NL, Richard-Le Goff O, Huntington ND, Sousa AP, Ribeiro VS, Bordack A, Vives FL, Peduto L, Chidgey A, Cumano A, Boyd R, Eberl G, Di Santo JP. Characterization of the thymic IL-7 niche in vivo. Proc Natl Acad Sci U S A. 2009. 106:1512–1517.


52. Repass JF, Laurent MN, Carter C, Reizis B, Bedford MT, Cardenas K, Narang P, Coles M, Richie ER. IL7-hCD25 and IL7-Cre BAC transgenic mouse lines: new tools for analysis of IL-7 expressing cells. Genesis. 2009. 47:281–287.


53. Mazzucchelli RI, Warming S, Lawrence SM, Ishii M, Abshari M, Washington AV, Feigenbaum L, Warner AC, Sims DJ, Li WQ, Hixon JA, Gray DH, Rich BE, Morrow M, Anver MR, Cherry J, Naf D, Sternberg LR, McVicar DW, Farr AG, Germain RN, Rogers K, Jenkins NA, Copeland NG, Durum SK. Visualization and identification of IL-7 producing cells in reporter mice. PLoS One. 2009. 4:e7637.


54. Shalapour S, Deiser K, Sercan O, Tuckermann J, Minnich K, Willimsky G, Blankenstein T, Hämmerling GJ, Arnold B, Schüler T. Commensal microflora and interferon-gamma promote steady-state interleukin-7 production in vivo. Eur J Immunol. 2010. 40:2391–2400.


55. Soriano P. Generalized lacZ expression with the ROSA26 Cre reporter strain. Nat Genet. 1999. 21:70–71.


56. Srinivas S, Watanabe T, Lin CS, William CM, Tanabe Y, Jessell TM, Costantini F. Cre reporter strains produced by targeted insertion of EYFP and ECFP into the ROSA26 locus. BMC Dev Biol. 2001. 1:4.
57. Zamisch M, Moore-Scott B, Su DM, Lucas PJ, Manley N, Richie ER. Ontogeny and regulation of IL-7-expressing thymic epithelial cells. J Immunol. 2005. 174:60–67.


58. Szymczak AL, Workman CJ, Wang Y, Vignali KM, Dilioglou S, Vanin EF, Vignali DA. Correction of multi-gene deficiency in vivo using a single 'self-cleaving' 2A peptide-based retroviral vector. Nat Biotechnol. 2004. 22:589–594.


59. Fry TJ, Mackall CL. Interleukin-7: master regulator of peripheral T-cell homeostasis? Trends Immunol. 2001. 22:564–571.


60. Alves NL, Huntington ND, Mention JJ, Richard-Le Goff O, Di Santo JP. Cutting Edge: a thymocyte-thymic epithelial cell cross-talk dynamically regulates intrathymic IL-7 expression in vivo. J Immunol. 2010. 184:5949–5953.


61. Chappaz S, Flueck L, Farr AG, Rolink AG, Finke D. Increased TSLP availability restores T- and B-cell compartments in adult IL-7 deficient mice. Blood. 2007. 110:3862–3870.


62. Mazo IB, Honczarenko M, Leung H, Cavanagh LL, Bonasio R, Weninger W, Engelke K, Xia L, McEver RP, Koni PA, Silberstein LE, von Andrian UH. Bone marrow is a major reservoir and site of recruitment for central memory CD8+ T cells. Immunity. 2005. 22:259–270.


63. Oshima S, Nakamura T, Namiki S, Okada E, Tsuchiya K, Okamoto R, Yamazaki M, Yokota T, Aida M, Yamaguchi Y, Kanai T, Handa H, Watanabe M. Interferon regulatory factor 1 (IRF-1) and IRF-2 distinctively up-regulate gene expression and production of interleukin-7 in human intestinal epithelial cells. Mol Cell Biol. 2004. 24:6298–6310.

