Abstract
The capacity to perform certain activities is frequently compromised after total knee arthroplasty (TKA) due to a functional decline resulting from decreased range of motion and a diminished ability to kneel. In this manuscript, the current biomechanical understanding of hyperflexion and kneeling before and after TKA will be discussed. Patellofemoral and tibiofemoral joint contact area, contact pressure, and kinematics were evaluated in cadaveric studies using a Tekscan pressure measuring system and Microscribe. Testing was performed on intact knees and following cruciate retaining and posterior stabilized TKA at knee flexion angles of 90°, 105°, 120°, and 135°. Three loading conditions were used to simulate squatting, double stance kneeling, and single stance kneeling. Following TKA with double stance kneeling, patellofemoral contact areas did not increase significantly at high knee flexion angle (135°). Kneeling resulted in tibial posterior translation and external rotation at all flexion angles. Moving from double to single stance kneeling tended to increase pressures in the cruciate retaining group, but decreased pressures in the posterior stabilized group. The cruciate retaining group had significantly larger contact areas than the posterior stabilized group, although no significant differences in pressures were observed comparing the two TKA designs (p < 0.05). If greater than 120° of postoperative knee range of motion can be achieved following TKA, then kneeling may be performed with less risk in the patellofemoral joint than was previously believed to be the case. However, kneeling may increase the likelihood of damage to cartilage and menisci in intact knees and after TKA increases in tibiofemoral contact area and pressures may lead to polyethyelene wear if performed on a chronic, repetitive basis.
Degenerative joint disease in the knee is a common and significant problem for the elderly active patient population. In advanced degenerative joint disease, total knee arthroplasty (TKA) is a common procedure to relieve pain and restore function. Approximately 450,000 TKAs are performed annually in the United States alone and the number is increasing primarily due to the aging population and longer life expectancies. The expectation today by the patients who receive TKA is to return to their previous lifestyles and occupations. However, the capacity to perform certain activities is frequently compromised after TKA due to a functional decline resulting from decreased range of motion and a diminished ability to kneel. Following TKA an individual may be precluded from participating in many ordinary and recreational activities, including those that require reaching toward the ground, such as picking items off the floor, shelving and stocking, gardening, or even playing with children or pets. Surprisingly, very limited literature exists with regard to hyperflexion and kneeling for normal knees and following TKA. In this manuscript, the current biomechanical understanding of hyperflexion and kneeling before and after TKA will be discussed.
Specific amounts of knee range of motion are required for various daily living activities. Analysis of normal subjects shows that 55° of knee flexion is needed for level walking, 100° for ascending and descending stairs, 105° for arising from a seated position, and at least 120° for hyperflexion for getting up from a seated position on the floor or in a bathtub.1,2,3) Full extension and 120° of knee flexion are the goal for an optimal functional result post-TKA. There is a significant correlation between pre- and postoperative range of motion.4) Other factors influencing postoperative range of motion are the type of prosthesis used (constrained vs. non-constrained), patellofemoral tracking, knee deformities, patient motivation, and the aggressiveness of postoperative physical therapy. Joint line elevation has been shown to reduce knee flexion.5) A tight peripatellar retinaculum, inappropriate patellar resection, and poor patellofemoral tracking all can result in problems with flexion range of motion.6) The reported postoperative TKA range of motion has improved gradually over time. In 1984, Oglesby and Wilson7) reported a loss of motion-pre- to postoperatively-from 82° to 76° in rheumatoid patients, and from 98° to 85° in osteoarthritis patients. In 1990, Dodd et al.8) reported an improvement in average range of motion from 91° preoperatively to 106° postoperative. In 1999, Mont et al.9) reported that flexion range of motion improved from 92° average preoperative to 110° postoperative. Adalberth et al.10) in 2000, reported average postoperative knee flexion to be 115°. Dennis et al.11) reported average maximum knee flexion angle following TKA to be 124.1° with posterior cruciate-substituting TKA. In a recent study by Kanekasu et al.12) 18 of 36 total knees were able to achieve flexion angles greater than 145°. These subjects were capable of achieving deep flexion with a posterior-stabilized TKA even though the kinematics were shown to be significantly different from the normal knee. These improvements in knee range of motion are necessary for achieving hyperflexion and kneeling following TKA and are likely due to advances in component design and surgical techniques which may be further improved with a better understanding of TKA biomechanics.
