Abstract
Background
MicroRNAs (miRNAs) have demonstrated their potential as biomarkers for lung cancer diagnosis. In recent years, miRNAs have been found in body fluids such as serum, plasma, urine and saliva. Circulating miRNAs are highly stable and resistant to RNase activity along with, extreme pH and temperatures in serum and plasma. In this study, we investigated serum miRNA profiles that can be used as a diagnostic biomarker of non-small cell lung cancer (NSCLC).
Methods
We compared the expression profile of miRNAs in the plasma of patients diagnosed with lung cancer using an miRNA microarray. The data from this assay were validated by quantitative real-time PCR (qRT-PCR).
Results
Six miRNAs were overexpressed and three miRNAs were underexpressed in both tissue and serum from squamous cell carcinoma (SCC) patients. Sixteen miRNAs were overexpressed and twenty two miRNAs were underexpressed in both tissue and serum from adenocarcinoma (AC) patients. Of the four miRNAs chosen for qRT-PCR analysis, the expression of miR-23a was consistent with microarray results from AC patients. Receiver operating characteristic (ROC) curve analyses were done and revealed that the level of serum miR-23a was a potential marker for discriminating AC patients from chronic obstructive pulmonary disease (COPD) patients.
MicroRNA (miRNA) is a non-coding, small, single- stranded, endogenous RNAs composed of 20~25 nucleotides that regulate interactions by inhibit messenger RNA translation or induce miRNAs cleavage1-3. Several reports suggested that aberrantly expressed miRNA may act as oncogenes or tumor suppressor genes in lung cancer4-6. miRNA regulates gene expression through sequence-specific interaction with 3'UTR in mRNAs2,3. Many genes have target sites for interaction with miRNAs and a single miRNA can modulate the expression of multiple genes7, hence the miRNA regulatory network is highly complex.
miRNA is known to circulate in a highly stable, cell-free form in the blood. Plasma and serum miRNA is resistant to RNase activity8,9. The similarity between circulating miRNA and tumor-derived miRNA patterns suggest that circulating miRNA might be useful as a biomarker10. Serum miR-21, miR-210, and miR-155 have been reported to be elevated in lymphoma patients; moreover, miR-21 expression is associated with relapsed- free survival11. Serum miR-141 can help distinguish patients with prostate cancer from healthy controls8. Thus, circulating miRNAs are useful as non-invasive biomarkers for cancers.
For this study, we hypothesize that serum miRNAs profiling could be used as to identify diagnostic biomarkers of non-small-cell lung cancer (NSCLC). To address this hypothesis, we screened serum miRNAs using a miRNA microarray. The results from this assay were validated with quantitative real-time polymerase chain reaction (qRT-PCR) analysis of individual serum samples.
Six sets of cancer tissues with matched non-cancerous lung tissue along with serum samples from six cancer patients (three squamous cell carcinoma [SCCs] and three adenocarcinoma [ACs]) and six serum healthy controls were used for the microarray. Eighteen serum samples from NSCLC patients (sevene SCCs and eleven AC) who were diagnosed at Konyang University Hospital were used as the training group for qRT-PCR. An additional 36 serum samples from NSCLC patients (19 SCCs and 17 AC) diagnosed at Kyungpook National University Hospital were used as the validation group for qRT-PCR (Table 1). Fifteen control serum samples were also collected from chronic obstructive pulmonary disease (COPD) patients for the qRT-PCR analysis. None of the patients had received chemotherapy or radiotherapy before sampling. All serum samples were stored at ??0℃ prior to use. Written, informed consent was obtained from each patient. This study was approved by the Bioethics Committee of Konyang University Hospital.
1) RNA extraction and cel-miRNA spike in: Total RNA containing small RNA was isolated from serum using Trizol reagent (Ambion Inc., Austin, TX, USA) according to the manufacturer's protocol with the following modifications. The Trizol reagent was mixed at a 3:1 ratio with serum. After the addition of chloroform, the tubes were shaken and centrifuged to separate the upper aqueous phase which was carefully transferred to a fresh tube. Isopropanol was then added to the aqueous phase followed by centrifugation at 12,000×g for 10 minutes. The precipitaqted RNA was then washed with 75% ethanol and centrifuged at 7,500 for 5 minute. Each sample was spiked with 2 ng of cel-miR-39 after adding phenol and guanidine thiocyanate to serve as an external processing control8,9.
