Abstract
Fibroblast growth factor 21 (FGF21) is an endocrine hormone that is primarily expressed in the liver and exerts beneficial effects on obesity and related metabolic diseases. In addition to its remarkable pharmacologic actions, the physiological roles of FGF21 include the maintenance of energy homeostasis in the body in conditions of metabolic or environmental stress. The expression of FGF21 is induced in multiple organs in response to diverse physiological or pathological stressors, such as starvation, nutrient excess, autophagy deficiency, mitochondrial stress, exercise, and cold exposure. Thus, the FGF21 induction caused by stress plays an important role in adaptive response to these stimuli. Here, we highlight our current understanding of the functional importance of the induction of FGF21 by diverse stressors as a feedback mechanism that prevents excessive stress.
Fibroblast growth factor 21 (FGF21) was identified and cloned as the 21st member of the FGF family [1]. The biological function of FGF21 as a novel metabolic regulator was first reported by researchers from the Lilly Research Laboratories [2]. These researchers discovered that FGF21 is a secretory protein that enhances glucose uptake in adipocytes and has beneficial metabolic effects on insulin resistance and diabetes [2]. Specifically, transgenic mice overexpressing FGF21 exhibit resistance to diet-induced obesity, which indicates the potential of FGF21 as an antiobesity molecule [2]. In line with this report, subsequent studies have suggested that FGF21 and FGF21 mimetics can improve the metabolic parameters of obese diabetic rodents [3,4,5], rhesus monkeys [6,7] and human subjects [8]. Such therapeutic effects of FGF21 have been attributed to the pleiotropic metabolic actions of FGF21 in multiple target organs such as the adipose tissue [9,10,11], liver [4,12], pancreas [13], and hypothalamus [14,15]. Intriguingly, emerging studies have suggested the potential role of FGF21 as a regulator that mediates the therapeutic effects of several antidiabetic drugs or compounds, such as thiazolidinedione [16], glucagon analogue [17], glucagon-like peptide-1 analogue [18], and metformin [19]. In addition to the beneficial pharmacological effects of exogenous FGF21, endogenous FGF21 also plays an important role in the maintenance of energy homeostasis in several stressful conditions, such as nutrient starvation [20,21] and cold exposure [22,23]. Furthermore, a growing body of research suggests that FGF21 is also able to exert protective functions in pathological conditions that occurring after the administration of chemicals, such as acetaminophen-induced liver toxicity [24], dioxin-induced toxicity [25], cerulein-induced pancreatitis [26], and phenylephrine-induced cardiac hypertrophic damage [27]. These findings suggest that FGF21 acts as a key regulator in the adaptation to stress and can limit the progression of stress in diverse disease conditions. Among the numerous actions of FGF21, we highlight our recent understanding of FGF21 as a stress hormone in the present review and focus on the functional significance and molecular mechanisms of the induction of FGF21 in response to diverse stressors such as nutrient deprivation or overload, autophagy deficiency, mitochondrial stress, exercise, and cold exposure (Fig. 1).
Several previous studies have suggested that FGF21 expression is regulated by various nutrient stresses such as starvation [20,21,28], a ketogenic diet [29], amino acid deprivation [30,31], undernutrition (or malnutrition) [32], and a high-fat diet or obesity [33,34]. Consequently, increased FGF21 levels might play a role in the adaptation to nutritional stress.
The physiological function of FGF21 in the maintenance of nutritional homeostasis was suggested in papers that showed that FGF21 is a molecule regulating lipid metabolism in response to fasting [20,21]. In starvation, FGF21 expression has been reported to be induced in the liver via the peroxisome proliferator-activated receptor α (PPARα). Additionally, recent studies have suggested that the cAMP-responsive element binding protein H and sirtuin 1 also participate in fasting-induced FGF21 expression [28,35]. Subsequently, increased FGF21 expression promotes lipolysis in adipose tissue, and the fatty acids released from adipose tissue are transported to the liver where they are directly oxidized for energy production or utilized as a source for ketone body formation. Furthermore, hepatic FGF21 induction contributes to the alleviation of fasting-induced hepatosteatosis by enhancing the expression of the genes involved in fatty acid oxidation [28]. In addition to fasting-induced changes in lipid metabolism, PPARα-mediated FGF21 induction is also involved in the increases in fatty acid oxidation and ketogenesis that result from ketogenic diets [29]. These results indicate that FGF21 acts as a critical regulator in the metabolic adaptation to fasting or ketotic states.