Without full flexion range of motion, most patients cannot squat or kneel and this prevents individuals from participating in many daily, recreational, religious, and cultural activities as well as certain occupations. Hyperflexion and kneeling are required for many labor-intensive occupations, including service and construction trades. A study by Thun et al.,13) showed that carpet and floor layers experience substantially more knee morbidity than other occupational groups, and that kneeling and use of the knee kicker are risk factors, providing opportunities for prevention. Kivimaki14) in 1992 used ultrasonography to show that soft-tissue changes in the anterior knee area were associated with knee pain among carpet and floor layers, demonstrating that kneeling work increases the risk of knee disorders and identifying radiographic changes that may be an initial sign of knee degeneration.15,16) Coggon et al.17) and Cooper et al.,18) suggested that prolonged or repeated knee bending is a risk factor for knee osteoarthritis, and that risk may be higher in jobs that require knee bending and mechanical loading. Jensen and Eenberg19) showed an increased prevalence of prepatellar bursitis in subjects performing kneeling work, but suggested that occupational exposure may not be sufficiently documented as causative for meniscal lesions and chondromalacia.
Using bipolar radiographs, Hefzy et al.20) showed that beyond 135° of flexion, the patella cleared the femoral groove and was in contact only with the condyles. During deep flexion, the femur's motion on the tibia did not reveal the classic femoral "rollback." The lateral femoral condyle rolled over the posterior medial area of the lateral tibial plateau, while the medial femoral condyle's contact moved more anteriorly, but still articulated in the posterior part of the medial plateau. This asymmetric motion indicated an element of internal tibial rotation. Further, in deep flexion the tibia was found to articulate with the femur at the most proximal area of the condyles.20,21)
Schai et al.22) in 1999 showed that patients' perceived ability to kneel after TKA was less than that measured. Fear of harming the prosthesis and lack of information influenced kneeling activities in 49% of the patients (27 of 56 knees). Of those patients with observed difficulty in kneeling, pain and back-related problems seemed to be the major limiting factors. These findings indicate that kneeling should be given more consideration in rating the functional results of TKA.22)
Inability to kneel after knee surgery is a frequent cause of dissatisfaction with the treatment. Kneeling can be a painful experience for patients with degenerative knee conditions both before and after surgery. A study by Weiss et al.23) addressed patients' perspectives on important functional activities and their ability to perform them, finding that patients' expectations determine their assessment of the treatment plan's success. Among the most prevalent activities listed were kneeling and gardening, also listed as being among the most difficult to perform, with more than 75% of patients reporting limitations. Despite the success of knee arthroplasty in relieving pain, it may not restore as much function as previously thought, particularly for high-demand patients.
Biomechanical aspects of kneeling following TKA have also been investigated. In normal knees, Hassaballa et al.24) measured weight transmission to the knee while kneeling at 90° and at full flexion. They found that the average percentage of body weight transmitted to the anterior knee at 90° was 97%, and at full flexion was 51%. They suggest that improvements in range of motion post arthroplasty may reduce forces through the knee while kneeling, and therefore decrease pain. Palmer et al.25) showed differences between the perceived and actual ability to kneel after TKA, despite no difference with regard to overall knee score or range of movement. Fifty-four percent of the patients avoided kneeling because of uncertainties or recommendations from third parties. Palmer et al.25) suggest that negative advice given to patients regarding kneeling seems to have arisen out of concern for the prosthesis, although they state that no published data exists concerning this risk. They also documented radiographically two patterns of kneeling: "upright kneeling," which occurs with 90° of knee flexion, and "flexed kneeling," which occurs with more than 110° of knee flexion. The important distinction with these two kneeling patterns is based on patterns of contact with the ground. In upright kneeling the points of contact are the patella and the tibial tuberosity, while in flexed kneeling, only the tibial tuberosity bears weight. Their analysis of the kneeling radiographs showed that compressive forces through the femoral component are similar to those experienced in normal standing and walking. They also state that the tibial tray appears to be protected since it has no contact with the weight-bearing surface, and that the patellar button is in compression and experiences some shear due to its obliquity. They conclude that the forces through the prosthesis appear theoretically to be benign and hypothesize that kneeling in a flexed position (> 110°) reduces the forces across the patellofemoral articulation. Other studies have focused on the tibiofemoral kinematics with hyperflexion and kneeling12,26,27,28) but limited data exists with regards to tibiofemoral and patellofemoral contact pressure and area with respect to kneeling.
