Abstract
Background
The restriction of wrist motion results in limited hand function, and the evaluation of the range of wrist motion is related to the evaluation of wrist function. To analyze and compare the wrist motion during four selected tasks, we developed a new desktop motion analysis system using the motion controller for a home video game console.
Methods
Eighteen healthy, right-handed subjects performed 15 trials of selective tasks (dart throwing, hammering, circumduction, and winding thread on a reel) with both wrists. The signals of light-emitting diode markers attached to the hand and forearm were detected by the optic receptor in the motion controller. We compared the results between both wrists and between motions with similar motion paths.
Results
The parameters (range of motion, offset, coupling, and orientations of the oblique plane) for wrist motion were not significantly different between both wrists, except for radioulnar deviation for hammering and the orientation for thread winding. In each wrist, the ranges for hammering were larger than those for dart throwing. The offsets and the orientations of the oblique plane were not significantly different between circumduction and thread winding.
Conclusions
The results for the parameters of dart throwing, hammering, and circumduction of our motion analysis system using the motion controller were considerably similar to those of the previous studies with three-dimensional reconstruction with computed tomography, electrogoniometer, and motion capture system. Therefore, our system may be a cost-effective and simple method for wrist motion analysis.
The evaluation of the range of wrist motion is related to the evaluation of wrist function.1) There are several ways to assess wrist motions including electrogoniometry,23) three-dimensional (3D) motion capture system,45) reconstruction with computed tomography (CT) or magnetic resonance imaging (MRI) data,678910111213) measurement with light-reflective or light-emitting markers,1415) and magnetic tracking devices.16) Of these, the motion capture system is most commonly used; however, it requires expensive special infrared cameras, special programs, and sufficient space. Recently, the Wiimote (Nintendo, Kyoto, Japan) has been applied in the research of human body movements such as tremors, respiration tracking, and head postures.171819) The purpose of this study was to analyze and compare four selected wrist motions three-dimensionally using the Wiimote.
Eighteen participants (15 males and three females) without a history of wrist trauma or disease were recruited. We conducted this study in compliance with the principles of the Declaration of Helsinki. The protocol of this study was reviewed and approved by the Institutional Review Board of Inje University Sanggye Paik Hospital (IRB No. SGPAIK 2014-08-002). Written informed consents were obtained. The average age of the participants was 35 years (range, 27 to 44 years) and all were right-handed.
The light-emitting diode (LED) trackers, composed of four 950 nm infrared LEDs, were taped onto the dorsal aspects of the third metacarpal shaft and on the dorsum of Lister's tubercle, not crossing the wrist crease. Infrared signals were detected using optical tracking devices with two Wiimotes and were transformed into data in real-time using a program developed by Microsoft Visual Studio 2005 (Microsoft, Seattle, WA, USA), which performed the coordinate calculation. Two-dimensional coordinate information of infrared signals from the calibration box (Withrobot, Seoul, Korea) was converted into 3D coordinate information using a stereo-matching method to make the virtual 3D space reflect the actual experimental environment.
We selected four tasks that could reflect the functional status of the wrist joint: (1) dart throwing, (2) hammering, (3) circumduction, and (4) winding thread on a reel (Fig. 1). Dart throwing, composed of radial extension and ulnar flexion, is a representative task that utilizes the wrist joint efficiently. Each subject threw a dart 15 times. Hammering motion was performed 15 times by each patient with a mallet to help subjects simulate the motion more comfortably. Range of motion (ROM) in dart throwing and hammering was also measured with a goniometer. In circumduction, the participants rotated their right wrists clockwise and their left wrists counterclockwise 15 times as much as possible at a comfortable speed. For thread winding, the participants were instructed to wind the thread 20 turns using the one hand on a reel held by the other hand as fast as they could, while the forearm was fixed, and the wrist motion and time spent were measured.
The Z-axis was established along the longitudinal axis of the forearm using the forearm marker. The location of the virtual origin of the coordinate system of the wrist motion was marked on the Z-axis. The X-axis (radial [−] and ulnar [+] deviation) was defined perpendicular to the Z-axis in the same plane made by the forearm marker, and the Y-axis (flexion [−] and extension [+]) was defined by the Z-axis and X-axis. The relative differences of the hand's local coordinate to the forearm's coordinate were calculated to reveal the real-time wrist position. As the Z-axis (supination and pronation) was considered to be fixed, the hand's coordinate in this coordinate system described by the X-axis (radial and ulnar deviation) and Y-axis (flexion and extension) was sufficient to evaluate the wrist motion. The hand's location was described by the angles between the position vector of the hand marker in this coordinate system and the X-axis and between the vector and the Y-axis.
The averages of total ROM and the offsets in flexion-extension (FE) and radioulnar deviation (RUD) were calculated. The offsets are the deviation from the kinetic motion path centroid to the FE and RUD axis,4) which can correspond to the medians of ROM. The parameters between similar tasks, such as dart throwing and hammering, or circumduction and thread winding, were compared. As dart throwing and hammering might have a curved kinematic motion path, the principal axis of the motion curve was modeled using the linear-fitting method, and coupling between FE and RUD was calculated as the angle between the principal axis and the X-axis (Fig. 2A).4) On circumduction and thread winding, the circumduction curve was drawn using the curve- and circle-fitting method. The orientation of the oblique circumduction plane was calculated as the angle between the long axis of the elliptical curve or the oblique plane of the circumduction curve and the X-axis, and the area under the circumduction curve was calculated to describe the two-dimensional maximal motion range of circumduction (Fig. 2B).3)