2) miRNA microarry: The miRNA assay probes correspond to 470 well-annotated human miRNA sequences (miRBase version 9.1, February 2007; http://microrna. sanger.ac.uk/) and 265 miRNAs recently identified12. Assay probes were designed with a Tm of 60±8.6℃ and a length of 17~21 nt (average 18 nt). To maximize the specificity, candidate probes were collectively examined to minimize sequence similarity, particularly at the 3'-ends. Briefly, 15µL of the cDNA from the synthesis reaction was added to 5µL of the multiplexed MSO pool (MAP; Illumina Inc., San Diego, CA, USA). Next 30µL of a reagent containing streptavidin paramagnetic particles (OB1; Illumina Inc.) was added, then the mixture was heated to 70℃ and allowed to anneal at 40℃. All 735 human miRNAs were assayed simultaneously.
After binding and washing, the annealed MSOs were extended with the cDNA primer, forming an amplifiable product. The extended oligos were eluted from the streptavidin beads and added to a PCR reaction in which one of the universal primers was fluorescently labeled and the other universal primer was biotinylated. The PCR products were captured by streptavidin paramagnetic beads, washed, and denatured to yield single- stranded fluorescent molecules for hybridizing to the arrays. The universal arrays used for fluorescent reporting consisted of captured oligos immobilized on beads and randomly assembled into wells etched in a Sentrix universal bead chip (1536 bead array). The identity of each bead was determined before hybridization to the miRNA assay product, and the same arrays were used to report the results of similar assays using the address sequence technique (GoldenGate_Genotyping Assay, and DASL Gene Expression Assay, GoldenGate Methylation Assay). Arrays were scanned on the BeadArray Reader, and software for automatic image registration and intensity extraction was used to derive intensity data per bead type corresponding to each miRNA. The quality of hybridization and overall chip performance were monitored by visual inspection of both the internal quality control checks and raw scanned data. Raw data were extracted using the software provided by the manufacturer (Illumina BeadStudio version 3.1.3 (Gene Expression Module version 3.3.8). Array data were filtered by a detection p-value<0.05 (similar to signal to noise) for all samples. Selected gene signal values were transformed by a logarithm and normalized by the quantile method. Comparative analysis between the Test and Control groups was carried out using a t-test (adjusted Benjamini-Hochberg false discovery rate [FDR] was 5% controlled) and expressed as fold change. Hierarchical cluster analysis was performed using complete linkage and Euclidean distance as a measure of similarity. All data analysis and visualization of differentially expressed genes was conducted using ArrayAssist® (Stratagene, La Jolla, CA, USA) and R statistical language version 2.4.1.
Total RNA was extracted from each sample using Trizol reagent (Ambion Inc., Austin, TX, USA) according to the manufacturer's instructions. miRNA qRT-PCR analysis was performed in duplicate with a TaqMan MicroRNA assay kit (Applied Biosystems, Foster City, CA, USA) according to the manufacturer's instructions. The relative fold-changes of miRNAs expression were calculated by the standard curve method and cel-miR-39 was used for normalization.
SPSS version 12.0 software was used for statistical analysis (SPSS, Chicago, IL, USA). The optimal cut-off was chosen according to receiver operating characteristic (ROC) curve analysis. The area under the ROC curve (AUC) value was calculated to measure of the accuracy of the test.
We compared the miRNA profiles of lung cancer tissue versus non-cancerous lung tissues profiles, and those of serum from lung cancer patients versus serum from healthy controls. Six miRNAs were overexpressed and three miRNA were underexpressed in both tissue and serum from SCC patients (Table 2). 16 miRNAs were over-expressed and 22 miRNA were down-expressed on both tissue and serum of AC patients (Table 3).