In addition to fasting, FGF21 has been reported to be important in the regulation of lipid metabolism in response to amino acid deficiency [30,31]. In mice fed leucine-deficient diets, FGF21 expression increases in the liver likely through the activation of a transcription factor 4 (ATF4)-dependent mechanism, but no differences in the expression of FGF21 in leucine deficient mice and control mice occur in other metabolic organs, including adipose tissue and skeletal muscle [30,36]. Importantly, mice that are fed a leucine-deficient diet exhibit reduced body weight and fat mass compared to mice that are fed a control diet [30,36]. Importantly, such changes in body weight and fat mass due to a leucine-deficient diet are significantly attenuated in FGF21-knockout mice. These results have been attributed to the absence of the action of FGF21, which leads to the suppression of lipolysis and the enhancement of lipogenesis in adipose tissue in the leucine-deficient condition. Taken together, these findings suggest that hepatic FGF21 induction by amino acid deprivation influences lipid metabolism in adipose tissues.
FGF21 has also been reported to affect the changes in skeletal metabolism that are caused by undernutrition (or malnutrition) [32]. It is well known that an insufficient supply of nutrients impedes bone growth. In nutrient-deficient conditions, FGF21-knockout mice exhibit less inhibition of skeletal growth than do control mice [32], which indicates that FGF21 has a causal role in the undernutrition-related reduction in bone growth. FGF21-induced bone loss is probably due to the direct suppression of osteoblastogenesis and chondrogenesis [32,37,38]. Thus, these results demonstrate the functional significance of FGF21 as a key regulator of skeletal homeostasis as well as liver and adipose tissue homeostasis in nutritionally stressful states. In contrast to malnutrition, caloric restriction alone does not cause the induction of FGF21 in mice [30,39]. Furthermore, we did not observe differences in the metabolic parameters between FGF21-knockout and control mice after feeding them calorie-restricted diets [30], which indicates that the beneficial metabolic effects of caloric restriction are unrelated to the induction of FGF21. Thus, we speculate that FGF21 might play an important role in the metabolic response to undernutrition but not in the response to caloric restriction.
Nutrient overload is also capable of influencing FGF21 expression. Specifically, serum FGF21 levels increase in obese human subjects or mice following the consumption of a high-fat diet [33,34]. However, FGF21 signaling is impaired in the liver and white adipose tissue (WAT) of obese insulin-resistant mice [34] and in the pancreas islets of obese diabetic mice [40], which suggests that obesity might be a state of FGF21 resistance. However, the administration of exogenous FGF21 overcomes this resistance and leads to improvements in obesity-related metabolic deterioration. Additionally, the functional importance of endogenous FGF21 induction in the development and progression of obesity-related insulin resistance was recently evaluated using FGF21-knockout mice that were generated independently by three groups. In one study, the FGF21-knockout mice exhibited exacerbated glucose intolerance without changes in body weight compared to the control mice on high-fat diets [16]. In contrast, FGF21-knockout mice on high-fat diets were found to have body weights and glucose intolerance levels that were similar to those of control mice in another study [34]. Intriguingly, the same knockout strain that was employed in the second study exhibited increased body weight compared to control mice despite exhibiting similar glucose intolerance levels after consuming a high-fat diet when the mice were maintained in another facility [41]. However, in our experiments that employed a different FGF21-knockout strain [42], no significant differences in both body weight or glucose intolerance were observed between the FGF21-knockout mice and controls that were fed a high-fat diet. These discrepancies in the metabolic phenotypes of the three FGF21-knockout lines are probably attributable to differences in several factors such as diet composition, animal core facility, age, breeding strategy, and genetic background. Therefore, the fundamental role of the endogenous FGF21 that is induced by obesity remains controversial and to be determined, but the metabolically beneficial effects of exogenous FGF21 are irrefutable.