The averages of the parameters of the participants were compared statistically using a nonparametric statistical test such as the Wilcoxon rank-sum test and Wilcoxon signed-rank test because the paired data were 18 sets from 18 subjects.
The ROM values measured with our motion analysis system in dart throwing and hammering were not significantly different from those measured with a goniometer (Table 1). On dart throwing and hammering, their linear-fitting axes were from radial extension to ulnar flexion (Fig. 3A and B). The average of coupling in dart throwing was 63.0° in the right wrist and 58.6° in the left wrist (Table 2). On dart throwing, the average ROM was 34.0° in both sides in FE and 22.1° in the right wrist and 20.3° in the left wrist in RUD. The averages of offset were located on the dorsoulnar side, with the right offset was located more toward the ulnar side.
The average of coupling in hammering was 65.2° in the right side and 60.7° in the left side. On hammering, the average ROM of FE was 49.9° in the right wrist and 46.8° in the left wrist, showing no statistical difference between both wrists, but the average ROM of RUD of the right wrist was 37.2°, which was larger than 29.6° of the left wrist (p = 0.005). The right offset in hammering was located more in extension (p = 0.003) and more towards the ulnar side (p = 0.001).
Circumduction and thread winding were similar ellipsoid motions (Fig. 3C and D). On circumduction, the average orientation of the oblique circumduction plane was 21.8° in the right wrist and 27.6° in the left wrist. The averages of ROM and offset were not significantly different between both wrists (p > 0.05).
On thread winding, the average orientation of the oblique plane was 22.7° in the right wrist and 27.2° in the left wrist, showing significant difference (p = 0.005). The averages of ROM and offset were not significantly different between both wrists (p > 0.05).
The circumduction curve for circumduction and thread winding was elliptical. For circumduction, the area under the curve of the right was greater than that of the left, which revealed that the right wrist executed exercises in a wider range (Table 3). However, for thread winding, the area of the right was smaller than that of the left. The average time for thread winding for 20 turns in the right wrist (19.0 seconds) was shorter than that of the left (19.6 seconds, p = 0.01). The ratio of the length of the long axis to that of the short axis was smaller in the right wrist than in the left wrist.
On comparison of dart throwing and hammering, coupling was not significantly different in the right (p = 0.948) and in the left (p = 0.647) (Table 4). The ROM values of FE and RUD for hammering were larger than those for dart throwing, but offsets were not significantly different in each side of the wrist.
For circumduction and thread winding, the orientations of the oblique plane were not significantly different between the two motions. The ROM values of FE and RUD in circumduction were larger than those in thread winding. Offsets were not significantly different in both wrists.
We used the technique of acquiring 3D-location information using infrared sensors of the Wiimote and calculated the wrist motion parameters in four selective tasks.
There have been some studies presenting coupling of dart throwing (Table 5). Goto et al.20) evaluated the scaphoid and lunate motion using a volume-based registration model from MRI and presented that dart-throwing plane was deviated 22.9° ± 8.8° ulnarly, which corresponded to 67.1° ± 8.8° by coupling as in our study. Moritomo et al.12) reported that coupling of dart throwing was 59° ± 9°. Garg et al.4) analyzed various wrist motions including dart throwing and hammering with 10 right-handed men using a 3D motion capture system and presented coupling of dart throwing was 64° ± 9°. Coupling of our study was considerably similar to that of previous studies. On dart throwing, the results of our study were considerably similar to those of the study by Garg et al.,4) except that our FE range was smaller (Table 5).
On hammering, the results of three studies were various (Table 6), which might result from differences in the technique of hammering used in each experiment. There has been no gold standard method of hammering proposed in academic fields. The actual behavior of driving a nail with a metal hammer to insert a nail and simulated hammering motion with a light rubber mallet might have different motion paths, and the simulated hammering in the three studies might be different. In the study of Leventhal et al.,13) hammering was simulated in five targeted positions along the hammering path, which was not actual real-time motion. Garg et al.4) utilized a ball-peen hammer to drive nails actually, and we used a lighter rubber-plastic mallet only to simulate hammering motion. Comparison among studies on hammering motion should be further investigated once gold standard methods are established.
Leventhal et al.13) performed a simulated hammering task and reconstructed a 3D bone surface model from CT images and reported that the motion path was 41° in the sagittal plane (corresponding to 49° by coupling as in our study) which was smaller than our result (Table 6). The range of hammering in our study was smaller, but the offset seemed similar to their results. Various factors seem to cause the different hammering patterns. The motion paths by ordinary persons and professional hammerers may differ. According to the condition in each experiment, the speed and the force applied on the stroke may cause variance in the coupling and ROM. However, three studies including our study observed similar values of coupling and reported that the offset of hammering was located in extension and ulnar deviation.413) Hammering also has a motion path from radial extension to ulnar flexion, which is similar to that of dart throwing, but it requires more force applied on the stroke. A larger ROM was expected in hammering than in dart throwing because dart throwing is performed mainly from radial extension to a nearly neutral position of the wrist, where the fingers release the darts; whereas hammering should be performed throughout the ulnar flexion, where the acceleration force added during the motion arc is essential for effective hammering. The results support our conjecture that the ranges of FE and RUD in hammering would be significantly larger than those in dart-throwing motion in both wrists. However, the offsets and coupling were not statistically different between the two motions in any side of the wrists.