Of the four miRNAs chosen for further analysis, two miRNA (miR-150 and miR-1268) were chosen in case of SCC and two miRNA (miR-23a and miR-1233) were chosen in AC cases. The miRNAs most differentially expressed in serum from patients with NSCLC versus the controls were selected based primarily on fold-change followed by p-values and false discovery rates (data not shown). Expression levels of miR-150 and miR-1268 shown by qRT-PCR were not consistent with the microarray results for cased of SCC (Figure 1A). Interestingly, the qRT-PCR results for mir-150 conflicted with those from microarray in both training and validation groups. For ACs, the results from qRT-PCR of miR-1233 was not consistent with the results from microarray for training and validation groups, but only the results from qRT-PCR of miR-23a were concordant with those from microarray for training and validation groups (Figure 1B). To evaluate whether serum miR-23a can be used as a potential marker for diagnosing ACs, ROC curve analyses were performed on all patients in training and validation group. These analyses revealed that the level of serum miR-23a was a potential marker for discriminating AC from COPD patients with a ROC curve area of 0.896 (95% CI, 0.75~0.97) (Figure 2). At a cut-off value of 0.35, the sensitivity and specificity for miR-23a was 75% and 92.7%, respectively.
The potential use of miRNAs as biomarkers for lung cancer has been demonstrated. Several studies have identified pathognomonic or tissue-specific miRNA expression profiles in lung cancer and other cancer5,13,14. Specific microRNA biomarkers may also accurately and reliably distinguish squamous from nonsquamous NSCLC. miR-205 is a highly accurate marker for lung cancer with squamous histology15. miRNAs regulate tumor activities such as tumor progression and metastasis; hence, miRNA profiling has been associated with clinical outcomes. The expression of let-7 is down-regulated in NSCLC patients and is associated with poor prognosis5,16. Saito et al.17 reported that high levels of miR-21 are associated with worse cancer-specific mortality and relapse-free survival rates independent of other clinical factors in stage I patients. Moreover, the expression of certain miRNAs is associated with chemoresistance in lung cancer18,19.
More recent methods have improved the threshold sensitivity of miRNA detection down to a few nanograms of total RNA9. This amount can easily be obtained in clinical specimens from fine needle aspiration biopsies (FNAB). The measurement of miRNA by qRT-PCR on FNAB samples has been reported20. miRNAs stability is high in both fresh and formalin-fixed paraffin-embedded tissues. This property makes miRNAs in clinical samples potentially useful diagnostic and prognostic biomarkers.
In recent years, miRNAs have been found in body fluids such as serum, plasma, urine, and saliva. Three different routes have been proposed to explain how miRNAs enter the circulation21. First, free miRNAs may be directly secreted by cells in a fashion similar to cytokines and hormones. Second, miRNAs may be selectively packed into microparticles and then released by cells via the shedding of microvesicles. Finally, miRNAs could be released via cell-derived exosomes.
Circulating miRNAs are highly stable and resistant to RNase activity as well as extreme pH and temperatures in serum and plasma8,22. The exact mechanism underlying the stability of circulating miRNAs remains largely unknown; however, a couple hypotheses have been proposed. First, miRNAs may be modified by methylation, adenylation, and uridylation. These modifications have a role of in stabilizing miRNAs23. Second, circulating miRNAs could be protected by specific proteins. RNA-binding protein could protect miRNA from degradation 24.
The results of this study showed that only the expression of miR-23a was consistent with the results of the microarray analysis. In contrast, the qRT-PCR results for miR-150, miR-1268 and miR-1233 was not consistent with those of the microarray analysis. There are two possible explanations for disagreement between the microarray and qRT-PCR results. First, the sample size for the microarray analysis was too small and may have resulted in false positives. Second, this discrepancy might be explained by the fact that these two methods used different control groups. The profile of circulating miRNAs could be also influenced by the presence of other diseases or even subtle physiological condition changes. Wang et al.25 reported that serum miR-146a and miR-223 levels are significantly reduced in septic patients compared to SIRS patients and healthy controls. In particular, the level of liver-specific miR-122 was elevated in plasma or serum from not only hepatocellular carcinoma patients but also individuals with hepatitis B virus infection, cirrhosis, and general liver injury26. These data suggested that the miRNAs profiles of healthy controls and COPD patinets might be different.
mioRNA expression analysis has found that the miR-23a family is up-regulated in various types of cancer 27-29. Additionally a previous study showed that miR-23a functions as an oncogene in gastric cancer, prostatic cancer and HCC. miR-23a promotes the growth of the gastric cancer and has anti-apoptotic effects on HCC cells through the regulation of target genes30,31. More importantly, the interleukin-6 receptor (IL-6R) tumor suppressor gene is directly regulated by miR-23a30.