Autophagy is a lysosomal catabolic process of degradation of subcellular materials that are surrounded by double-membrane structures called autophagosomes. Autophagy plays an important role in the maintenance of energy balance by degrading or recycling cellular constituents and also plays an important role in cellular quality control via the turnover of damaged organelles and in the removal of aggregated proteins [43]. Thus, the impairment of autophagy causes the accumulation of dysfunctional mitochondria and an increase in the lipid contents of the affected organs; these effects might lead to the development of insulin resistance. Contrary to this expectation, it has been reported that mice with autophagy deficiencies in the liver or skeletal muscle are resistant to obesity-induced insulin resistance; this effect has been attributed to the induction of FGF21 in the autophagy-deficient insulin target tissues and the consequent leanness [30]. Mitochondrial dysfunction due to autophagy deficiency appears to be the cause of these metabolic changes and FGF21 induction, which is dependent on the eukaryotic translation factor 2α (eIF2α)-ATF4 axis [30]. The effects of mitochondrial stress on FGF21 induction have also been observed in other reports that observed increased FGF21 levels in patients with mutations of mitochondrial DNA and in mice with mitochondrial myopathy [44,45]. Thus, FGF21 could be a biomarker of human mitochondrial disorders. The induction of FGF21 by metformin might also be due to mitochondrial stress [19] because mitochondrial complex I activity is inhibited by metformin [46]. Thus, we speculate that FGF21 plays a role as an adaptive regulator that counteracts the metabolic stress imposed by autophagy deficiency or by mitochondrial dysfunction. Furthermore, given the evidence of the direct effects of FGF21 on the enhancement of mitochondrial function or capacity [47], we hypothesize that FGF21 induction by mitochondrial stress can serve as a compensatory mechanism that alleviates mitochondrial dysfunction. Further studies are necessary to validate this hypothesis and to evaluate the role of endogenous FGF21 in the pathogenesis of diseases that are related to mitochondrial dysfunction.
Exercise can ameliorate the severity of obesity and its metabolic complications by increasing energy expenditure [48]. Specifically, exercise increases the levels of circulating proteins called myokines that are released from skeletal muscle and mediate the metabolically beneficial effects of exercise [49]. The expressions of FGF21 in skeletal muscle and in the liver have been reported, which indicates that FGF21 might act as a myokine [50]. Given that FGF21 exerts its beneficial effects by reducing fat mass and enhancing energy expenditure [3], its beneficial metabolic effect are similar to those of exercise. Thus, it has been speculated that exercise might influence circulating FGF21 levels and that exercise-induced metabolic improvements might involve the induction of FGF21. Accordingly, it has recently been shown that acute exercise increases circulating FGF21 levels in humans and mice [51]. However, the induction of FGF21 following exercise has been observed in the livers of mice but not in the skeletal muscle, which suggests that the FGF21 released from the liver contributes to the increased serum FGF21 levels [51]. In addition to the effect of acute exercise on FGF21 induction, chronic exercise has been reported to induce FGF21 expression in human individuals [52]. Although conflicting effects of exercise on FGF21 induction have also been reported [23,53], these results suggest that FGF21 induction might contribute to the beneficial metabolic effects of exercise. Further studies are warranted to determine the functional relevance of FGF21 as a potent regulator that mediates the beneficial effects of exercise on obesity-related metabolic complications.