Singh et al.3) measured circumduction in both wrists with an electrogoniometer. The shape of the circumduction curves in our study was similar to that in their study. However, the angle of oblique circumduction plane was smaller in the dominant hand, but similar in the nondominant hand (Table 7). The ranges of FE and RUD, and the area of circumduction were smaller in our study. Our study was performed in Asian participants. The participants in the study by Singh et al.3) may be Caucasian, as the study was performed in the Netherlands, although the races of the participants were not revealed. Racial difference, such as in the flexibility or stability of the carpal ligaments and the size of the carpal bones, might result in the difference in circumduction range. There were two females out of 18 participants in our study, whereas 14 females out of 38 participants in the study by Singh et al.,3) which may explain the smaller ROM in our study; females generally have more flexible wrist motion. Our study used sets of infrared LED markers attached on the dorsum of the hand and distal forearm, which might be heavier and larger than an electrogoniometer and could have prevented the participants from moving the wrist freely to the maximum range.
On comparison of the parameters of the wrist motions between the right (dominant) and left wrists, the offset of dart throwing and hammering were located more toward the ulnar side. These two motions are used when the hand is aiming at the chosen target and the directions of the motions are similar. Garg et al.4) showed the offsets of RUD were located in the ulnar position for wrist motions for basketball free-throwing, football throwing, overhand baseball throwing, clubbing, pouring, as well as dart throwing and hammering. Dart throwing and hammering might be performed better with the wrist more in ulnar position, and dominant wrists might achieve the effective position better than nondominant wrists.
On hammering, the averages ROM of RUD of the right wrist were larger and the right offset was located more in extension and more towards the ulnar side. Hammering requires force applied on the stroke, and the dominant hand can perform more accelerated and more powerful stroke with a larger ROM. Power stroke could be achieved by the action of the flexor carpi ulnaris muscle and it might move the offset more toward the ulnar side in the dominant hand. An increased ROM might move the offset more in extension because the wrist position should be maintained at the moment of stroke while snapping in ulnar flexion and more radial extension could only be possible to increase the arc of motion of the wrist. However, some studies reported that the simple active ROM of dominant wrists without powerful stroke was smaller than that of nondominant wrists.2122) Further studies are required to figure out the differences of hammering between dominant and nondominant wrists.
The right wrist executed circumduction, or the maximum rotational movement, in a wider range than the left wrist, thus it seemed natural that all the right wrists were the dominant wrists in this study. For thread winding, the area under the curve of the right was smaller and the average time in the right wrist was shorter than that of the left. These findings imply that the right, dominant wrists executed winding motion more precisely and efficiently.
Circumduction, the motion with the maximal range of the wrist, and thread winding on a reel were circular motions of the wrist with similar, elliptical motion paths, although the range of circumduction was significantly larger than that of thread winding. Thread winding on a reel was a test devised by one of the authors on an assumption that this motion could not be performed well or take a longer time if the wrist ROM was limited or the wrist function was impaired. The ROM of circumduction was larger than that of thread winding, but the offset and orientation of the oblique plane were significantly different between the two motions in both wrists.
There were several limitations of our study. First, the number of the participants was small (18 participants including three females), and thus the study had a low statistical power. Second, the sensors were attached on the skin and the position might have been changed during wrist motions, which could have impacted the accurate representation of joint motions. However, the differences between the motion of the bone and joint and the motions acquired from the skin would be negligible and the standard 3D motion capture system also used the skin markers. Third, we did not perform 3D motion capture analysis and could not compare directly our results with this gold standard method. We were not equipped with a 3D motion capture system, and could help but compare our results with the results of the similar motions found in the references. Comparison of tracking accuracy with a more standard motion capture system and/or more accurate tracking system would strengthen the power of this study. Fourth, in the absence of any standardized methods for evaluation of the four wrist motions included in our study, we established our own methods. Therefore, the results of our study could only be indirectly compared with those of other studies. Fifth, thread winding was first used in this study to evaluate wrist function and thus could not be compared with other studies.
We developed a new desktop motion analysis system using the motion controller for a home video game console. The results from our experiment showed similar kinematic motion paths to other studies using the motion capture system. Our motion analysis system may be a cost-effective and simple method for wrist motion analysis, which might be applied to evaluate the pathological conditions and outcomes of the treatment.
ACKNOWLEDGEMENTS
The present work benefited from the help of Minkyu Seong, MD (Inje University Sanggye Paik Hospital) who helped with statistical analyses.
This work was supported by Basic Science Research Program through the National Research Foundation of Korea funded by the Ministry of Education, Science and Technology of South Korea (NRF-2013R1A1A3004855).
References
1. Franko OI, Zurakowski D, Day CS. Functional disability of the wrist: direct correlation with decreased wrist motion. J Hand Surg Am. 2008; 33(4):485–492. PMID: 18406951.