The effect of hyperflexion and kneeling on patellofemoral joint forces and patellofemoral contact pressures and area was evaluated in intact knees29) and following TKA30) in two separate studies. For the intact knees eight fresh-frozen cadaver knees were used (four males, four females; age, 73 to 88 years). For the knees that received TKA, eight fresh frozen left cadaver knees without gross deformity ranging in age from 75 to 92 years old were used with the Foundation Knee System (DJO Surgical, Austin, TX, USA). For both studies the same specimen preparation and testing system were used. The specimens were dissected free of all skin and soft tissue, leaving only the extensor mechanism, iliotibial band (ITB), joint capsule, and retinaculum intact. Vastus medialis (VM), rectus femoris (RF)/vastus intermedius (VI), vastus lateralis (VL), and ITB were separated using fascial planes. An incision was made in the suprapatellar pouch to allow insertion of Fuji film for collection of contact area and pressure data. Each knee was mounted on a custom knee testing system where a material testing machine (Instron, Norwood, MA, USA) was used to adjust and set knee flexion. Muscles were clamped on their tendinous insertions, preserving their resultant force vectors perpendicular to the muscle fibers. The clamps were attached to a cable-pulley system connected to pneumatics, all run by a customized muscle force control program. Anatomically-based multi-plane loading of the quadriceps mechanism (VM 67 N, RF/VI 111 N, VL 98 N) and the ITB (27 N) was used to simulate physiologic loading.31,32) The knee was first tested without a posteriorly-directed load on the anterior aspect of the knee to simulate hyperflexion at flexion angles of 75°, 90°, 105°, 120°, and 135° for the intact knee, for the knees following TKA flexion angles of 90°, 105°, 120°, and 135° were tested. An average body weight (70 kg = 660 N) was used to determine the force to apply to the knee. Each knee flexion angle had 3 conditions: no anterior load (to simulate squatting), 330 N (50%) to simulate double stance kneeling, and 660 N (100%) to simulate single stance kneeling. This posteriorly directed load to the knee was applied with a custom loading device designed using a 2,200 N capacity S load cell (Omega Engineering Inc., Stamford, CT, USA) and a load plate 9 cm in diameter (Fig. 1). Patellofemoral contact areas and pressures were obtained with Fuji prescale (super-low scale [intact], medium scale [TKA]) pressure-sensitive film (Sensor Products Inc., Madison, NJ, USA). A six degrees of freedom load cell (ATI Industrial Automation, Apex, CA, USA) directly measured femoral joint reaction force. For the knees following TKA, patellar kinematics were also measured. To measure patellear kinematics, two k-wires were inserted through the patella in the medial-lateral direction. Pictures were taken of each specimen at each testing position and condition using digital cameras mounted orthogonally to each other. The digital images were imported into Adobe photoshop to measure the patellofemoral, patellotibial and patellar tilt angles (Fig. 2). For statistics, repeated measures analysis of variance with Tukey post hoc analysis with a confidence level of 0.05 was performed.
Patellofemoral contact pressure and area increased significantly at 75° and 90° of knee flexion with kneeling compared to the unloaded, or squatting, condition for the intact knee; whereas following TKA contact pressure and area increased at all knee flexion angles (p < 0.05) (Fig. 3 and Table 1). For intact knees at 90° knee flexion angle and for TKA, at 135° of knee flexion, double stance kneeling had no statistically significant effect on patellofemoral joint contact area or contact pressures. Increasing the posteriorly-directed load to the knee significantly increased the femoral joint reaction force at all knee flexion angles with larger differences seen following TKA (Figs. 4 and 5). The patellofemoral angle (patellar flexion) increased with the increase in the anterior load simulating kneeling (p < 0.05) an average across all knee flexion angles of 7.4° ± 1.2° for double stance kneeling and 9.6° ± 1.0° for single stance kneeling. However, kneeling had minimal changes on patellar tilt, with statistically significant changes in patellar tilt only seen with kneeling at 120° knee flexion (1.1° ± 0.4° for single stance kneeling, p = 0.02 and 1.0° ± 0.4°, p = 0.03).