Cold exposure promotes heat production for the maintenance of body temperature. In addition an increase in thermogenesis in brown adipose tissue (BAT), cold exposure has been reported to stimulate the conversion of WAT to BAT-like tissue in a process called 'browning' [22,54]. BAT-like adipocytes known as 'brite' or 'beige' cells are capable of expressing high levels of uncoupling protein 1 in response to cold exposure, which contributes to heat production and the maintenance of body temperature at the expense of ATP generation [55]. Importantly, emerging studies have suggested that FGF21 expression is increased by cold stimuli in BAT and WAT with thermogenic potential, such as subcutaneous fat, but not in visceral fat [22,56]. FGF21-knockout mice exhibit reduced core body temperatures during cold exposure compared to control mice, which is probably due to an impairment in thermogenesis via the 'browning' effect [22]. Furthermore, humans with relatively abundant BAT exhibit increased FGF21 levels following exposure to cold environments compared to individuals with relative BAT paucities [23]. Together, these results suggest that FGF21 plays a key role in the regulation of adaptive thermogenesis by brown fat or beige fat in response to cold exposure.
Numerous studies have suggested that FGF21 is promising therapeutic target for the treatment of insulin resistance and obesity. These beneficial effects of FGF21 are mediated by its multiple actions, including enhancing lipolysis and β-oxidation, browning of WAT, increasing glucose uptake and promoting insulin release. Additionally, an emerging body of research suggests that FGF21 is a key mediator in the adaptations to changes in energy homeostasis that are caused by several nutritional or environmental stressors (Fig. 1). Thus, further studies on the fundamental role of FGF21 in adaptive metabolic changes that occur in response to diverse environmental stressors are needed to evaluate the potential of FGF21 as a therapeutic target for the treatment of human diseases that are characterized by chronic stress, such as insulin resistance and obesity.
Figures and Tables
Fig. 1
The functional role of fibroblast growth factor 21 (FGF21) induction due to diverse stressors. FGF21 expression is increased in multiple major metabolic organs, including the liver, skeletal muscle, white adipose tissue and brown adipose tissue (as illustrated in the middle column of the figure from the above), in response to diverse stressors. Consequently, elevated FGF21 induces various metabolic effects on the major metabolic organs which help adapt to these stressors. mtDNA, mitochondrial DNA.
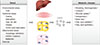
ACKNOWLEDGMENTS
Related work in the authors' laboratory is funded by the Basic Science Research Program through the National Research Foundation of Korea (2013R1A6A3A04065825 to KHK) and the Global Research Laboratory Grant of the National Research Foundation of Korea (K21004000003-10A0500-00310 to MSL).
References
1. Nishimura T, Nakatake Y, Konishi M, Itoh N. Identification of a novel FGF, FGF-21, preferentially expressed in the liver. Biochim Biophys Acta. 2000; 1492:203–206.
2. Kharitonenkov A, Shiyanova TL, Koester A, Ford AM, Micanovic R, Galbreath EJ, Sandusky GE, Hammond LJ, Moyers JS, Owens RA, Gromada J, Brozinick JT, Hawkins ED, Wroblewski VJ, Li DS, Mehrbod F, Jaskunas SR, Shanafelt AB. FGF-21 as a novel metabolic regulator. J Clin Invest. 2005; 115:1627–1635.
3. Coskun T, Bina HA, Schneider MA, Dunbar JD, Hu CC, Chen Y, Moller DE, Kharitonenkov A. Fibroblast growth factor 21 corrects obesity in mice. Endocrinology. 2008; 149:6018–6027.
4. Xu J, Lloyd DJ, Hale C, Stanislaus S, Chen M, Sivits G, Vonderfecht S, Hecht R, Li YS, Lindberg RA, Chen JL, Jung DY, Zhang Z, Ko HJ, Kim JK, Veniant MM. Fibroblast growth factor 21 reverses hepatic steatosis, increases energy expenditure, and improves insulin sensitivity in diet-induced obese mice. Diabetes. 2009; 58:250–259.
5. Wu AL, Kolumam G, Stawicki S, Chen Y, Li J, Zavala-Solorio J, Phamluong K, Feng B, Li L, Marsters S, Kates L, van Bruggen N, Leabman M, Wong A, West D, Stern H, Luis E, Kim HS, Yansura D, Peterson AS, Filvaroff E, Wu Y, Sonoda J. Amelioration of type 2 diabetes by antibody-mediated activation of fibroblast growth factor receptor 1. Sci Transl Med. 2011; 3:113ra126.