2. Salvia P, Woestyn L, David JH, et al. Analysis of helical axes, pivot and envelope in active wrist circumduction. Clin Biomech (Bristol, Avon). 2000; 15(2):103–111.


3. Singh HP, Dias JJ, Slijper H, Hovius S. Assessment of velocity, range, and smoothness of wrist circumduction using flexible electrogoniometry. J Hand Surg Am. 2012; 37(11):2331–2339. PMID: 23101531.


4. Garg R, Kraszewski AP, Stoecklein HH, et al. Wrist kinematic coupling and performance during functional tasks: effects of constrained motion. J Hand Surg Am. 2014; 39(4):634–642.e1. PMID: 24582842.


5. Wolff AL, Garg R, Kraszewski AP, et al. Surgical treatments for scapholunate advanced collapse wrist: kinematics and functional performance. J Hand Surg Am. 2015; 40(8):1547–1553. PMID: 26092664.


6. Wolfe SW, Crisco JJ, Katz LD. A non-invasive method for studying in vivo carpal kinematics. J Hand Surg Br. 1997; 22(2):147–152. PMID: 9149975.


7. Crisco JJ, McGovern RD, Wolfe SW. Noninvasive technique for measuring in vivo three-dimensional carpal bone kinematics. J Orthop Res. 1999; 17(1):96–100. PMID: 10073653.
8. Feipel V, Rooze M. Three-dimensional motion patterns of the carpal bones: an in vivo study using three-dimensional computed tomography and clinical applications. Surg Radiol Anat. 1999; 21(2):125–131. PMID: 10399213.


9. Snel JG, Venema HW, Moojen TM, Ritt JP, Grimbergen CA, den Heeten GJ. Quantitative in vivo analysis of the kinematics of carpal bones from three-dimensional CT images using a deformable surface model and a three-dimensional matching technique. Med Phys. 2000; 27(9):2037–2047. PMID: 11011731.
10. Wolfe SW, Neu C, Crisco JJ. In vivo scaphoid, lunate, and capitate kinematics in flexion and in extension. J Hand Surg Am. 2000; 25(5):860–869. PMID: 11040301.