The findings from this study suggest that biomechanical demands placed on the knee increases with kneeling for both intact knees and following TKA. For intact knees at higher angles of knee flexion kneeling had minimal influence on the patellofemoral contact pressure and area due to the dominance of tendofemoral contact and lack of patellofemoral contact when kneeling at higher knee flexion angles. Following TKA with double stance kneeling, patellofemoral contact areas did not increase significantly at high knee flexion angle (135°). At higher knee flexion angles, the patella does not contact the floor in the "flexed kneeling" position25) and therefore, the ability to achieve greater postoperative range of motion in TKA may allow patients to kneel more easily, with decreased concern for premature failure of the components. The ability to kneel after TKA remains a problem that compromises the success of the procedure for some patients. The findings of this study suggest that if improvements are made in the achievable postoperative range of motion, kneeling may be performed with less discomfort, and with less risk of damage to the patellofemoral joint.
Tibiofemoral joint contact areas, pressures, and kinematics in response to kneeling were quantified in intact33) cadaveric knees and following both posterior cruciate retaining and sacrificing (posterior stabilized) TKA.34) Five cadaveric knees (3 males and 2 females) average age 84.2 years old (range, 78 to 93 years old) were dissected of all skin and subcutaneous tissue, leaving the extensor mechanism, hamstrings, joint capsule, ligaments, and retinaculum intact. The femur was potted in PVC pipe with the femoral epicondylar axis aligned perpendicular to the longitudinal axis of the pipe. A 25 mm diameter fiberglass rod was inserted and secured in the tibial intramedullary canal. The specimens were securely mounted on a custom knee testing jig that permits physiologic muscle loading and the application of an poseriorly directed force to the anterior knee to simulate kneeling (Fig. 6). The femoral epicondylar axis was aligned parallel to the coronal plane of the jig. This testing setup provided independent control of six degrees of freedom at the femur and five degrees of freedom at the tibia. The femur was locked in place during testing. Five degrees of freedom was maintained for the tibia. Anatomically based multi-plane loading of the quadriceps mechanism (VM 51 N, RF/VI 87 N, VL 77 N) and hamstrings (biceps femoris 31 N, semimembranosus/semitendinosus 54 N) was used to simulate physiologic loading of the knee joint. The knees were tested under three loading conditions at flexion angles of 90°, 105°, 120°, and 135°. The three loading conditions were as follows: no posteriorly directed load to simulate squatting; 339 N of force to simulate double stance kneeling, corresponding to 50% mean body weight (MBW) of a 70 kg person; 668 N applied to the anterior knee to simulate single stance kneeling, corresponding to 100% MBW of a 70 kg person. The load was applied at a 90° angle to the tibial axis with a load plate attached to a uniaxial load cell (Omega Engineering Inc.).
Tibiofemoral joint kinematics were determined by digitizing three points on the distal femur and three points on the proximal tibia using a Microscribe 3DLX (Revware Inc, Raleigh, NC, USA). The distal femur points were on the lateral femoral epicondyle, the medial femoral epicondylar sulcus, and the posterior femur 6 cm superior to tibiofemoral joint line. The proximal tibia points were on Gerdy's tubercle laterally, a point 3 cm inferior to the medial joint line and centered in the anteroposterior tibial plane, and the posterior tibia 6 cm inferior to tibiofemoral joint line. Tibiofemoral joint contact characteristics were measured using Tekscan (Tekscan Inc., South Boston, MA, USA). The Tekscan sensors were inserted through the posterior capsule, taking care to preserve the menisci, collateral ligaments, and cruciate ligaments. Tibiofemoral joint contact areas, contact pressures, and peak contact pressures were obtained for the medial and lateral joint compartments. Repeated measures analysis of variance with a Tukey's post hoc test was used for statistical analysis (p < 0.05).
Following testing of the intact knees TKA was performed using the Encore Foundation System (DJO Surgical) according to the manufacturer's protocol. First the cruciate retaining TKA components were then implanted and cemented in place with plaster of Paris. The cruciate retaining TKA specimens were then subjected to the testing protocol. Thereafter, the femoral component was carefully removed and the femoral cutting guide for the posterior stabilized TKA system was utilized in order to cut the femoral box. The posterior stabilized component was then cemented in place, and a posterior stabilized tibial polyethylene insert was inserted. The posterior stabilized TKA specimens were then subjected to the testing protocol.