6. Kharitonenkov A, Wroblewski VJ, Koester A, Chen YF, Clutinger CK, Tigno XT, Hansen BC, Shanafelt AB, Etgen GJ. The metabolic state of diabetic monkeys is regulated by fibroblast growth factor-21. Endocrinology. 2007; 148:774–781.
7. Foltz IN, Hu S, King C, Wu X, Yang C, Wang W, Weiszmann J, Stevens J, Chen JS, Nuanmanee N, Gupte J, Komorowski R, Sekirov L, Hager T, Arora T, Ge H, Baribault H, Wang F, Sheng J, Karow M, Wang M, Luo Y, McKeehan W, Wang Z, Veniant MM, Li Y. Treating diabetes and obesity with an FGF21-mimetic antibody activating the betaKlotho/FGFR1c receptor complex. Sci Transl Med. 2012; 4:162ra153.
8. Gaich G, Chien JY, Fu H, Glass LC, Deeg MA, Holland WL, Kharitonenkov A, Bumol T, Schilske HK, Moller DE. The effects of LY2405319, an FGF21 analog, in obese human subjects with type 2 diabetes. Cell Metab. 2013; 18:333–340.
9. Ding X, Boney-Montoya J, Owen BM, Bookout AL, Coate KC, Mangelsdorf DJ, Kliewer SA. βKlotho is required for fibroblast growth factor 21 effects on growth and metabolism. Cell Metab. 2012; 16:387–393.
10. Lin Z, Tian H, Lam KS, Lin S, Hoo RC, Konishi M, Itoh N, Wang Y, Bornstein SR, Xu A, Li X. Adiponectin mediates the metabolic effects of FGF21 on glucose homeostasis and insulin sensitivity in mice. Cell Metab. 2013; 17:779–789.
11. Holland WL, Adams AC, Brozinick JT, Bui HH, Miyauchi Y, Kusminski CM, Bauer SM, Wade M, Singhal E, Cheng CC, Volk K, Kuo MS, Gordillo R, Kharitonenkov A, Scherer PE. An FGF21-adiponectin-ceramide axis controls energy expenditure and insulin action in mice. Cell Metab. 2013; 17:790–797.
12. Xu J, Stanislaus S, Chinookoswong N, Lau YY, Hager T, Patel J, Ge H, Weiszmann J, Lu SC, Graham M, Busby J, Hecht R, Li YS, Li Y, Lindberg R, Veniant MM. Acute glucose-lowering and insulin-sensitizing action of FGF21 in insulin-resistant mouse models: association with liver and adipose tissue effects. Am J Physiol Endocrinol Metab. 2009; 297:E1105–E1114.
13. Wente W, Efanov AM, Brenner M, Kharitonenkov A, Koster A, Sandusky GE, Sewing S, Treinies I, Zitzer H, Gromada J. Fibroblast growth factor-21 improves pancreatic beta-cell function and survival by activation of extracellular signal-regulated kinase 1/2 and Akt signaling pathways. Diabetes. 2006; 55:2470–2478.
14. Sarruf DA, Thaler JP, Morton GJ, German J, Fischer JD, Ogimoto K, Schwartz MW. Fibroblast growth factor 21 action in the brain increases energy expenditure and insulin sensitivity in obese rats. Diabetes. 2010; 59:1817–1824.
15. Bookout AL, de Groot MH, Owen BM, Lee S, Gautron L, Lawrence HL, Ding X, Elmquist JK, Takahashi JS, Mangelsdorf DJ, Kliewer SA. FGF21 regulates metabolism and circadian behavior by acting on the nervous system. Nat Med. 2013; 19:1147–1152.
16. Dutchak PA, Katafuchi T, Bookout AL, Choi JH, Yu RT, Mangelsdorf DJ, Kliewer SA. Fibroblast growth factor-21 regulates PPARgamma activity and the antidiabetic actions of thiazolidinediones. Cell. 2012; 148:556–567.