The findings of this study have provided information about the serum miRNA profiles of lung cancer patients although only a small number of patients was examined. Moreover, miR-23a expression could be a potential serum-based biomarker used for minimally invasive procedures to diagnose AC. Additional large scale studies needed to validate the use of circulating miRNAs as novel biomarkers for lung cancer.
Figures and Tables
Figure 1
Box plot representing the expression of four miRNAs assessed by qRT-PCR. (A) Expression analysis to compare miR-150 and miR-1268 levels in the serum of SCC patients to serum samples obtained from COPD patients. (B) Expression analysis to compare miR-23a and miR-1233 levels in the serum of AC patients to serum samples obtained from COPD patients.
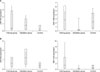
Figure 2
Receiver operating characteristic curves for miR23a in COPD control subjects and patients with adenocarcinoma. Curves were generated using data for miR-23a from qRT-PCR normalized to cel-miR-39.
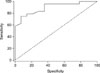
Acknowledgements
This work was supported by a Research Foundation Grant funded by the Korean Academy of Tuberculosis and Respiratory Disease (2009).
References
1. Ambros V. The functions of animal microRNAs. Nature. 2004. 431:350–355.
2. Bartel DP. MicroRNAs: genomics, biogenesis, mechanism, and function. Cell. 2004. 116:281–297.
3. Kim VN, Nam JW. Genomics of microRNA. Trends Genet. 2006. 22:165–173.
4. Volinia S, Calin GA, Liu CG, Ambs S, Cimmino A, Petrocca F, et al. A microRNA expression signature of human solid tumors defines cancer gene targets. Proc Natl Acad Sci USA. 2006. 103:2257–2261.
5. Yanaihara N, Caplen N, Bowman E, Seike M, Kumamoto K, Yi M, et al. Unique microRNA molecular profiles in lung cancer diagnosis and prognosis. Cancer Cell. 2006. 9:189–198.
6. Son JW, Kim YJ, Cho HM, Lee SY, Jang JS, Choi JE, et al. MicroRNA expression profiles in Korean non-small cell lung cancer. Tuberc Respir Dis. 2009. 67:413–421.
7. Lewis BP, Burge CB, Bartel DP. Conserved seed pairing, often flanked by adenosines, indicates that thousands of human genes are microRNA targets. Cell. 2005. 120:15–20.
8. Mitchell PS, Parkin RK, Kroh EM, Fritz BR, Wyman SK, Pogosova-Agadjanyan EL, et al. Circulating microRNAs as stable blood-based markers for cancer detection. Proc Natl Acad Sci USA. 2008. 105:10513–10518.
9. Kroh EM, Parkin RK, Mitchell PS, Tewari M. Analysis of circulating microRNA biomarkers in plasma and serum using quantitative reverse transcription-PCR (qRT-PCR). Methods. 2010. 50:298–301.
10. Lodes MJ, Caraballo M, Suciu D, Munro S, Kumar A, Anderson B. Detection of cancer with serum miRNAs on an oligonucleotide microarray. PLoS One. 2009. 4:e6229.
11. Lawrie CH, Gal S, Dunlop HM, Pushkaran B, Liggins AP, Pulford K, et al. Detection of elevated levels of tumour-associated microRNAs in serum of patients with diffuse large B-cell lymphoma. Br J Haematol. 2008. 141:672–675.
12. Berezikov E, van Tetering G, Verheul M, van de Belt J, van Laake L, Vos J, et al. Many novel mammalian microRNA candidates identified by extensive cloning and RAKE analysis. Genome Res. 2006. 16:1289–1298.
13. Rosenfeld N, Aharonov R, Meiri E, Rosenwald S, Spector Y, Zepeniuk M, et al. MicroRNAs accurately identify cancer tissue origin. Nat Biotechnol. 2008. 26:462–469.
14. Okies JE, Dietl C, Garrison HB, Starr A. Early and late results of resection of ventricular aneurysm. J Thorac Cardiovasc Surg. 1978. 75:255–260.