11. Moritomo H, Goto A, Sato Y, Sugamoto K, Murase T, Yoshikawa H. The triquetrum-hamate joint: an anatomic and in vivo three-dimensional kinematic study. J Hand Surg Am. 2003; 28(5):797–805. PMID: 14507511.


12. Moritomo H, Murase T, Goto A, Oka K, Sugamoto K, Yoshikawa H. In vivo three-dimensional kinematics of the midcarpal joint of the wrist. J Bone Joint Surg Am. 2006; 88(3):611–621. PMID: 16510829.


13. Leventhal EL, Moore DC, Akelman E, Wolfe SW, Crisco JJ. Carpal and forearm kinematics during a simulated hammering task. J Hand Surg Am. 2010; 35(7):1097–1104. PMID: 20610055.


14. Li ZM, Kuxhaus L, Fisk JA, Christophel TH. Coupling between wrist flexion-extension and radial-ulnar deviation. Clin Biomech (Bristol, Avon). 2005; 20(2):177–183.


15. van Andel CJ, Wolterbeek N, Doorenbosch CA, Veeger DH, Harlaar J. Complete 3D kinematics of upper extremity functional tasks. Gait Posture. 2008; 27(1):120–127. PMID: 17459709.


16. Ishikawa J, Niebur GL, Uchiyama S, et al. Feasibility of using a magnetic tracking device for measuring carpal kinematics. J Biomech. 1997; 30(11-12):1183–1186. PMID: 9456389.


17. Mamorita N, Iizuka T, Takeuchi A, Shirataka M, Ikeda N. Development of a system for measurement and analysis of tremor using a three-axis accelerometer. Methods Inf Med. 2009; 48(6):589–594. PMID: 19893856.


18. Guirao Aguilar J, Bellika JG, Fernandez Luque L, Traver Salcedo V. Respiration tracking using the Wii remote game controller. Stud Health Technol Inform. 2011; 169:455–459. PMID: 21893791.
19. Peng Y, Vedam S, Gao S, Balter P. A new respiratory monitoring and processing system based on Wii remote: proof of principle. Med Phys. 2013; 40(7):071712. PMID: 23822416.


20. Goto A, Moritomo H, Murase T, et al. In vivo three-dimensional wrist motion analysis using magnetic resonance imaging and volume-based registration. J Orthop Res. 2005; 23(4):750–756. PMID: 16022986.


21. Gunal I, Kose N, Erdogan O, Gokturk E, Seber S. Normal range of motion of the joints of the upper extremity in male subjects, with special reference to side. J Bone Joint Surg Am. 1996; 78(9):1401–1404. PMID: 8816658.
22. Macedo LG, Magee DJ. Differences in range of motion between dominant and nondominant sides of upper and lower extremities. J Manipulative Physiol Ther. 2008; 31(8):577–582. PMID: 18984240.