17. Habegger KM, Stemmer K, Cheng C, Muller TD, Heppner KM, Ottaway N, Holland J, Hembree JL, Smiley D, Gelfanov V, Krishna R, Arafat AM, Konkar A, Belli S, Kapps M, Woods SC, Hofmann SM, D'Alessio D, Pfluger PT, Perez-Tilve D, Seeley RJ, Konishi M, Itoh N, Kharitonenkov A, Spranger J, DiMarchi RD, Tschop MH. Fibroblast growth factor 21 mediates specific glucagon actions. Diabetes. 2013; 62:1453–1463.
18. Yang M, Zhang L, Wang C, Liu H, Boden G, Yang G, Li L. Liraglutide increases FGF-21 activity and insulin sensitivity in high fat diet and adiponectin knockdown induced insulin resistance. PLoS One. 2012; 7:e48392.
19. Kim KH, Jeong YT, Kim SH, Jung HS, Park KS, Lee HY, Lee MS. Metformin-induced inhibition of the mitochondrial respiratory chain increases FGF21 expression via ATF4 activation. Biochem Biophys Res Commun. 2013; 440:76–81.
20. Inagaki T, Dutchak P, Zhao G, Ding X, Gautron L, Parameswara V, Li Y, Goetz R, Mohammadi M, Esser V, Elmquist JK, Gerard RD, Burgess SC, Hammer RE, Mangelsdorf DJ, Kliewer SA. Endocrine regulation of the fasting response by PPARalpha-mediated induction of fibroblast growth factor 21. Cell Metab. 2007; 5:415–425.
21. Galman C, Lundasen T, Kharitonenkov A, Bina HA, Eriksson M, Hafstrom I, Dahlin M, Amark P, Angelin B, Rudling M. The circulating metabolic regulator FGF21 is induced by prolonged fasting and PPARalpha activation in man. Cell Metab. 2008; 8:169–174.
22. Fisher FM, Kleiner S, Douris N, Fox EC, Mepani RJ, Verdeguer F, Wu J, Kharitonenkov A, Flier JS, Maratos-Flier E, Spiegelman BM. FGF21 regulates PGC-1alpha and browning of white adipose tissues in adaptive thermogenesis. Genes Dev. 2012; 26:271–281.
23. Lee P, Linderman JD, Smith S, Brychta RJ, Wang J, Idelson C, Perron RM, Werner CD, Phan GQ, Kammula US, Kebebew E, Pacak K, Chen KY, Celi FS. Irisin and FGF21 are cold-induced endocrine activators of brown fat function in humans. Cell Metab. 2014; 19:302–309.
24. Ye D, Wang Y, Li H, Jia W, Man K, Lo CM, Wang Y, Lam KS, Xu A. FGF21 protects against acetaminophen-induced hepatotoxicity by potentiating PGC-1alpha-mediated antioxidant capacity in mice. Hepatology. 2014; 02. 06. DOI: http://dx.doi.org/10.1002/hep.27060.
25. Cheng X, Vispute SG, Liu J, Cheng C, Kharitonenkov A, Klaassen CD. Fibroblast growth factor (Fgf) 21 is a novel target gene of the aryl hydrocarbon receptor (AhR). Toxicol Appl Pharmacol. 2014; 278:65–71.
26. Johnson CL, Weston JY, Chadi SA, Fazio EN, Huff MW, Kharitonenkov A, Koester A, Pin CL. Fibroblast growth factor 21 reduces the severity of cerulein-induced pancreatitis in mice. Gastroenterology. 2009; 137:1795–1804.
27. Planavila A, Redondo I, Hondares E, Vinciguerra M, Munts C, Iglesias R, Gabrielli LA, Sitges M, Giralt M, van Bilsen M, Villarroya F. Fibroblast growth factor 21 protects against cardiac hypertrophy in mice. Nat Commun. 2013; 4:2019.
28. Li Y, Wong K, Giles A, Jiang J, Lee JW, Adams AC, Kharitonenkov A, Yang Q, Gao B, Guarente L, Zang M. Hepatic SIRT1 attenuates hepatic steatosis and controls energy balance in mice by inducing fibroblast growth factor 21. Gastroenterology. 2014; 146:539–549.e7.