For the intact knee medial and lateral tibiofemoral contact pressures and areas increased significantly (p < 0.007) with both double stance and single stance kneeling across all flexion angles with the exception of the medial compartment at 135°, where contact area increased significantly with only single stance kneeling (p = 0.03) (Fig. 7, Tables 2 and 3). Following TKA double and single stance kneeling significantly increased contact areas in both designs (p < 0.05). Double and single stance kneeling increased tibiofemoral contact pressures compared to squatting with variable significance in both groups (p < 0.05). Moving from double to single stance kneeling tended to increase pressures in the cruciate retaining group, but decreased pressures in the posterior stabilized group. The cruciate retaining group had significantly larger contact areas than the posterior stabilized group, although no significant difference in pressures were observed comparing the two TKA designs (p < 0.05).
Kneeling resulted in tibial posterior translation and external rotation at all flexion angles. The averages of posterior translation and external rotation across all knee flexion angles are shown in Figs. 8 and 9, respectively. There was a significantly smaller effect on tibiofemoral kinematics when moving from double stance kneeling to single stance kneeling (p < 0.005). Knees with both TKA designs exhibited tibial external rotation with kneeling, though the cruciate retaining group exhibited more variability between specimens. When moving from squatting to double stance kneeling the posterior stabilized design exhibited significantly more posterior translation than compared to the change when going from double to single stance kneeeling (p < 0.05). At 90° the posterior stabilized knees exhibited significantly less posterior translation than at higher flexion angles (p < 0.05). Both of these trends were observed in the cruciate retaining group but were not statistically significant.
Increased tibiofemoral contact areas and pressures seen with kneeling may increase the likelihood of damage to cartilage and menisci in intact knees as well as indicate a potential for increased polyethylene wear. The smaller contact areas in the posterior stabilized group likely result from contact being partly assumed by the cam-post articulation, causing similar contact pressures at the articulation of the condyles and tibial polyethylene surface between the groups. Kneeling also produces more consistent tibial external rotation with a posterior stabilized design likely due to more variable biomechanics of the native posterior cruciate ligament (PCL) in the cruciate retaining group. Both designs limit posterior translation with kneeling most effectively at 90° than at higher flexion angles, possibly due to the biomechanics of the PCL at this flexion angle in the cruciate retaining group and improved cam-post interaction in the posterior stabilized group.
Inability to kneel or squat imposes important functional limitations on TKA patients, who currently must accept significant consequences of limited knee motion. The often-reported range of motion of 115° to 120° following TKA is certainly not typical. More commonly, a range of motion of only 90° to 100° is achieved. Although range of motion following TKA depends on many factors, accommodating the ability to squat and kneel requires improved knee prosthetic designs or surgical techniques and/or recommendations for kneeling to minimize adverse affects on the prosthesis. The knee's structural integrity following TKA will be significantly challenged during kneeling and hyperflexion. This is based on altered knee kinematics for both the tibiofemoral and patellofemoral joint, increased contact pressures, decreased contact areas, eccentric loading patterns, and edge loading patterns, which are suggested to be significantly magnified for kneeling and hyperflexion. These studies from our laboratory provide new information on effects of kneeling and hyperflexion before and after TKA. The ability to kneel after TKA remains a problem that compromises the success of the procedure for some patients. These findings suggest that if greater than 120° of postoperative knee range of motion can be achieved following TKA then kneeling may be performed with less risk in the patellofeoral joint than was previously believed to be the case. However, kneeling after TKA increases tibiofemoral contact area and pressures, and may increase polyethyelene wear if performed on a chronic, repetitive basis.
Figures and Tables
Fig. 1
Custom testing sytem used for the quantification of effect of kneeling on patellofemoral joint contact for the intact, normal knee (A) and following total knee arthroplasty (B).
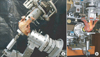
Fig. 3
Representative Fuji film patellofemoral joint contact patterns for squatting and double stance kneeling.
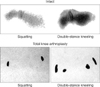
Fig. 4
Histogram showing resultant force for the intact knee with kneeling. *p < 0.05 vs. squatting. †p < 0.05 vs. single stance kneeling.