Fig. 1
Four selective tasks of the wrist motion. (A) Dart throwing. (B) Hammering. (C) Circumduction. (D) Winding thread on a reel.
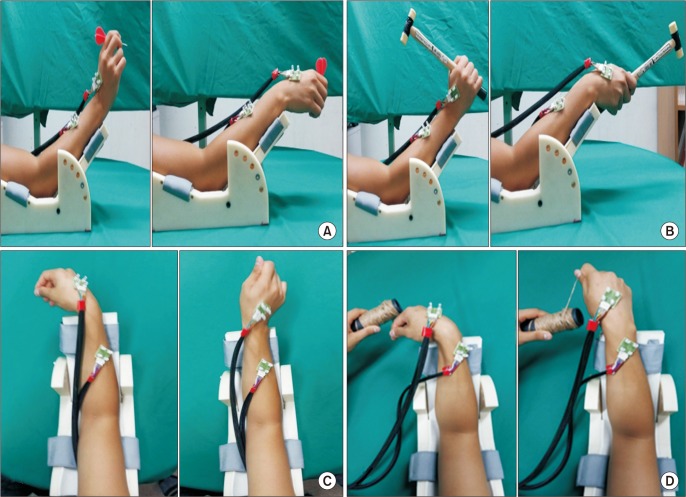
Fig. 2
Principal axes in the wrist motion. (A) Coupling. (B) Orientation of the oblique circumduction plane. FE: flexion-extension, ROM: range of motion, RUD: radioulnar deviation.
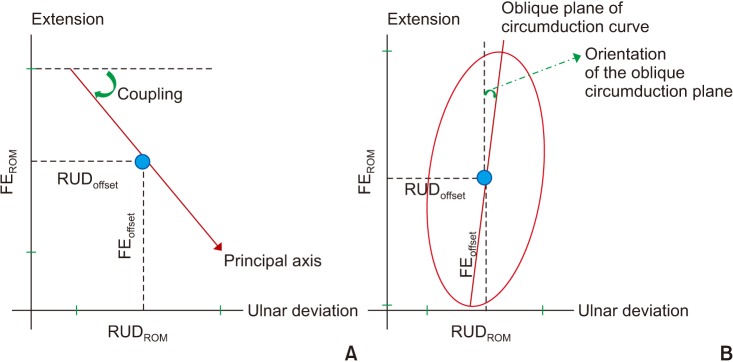
Fig. 3
Kinematic motion paths for dart throwing (A), hammering (B), circumduction (C), and thread winding (D).
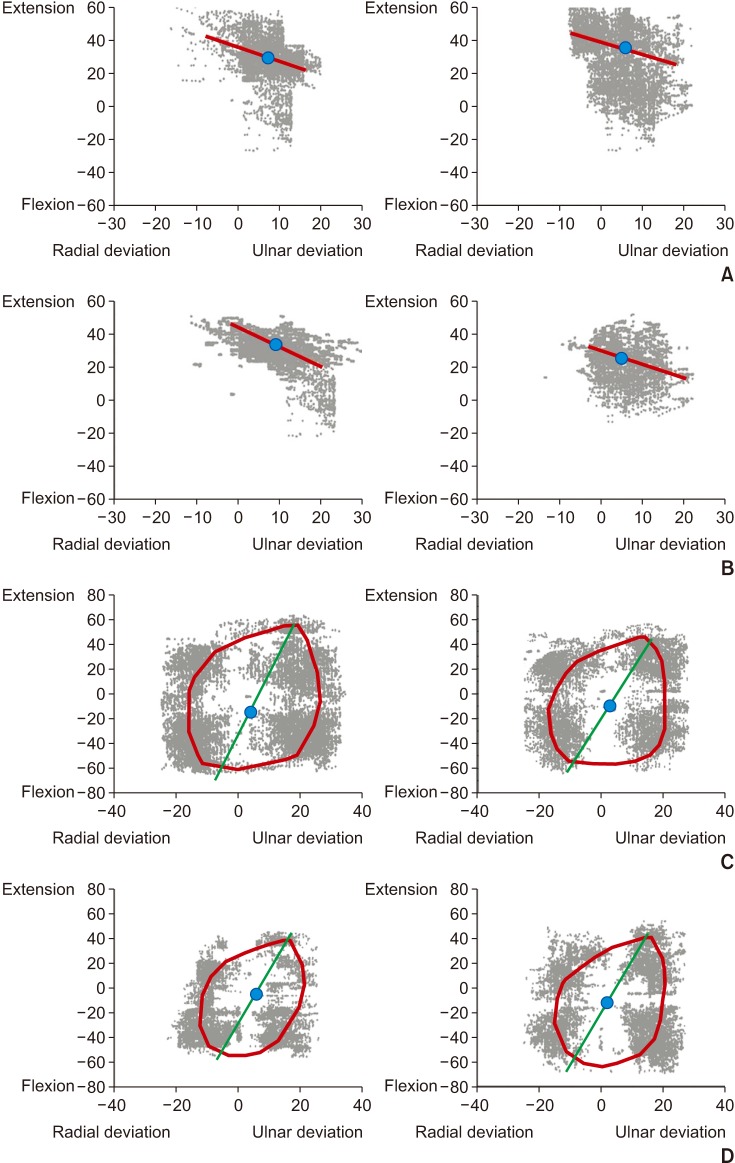
Table 1
Comparison of ROM Measured with Our New Motion Analysis System and a Goniometer in Dart Throwing and Hammering
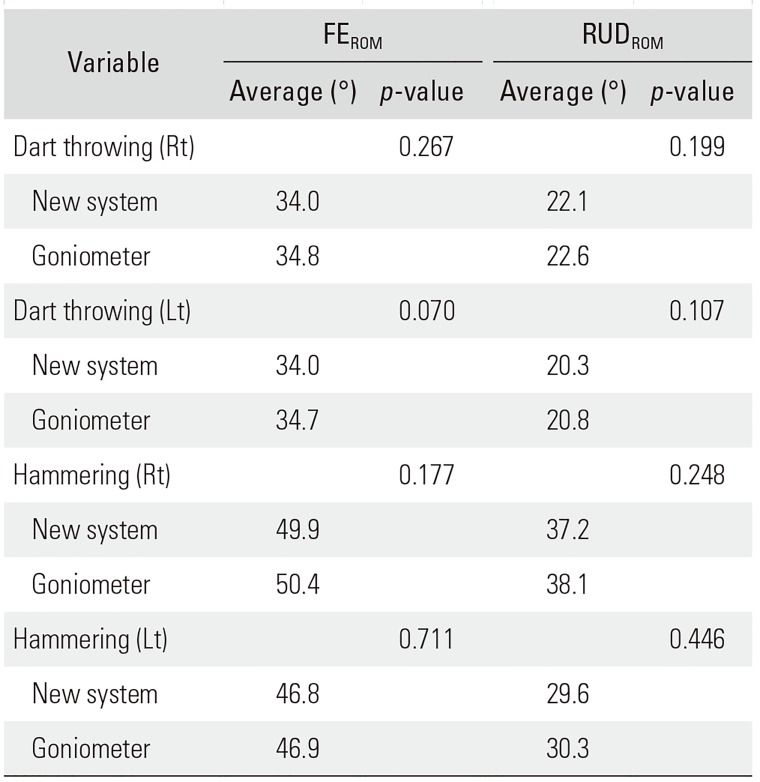
Table 2
Average Range of Motion, Offset, and Principal Axis of Four Selective Tasks of the Wrist
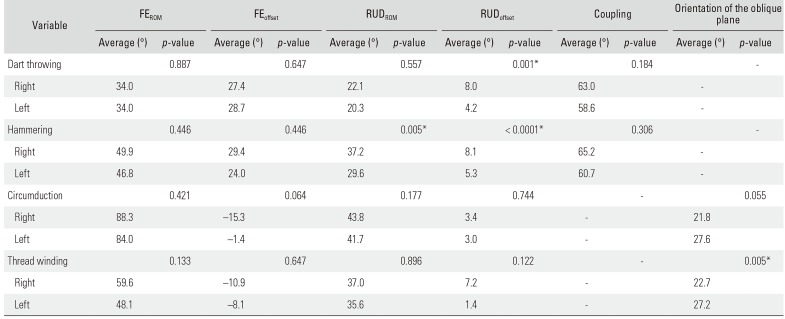
Table 3
Measurement of the Curves of Circumduction and Thread Winding
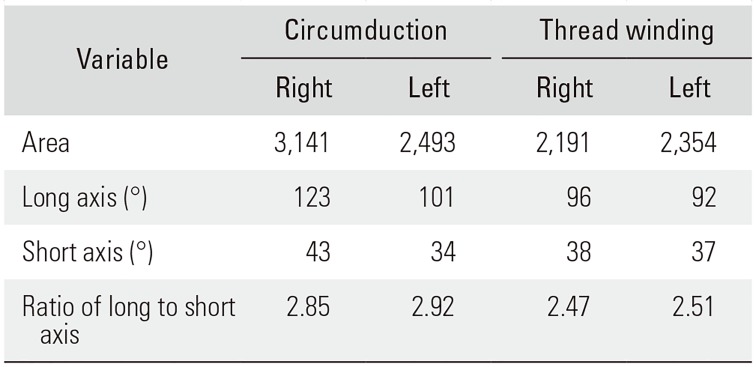