29. Badman MK, Pissios P, Kennedy AR, Koukos G, Flier JS, Maratos-Flier E. Hepatic fibroblast growth factor 21 is regulated by PPARalpha and is a key mediator of hepatic lipid metabolism in ketotic states. Cell Metab. 2007; 5:426–437.
30. Kim KH, Jeong YT, Oh H, Kim SH, Cho JM, Kim YN, Kim SS, Kim do H, Hur KY, Kim HK, Ko T, Han J, Kim HL, Kim J, Back SH, Komatsu M, Chen H, Chan DC, Konishi M, Itoh N, Choi CS, Lee MS. Autophagy deficiency leads to protection from obesity and insulin resistance by inducing Fgf21 as a mitokine. Nat Med. 2013; 19:83–92.
31. De Sousa-Coelho AL, Marrero PF, Haro D. Activating transcription factor 4-dependent induction of FGF21 during amino acid deprivation. Biochem J. 2012; 443:165–171.
32. Kubicky RA, Wu S, Kharitonenkov A, De Luca F. Role of fibroblast growth factor 21 (FGF21) in undernutrition-related attenuation of growth in mice. Endocrinology. 2012; 153:2287–2295.
33. Zhang X, Yeung DC, Karpisek M, Stejskal D, Zhou ZG, Liu F, Wong RL, Chow WS, Tso AW, Lam KS, Xu A. Serum FGF21 levels are increased in obesity and are independently associated with the metabolic syndrome in humans. Diabetes. 2008; 57:1246–1253.
34. Fisher FM, Chui PC, Antonellis PJ, Bina HA, Kharitonenkov A, Flier JS, Maratos-Flier E. Obesity is a fibroblast growth factor 21 (FGF21)-resistant state. Diabetes. 2010; 59:2781–2789.
35. Kim H, Mendez R, Zheng Z, Chang L, Cai J, Zhang R, Zhang K. Liver-enriched transcription factor CREBH interacts with peroxisome proliferator-activated receptor alpha to regulate metabolic hormone FGF21. Endocrinology. 2014; 155:769–782.
36. De Sousa-Coelho AL, Relat J, Hondares E, Perez-Marti A, Ribas F, Villarroya F, Marrero PF, Haro D. FGF21 mediates the lipid metabolism response to amino acid starvation. J Lipid Res. 2013; 54:1786–1797.
37. Wei W, Dutchak PA, Wang X, Ding X, Wang X, Bookout AL, Goetz R, Mohammadi M, Gerard RD, Dechow PC, Mangelsdorf DJ, Kliewer SA, Wan Y. Fibroblast growth factor 21 promotes bone loss by potentiating the effects of peroxisome proliferator-activated receptor gamma. Proc Natl Acad Sci U S A. 2012; 109:3143–3148.
38. Wu S, Levenson A, Kharitonenkov A, De Luca F. Fibroblast growth factor 21 (FGF21) inhibits chondrocyte function and growth hormone action directly at the growth plate. J Biol Chem. 2012; 287:26060–26067.
39. Zhang Y, Xie Y, Berglund ED, Coate KC, He TT, Katafuchi T, Xiao G, Potthoff MJ, Wei W, Wan Y, Yu RT, Evans RM, Kliewer SA, Mangelsdorf DJ. The starvation hormone, fibroblast growth factor-21, extends lifespan in mice. Elife. 2012; 1:e00065.
40. So WY, Cheng Q, Chen L, Evans-Molina C, Xu A, Lam KS, Leung PS. High glucose represses beta-klotho expression and impairs fibroblast growth factor 21 action in mouse pancreatic islets: involvement of peroxisome proliferator-activated receptor gamma signaling. Diabetes. 2013; 62:3751–3759.
41. Adams AC, Coskun T, Cheng CC, LS OF, Dubois SL, Kharitonenkov A. Fibroblast growth factor 21 is not required for the antidiabetic actions of the thiazoladinediones. Mol Metab. 2013; 2:205–214.