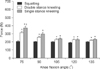
Fig. 5
Histogram showing resultant force following total knee arthroplasty with kneeling. *p < 0.05 vs. squatting. †p < 0.05 vs. single stance kneeling.
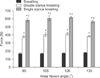
Fig. 7
Representative Tekscan images of tibiofemoral contact patterns of an intact knee and following total knee arthroplasty (TKA).
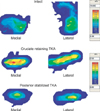
Fig. 8
Tibial translation with kneeling averaged across all knee flexion angles for intact knees, and following cruciate retaining and posterior stabilized total knee arthroplasty (TKA).
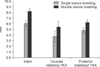
Fig. 9
Tibial external rotation with kneeling averaged across all knee flexion angles for intact knees, and following cruciate retaining and posterior stabilized total knee arthroplasty (TKA).
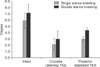
ACKNOWLEDGEMENTS
Funding was provided in part by VA Rehabilitation Research and Development and Merit Review, California Orthopaedic Research Institute and John C Griswold Foundation. TKA implants were donated by DJO Surgical (Austin, TX, USA).
References
1. Daluga D, Lombardi AV Jr, Mallory TH, Vaughn BK. Knee manipulation following total knee arthroplasty: analysis of prognostic variables. J Arthroplasty. 1991; 6(2):119–128.
2. Fox JL, Poss R. The role of manipulation following total knee replacement. J Bone Joint Surg Am. 1981; 63(3):357–362.
3. Robinson RP, Simonian PT, McCann KJ. Rehabilitation following total knee arthroplasty. In : Fu F, Harner C, Vince K, editors. Knee surgery. Baltimore: Williams & Wilkins;1994. p. 1414–1416.
4. Ritter MA, Stringer EA. Predictive range of motion after total knee replacement. Clin Orthop Relat Res. 1979; (143):115–119.
5. Cummings JF, Carpenter CW, Grood ES, Leach DU, Manley MT. Joint line elevation of a total knee replacement results in reduction of knee flexion. In : Transactions of the 36th Annual Meeting of the Orthopaedic Research Society; 1990 Feb 5-8; New Orleans, LA.
6. Holtgrewe J, Haynes DW, Hungerford DS. The effect of prosthetic patella thickness and anterior femoral surface on limiting flexion in total knee arthroplasty. In : Transactions of the 35th Annual Meeting of the Orthopaedic Research Society; Las Vegas, NV. 1989.
7. Oglesby JW, Wilson FC. The evolution of knee arthroplasty: results with three generations of prostheses. Clin Orthop Relat Res. 1984; (186):96–103.
8. Dodd CA, Hungerford DS, Krackow KA. Total knee arthroplasty fixation: comparison of the early results of paired cemented versus uncemented porous coated anatomic knee prostheses. Clin Orthop Relat Res. 1990; (260):66–70.
9. Mont MA, Yoon TR, Krackow KA, Hungerford DS. Eliminating patellofemoral complications in total knee arthroplasty: clinical and radiographic results of 121 consecutive cases using the Duracon system. J Arthroplasty. 1999; 14(4):446–455.
10. Adalberth G, Nilsson KG, Bystrom S, Kolstad K, Milbrink J. Low-conforming all-polyethylene tibial component not inferior to metal-backed component in cemented total knee arthroplasty: prospective, randomized radiostereometric analysis study of the AGC total knee prosthesis. J Arthroplasty. 2000; 15(6):783–792.
11. Dennis DA, Haas B, Komistek RD, Brumley JT, Dennis T. Range of motion of posteior cruciate substituting total knee arthroplasty: the effect of bearing mobility. In : 68th Annual Meeting American Academy of Orthopaedic Surgeons; 2001 Feb 28-Mar 4; San Francisco, CA.
12. Kanekasu K, Banks SA, Honjo S, Nakata O, Kato H. Fluoroscopic analysis of knee arthroplasty kinematics during deep flexion kneeling. J Arthroplasty. 2004; 19(8):998–1003.
13. Thun M, Tanaka S, Smith AB, et al. Morbidity from repetitive knee trauma in carpet and floor layers. Br J Ind Med. 1987; 44(9):611–620.
14. Kivimaki J. Occupationally related ultrasonic findings in carpet and floor layers' knees. Scand J Work Environ Health. 1992; 18(6):400–402.