Variable | Circumduction | Thread winding | ||
---|---|---|---|---|
Right | Left | Right | Left | |
Area | 3,141 | 2,493 | 2,191 | 2,354 |
Long axis (°) | 123 | 101 | 96 | 92 |
Short axis (°) | 43 | 34 | 38 | 37 |
Ratio of long to short axis | 2.85 | 2.92 | 2.47 | 2.51 |
Table 4
Dart Throwing versus Hammering and Circumduction versus Thread Winding of the Right and Left Wrists
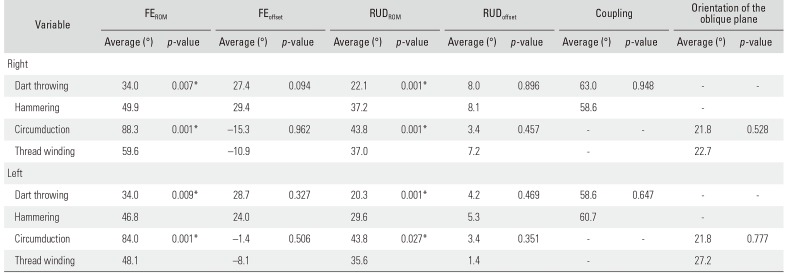
Table 5
Comparison of the Findings of the Current Study and Other Previous Stuidies: Dart Throwing
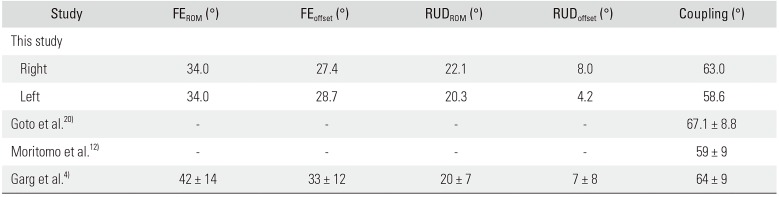
Study | FEROM (°) | FEoffset (°) | RUDROM (°) | RUDoffset (°) | Coupling (°) |
---|---|---|---|---|---|
This study | |||||
Right | 34.0 | 27.4 | 22.1 | 8.0 | 63.0 |
Left | 34.0 | 28.7 | 20.3 | 4.2 | 58.6 |
Goto et al.20) | - | - | - | - | 67.1 ± 8.8 |
Moritomo et al.12) | - | - | - | - | 59 ± 9 |
Garg et al.4) | 42 ± 14 | 33 ± 12 | 20 ± 7 | 7 ± 8 | 64 ± 9 |
Table 6
Comparison of the Findings of the Current Study and Other Previous Stuidies: Hammering