42. Hotta Y, Nakamura H, Konishi M, Murata Y, Takagi H, Matsumura S, Inoue K, Fushiki T, Itoh N. Fibroblast growth factor 21 regulates lipolysis in white adipose tissue but is not required for ketogenesis and triglyceride clearance in liver. Endocrinology. 2009; 150:4625–4633.
43. Mizushima N, Komatsu M. Autophagy: renovation of cells and tissues. Cell. 2011; 147:728–741.
44. Tyynismaa H, Carroll CJ, Raimundo N, Ahola-Erkkila S, Wenz T, Ruhanen H, Guse K, Hemminki A, Peltola-Mjosund KE, Tulkki V, Oresic M, Moraes CT, Pietilainen K, Hovatta I, Suomalainen A. Mitochondrial myopathy induces a starvation-like response. Hum Mol Genet. 2010; 19:3948–3958.
45. Suomalainen A, Elo JM, Pietilainen KH, Hakonen AH, Sevastianova K, Korpela M, Isohanni P, Marjavaara SK, Tyni T, Kiuru-Enari S, Pihko H, Darin N, Ounap K, Kluijtmans LA, Paetau A, Buzkova J, Bindoff LA, Annunen-Rasila J, Uusimaa J, Rissanen A, Yki-Jarvinen H, Hirano M, Tulinius M, Smeitink J, Tyynismaa H. FGF-21 as a biomarker for muscle-manifesting mitochondrial respiratory chain deficiencies: a diagnostic study. Lancet Neurol. 2011; 10:806–818.
46. Owen MR, Doran E, Halestrap AP. Evidence that metformin exerts its anti-diabetic effects through inhibition of complex 1 of the mitochondrial respiratory chain. Biochem J. 2000; 348(Pt 3):607–614.
47. Chau MD, Gao J, Yang Q, Wu Z, Gromada J. Fibroblast growth factor 21 regulates energy metabolism by activating the AMPK-SIRT1-PGC-1alpha pathway. Proc Natl Acad Sci U S A. 2010; 107:12553–12558.
48. Strasser B. Physical activity in obesity and metabolic syndrome. Ann N Y Acad Sci. 2013; 1281:141–159.
49. Pedersen BK, Febbraio MA. Muscles, exercise and obesity: skeletal muscle as a secretory organ. Nat Rev Endocrinol. 2012; 8:457–465.
50. Izumiya Y, Bina HA, Ouchi N, Akasaki Y, Kharitonenkov A, Walsh K. FGF21 is an Akt-regulated myokine. FEBS Lett. 2008; 582:3805–3810.
51. Kim KH, Kim SH, Min YK, Yang HM, Lee JB, Lee MS. Acute exercise induces FGF21 expression in mice and in healthy humans. PLoS One. 2013; 8:e63517.
52. Cuevas-Ramos D, Almeda-Valdes P, Meza-Arana CE, Brito-Cordova G, Gomez-Perez FJ, Mehta R, Oseguera-Moguel J, Aguilar-Salinas CA. Exercise increases serum fibroblast growth factor 21 (FGF21) levels. PLoS One. 2012; 7:e38022.
53. Fletcher JA, Meers GM, Laughlin MH, Ibdah JA, Thyfault JP, Rector RS. Modulating fibroblast growth factor 21 in hyperphagic OLETF rats with daily exercise and caloric restriction. Appl Physiol Nutr Metab. 2012; 37:1054–1062.
54. Cousin B, Cinti S, Morroni M, Raimbault S, Ricquier D, Penicaud L, Casteilla L. Occurrence of brown adipocytes in rat white adipose tissue: molecular and morphological characterization. J Cell Sci. 1992; 103(Pt 4):931–942.
55. Bartelt A, Heeren J. Adipose tissue browning and metabolic health. Nat Rev Endocrinol. 2014; 10:24–36.
56. Hondares E, Iglesias R, Giralt A, Gonzalez FJ, Giralt M, Mampel T, Villarroya F. Thermogenic activation induces FGF21 expression and release in brown adipose tissue. J Biol Chem. 2011; 286:12983–12990.