15. Kivimaki J, Riihimaki H, Alaranta H. Knee disorders in carpet and floor layers and painters. Part I. Isometric knee extension and flexion torques. Scand J Rehabil Med. 1994; 26(2):91–95.
16. Kivimaki J, Riihimaki H, Hanninen K. Knee disorders in carpet and floor layers and painters. Scand J Work Environ Health. 1992; 18(5):310–316.
17. Coggon D, Croft P, Kellingray S, Barrett D, McLaren M, Cooper C. Occupational physical activities and osteoarthritis of the knee. Arthritis Rheum. 2000; 43(7):1443–1449.
18. Cooper C, McAlindon T, Coggon D, Egger P, Dieppe P. Occupational activity and osteoarthritis of the knee. Ann Rheum Dis. 1994; 53(2):90–93.
19. Jensen LK, Eenberg W. Occupation as a risk factor for knee disorders. Scand J Work Environ Health. 1996; 22(3):165–175.
20. Hefzy MS, Kelly BP, Cooke TD. Kinematics of the knee joint in deep flexion: a radiographic assessment. Med Eng Phys. 1998; 20(4):302–307.
21. Hefzy MS, Kelly BP, Cooke TD, al-Baddah AM, Harrison L. Knee kinematics in-vivo of kneeling in deep flexion examined by bi-planar radiographs. Biomed Sci Instrum. 1997; 33:453–458.
22. Schai PA, Gibbon AJ, Scott RD. Kneeling ability after total knee arthroplasty: perception and reality. Clin Orthop Relat Res. 1999; (367):195–200.
23. Weiss JM, Noble PC, Conditt MA, et al. What functional activities are important to patients with knee replacements? Clin Orthop Relat Res. 2002; (404):172–188.
24. Hassaballa M, Vale T, Weeg N, Hardy JR. Kneeling requirements and arthroplasty surgery. Knee. 2002; 9(4):317–319.
25. Palmer SH, Servant CT, Maguire J, Parish EN, Cross MJ. Ability to kneel after total knee replacement. J Bone Joint Surg Br. 2002; 84(2):220–222.
26. Coughlin KM, Incavo SJ, Doohen RR, Gamada K, Banks S, Beynnon BD. Kneeling kinematics after total knee arthroplasty: anterior-posterior contact position of a standard and a high-flex tibial insert design. J Arthroplasty. 2007; 22(2):160–165.
27. Incavo SJ, Mullins ER, Coughlin KM, Banks S, Banks A, Beynnon BD. Tibiofemoral kinematic analysis of kneeling after total knee arthroplasty. J Arthroplasty. 2004; 19(7):906–910.
28. Komistek RD, Scott RD, Dennis DA, Yasgur D, Anderson DT, Hajner ME. In vivo comparison of femorotibial contact positions for press-fit posterior stabilized and posterior cruciate-retaining total knee arthroplasties. J Arthroplasty. 2002; 17(2):209–216.
29. Bingenheimer E, McGarry MH, Lee TQ. Biomechanical effects of kneeling on the patellofemoral joint. In : Transactions of the 51st Annual Meeting of the Orthopaedic Research Society; 2005 Feb 20-23; Washington, DC.
30. Wilkens KJ, Duong LV, McGarry MH, Kim WC, Lee TQ. Biomechanical effects of kneeling after total knee arthroplasty. J Bone Joint Surg Am. 2007; 89(12):2745–2751.
31. Powers CM, Lilley JC, Lee TQ. The effects of axial and multi-plane loading of the extensor mechanism on the patellofemoral joint. Clin Biomech (Bristol, Avon). 1998; 13(8):616–624.
32. Wickiewicz TL, Roy RR, Powell PL, Edgerton VR. Muscle architecture of the human lower limb. Clin Orthop Relat Res. 1983; (179):275–283.
33. Hofer JK, Gejo R, McGarry MH, Lee TQ. Effects on tibiofemoral biomechanics from kneeling. Clin Biomech (Bristol, Avon). 2011; 26(6):605–611.
34. Hofer JK, Gejo R, McGarry MH, Lee TQ. Effects of kneeling on tibiofemoral contact pressure and area in posterior cruciate-retaining and posterior cruciate-sacrificing total knee arthroplasty. J Arthroplasty. 2012; 27(4):620–624.