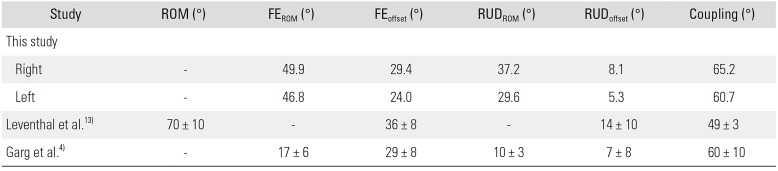
Study | ROM (°) | FEROM (°) | FEoffset (°) | RUDROM (°) | RUDoffset (°) | Coupling (°) |
---|---|---|---|---|---|---|
This study | ||||||
Right | - | 49.9 | 29.4 | 37.2 | 8.1 | 65.2 |
Left | - | 46.8 | 24.0 | 29.6 | 5.3 | 60.7 |
Leventhal et al.13) | 70 ± 10 | - | 36 ± 8 | - | 14 ± 10 | 49 ± 3 |
Garg et al.4) | - | 17 ± 6 | 29 ± 8 | 10 ± 3 | 7 ± 8 | 60 ± 10 |
Table 7
Comparison of the Findings of the Current Study and Other Previous Stuidies: Circumduction
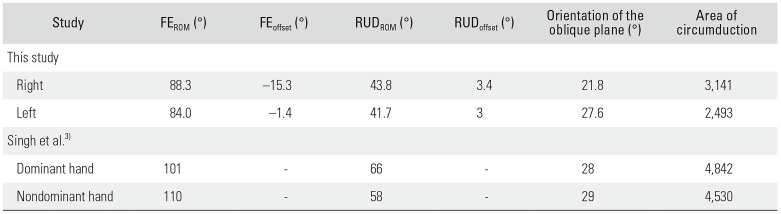
Study | FEROM (°) | FEoffset (°) | RUDROM (°) | RUDoffset (°) | Orientation of the oblique plane (°) | Area of circumduction |
---|---|---|---|---|---|---|
This study | ||||||
Right | 88.3 | −15.3 | 43.8 | 3.4 | 21.8 | 3,141 |
Left | 84.0 | −1.4 | 41.7 | 3 | 27.6 | 2,493 |
Singh et al.3) | ||||||
Dominant hand | 101 | - | 66 | - | 28 | 4,842 |
Nondominant hand | 110 | - | 58 | - | 29 | 4,530 |