15. Lebanony D, Benjamin H, Gilad S, Ezagouri M, Dov A, Ashkenazi K, et al. Diagnostic assay based on hsa-miR-205 expression distinguishes squamous from nonsquamous non-small-cell lung carcinoma. J Clin Oncol. 2009. 27:2030–2037.
16. Takamizawa J, Konishi H, Yanagisawa K, Tomida S, Osada H, Endoh H, et al. Reduced expression of the let-7 microRNAs in human lung cancers in association with shortened postoperative survival. Cancer Res. 2004. 64:3753–3756.
17. Saito M, Schetter AJ, Mollerup S, Kohno T, Skaug V, Bowman ED, et al. The association of microRNA expression with prognosis and progression in early-stage, non-small cell lung adenocarcinoma: a retrospective analysis of three cohorts. Clin Cancer Res. 2011. 17:1875–1882.
18. Cho WC, Chow AS, Au JS. Restoration of tumour suppressor hsa-miR-145 inhibits cancer cell growth in lung adenocarcinoma patients with epidermal growth factor receptor mutation. Eur J Cancer. 2009. 45:2197–2206.
19. Garofalo M, Quintavalle C, Di Leva G, Zanca C, Romano G, Taccioli C, et al. MicroRNA signatures of TRAIL resistance in human non-small cell lung cancer. Oncogene. 2008. 27:3845–3855.
20. Mattie MD, Benz CC, Bowers J, Sensinger K, Wong L, Scott GK, et al. Optimized high-throughput microRNA expression profiling provides novel biomarker assessment of clinical prostate and breast cancer biopsies. Mol Cancer. 2006. 5:24.
21. Zen K, Zhang CY. Circulating microRNAs: a novel class of biomarkers to diagnose and monitor human cancers. Med Res Rev. 2010 Nov 9 [Epub]. DOI: 10.1002/med.20215.
22. Chen X, Ba Y, Ma L, Cai X, Yin Y, Wang K, et al. Characterization of microRNAs in serum: a novel class of biomarkers for diagnosis of cancer and other diseases. Cell Res. 2008. 18:997–1006.
23. Katoh T, Sakaguchi Y, Miyauchi K, Suzuki T, Kashiwabara S, Baba T, et al. Selective stabilization of mammalian microRNAs by 3' adenylation mediated by the cytoplasmic poly(A) polymerase GLD-2. Genes Dev. 2009. 23:433–438.
24. Wang K, Zhang S, Weber J, Baxter D, Galas DJ. Export of microRNAs and microRNA-protective protein by mammalian cells. Nucleic Acids Res. 2010. 38:7248–7259.
25. Wang JF, Yu ML, Yu G, Bian JJ, Deng XM, Wan XJ, et al. Serum miR-146a and miR-223 as potential new biomarkers for sepsis. Biochem Biophys Res Commun. 2010. 394:184–188.
26. Xu J, Wu C, Che X, Wang L, Yu D, Zhang T, et al. Circulating microRNAs, miR-21, miR-122, and miR-223, in patients with hepatocellular carcinoma or chronic hepatitis. Mol Carcinog. 2011. 50:136–142.
27. Scapoli L, Palmieri A, Lo Muzio L, Pezzetti F, Rubini C, Girardi A, et al. MicroRNA expression profiling of oral carcinoma identifies new markers of tumor progression. Int J Immunopathol Pharmacol. 2010. 23:1229–1234.
28. Vaksman O, Stavnes HT, Kaern J, Trope CG, Davidson B, Reich R. miRNA profiling along tumour progression in ovarian carcinoma. J Cell Mol Med. 2011. 15:1593–1602.
29. Gottardo F, Liu CG, Ferracin M, Calin GA, Fassan M, Bassi P, et al. Micro-RNA profiling in kidney and bladder cancers. Urol Oncol. 2007. 25:387–392.
30. Zhu LH, Liu T, Tang H, Tian RQ, Su C, Liu M, et al. MicroRNA-23a promotes the growth of gastric adenocarcinoma cell line MGC803 and downregulates interleukin-6 receptor. FEBS J. 2010. 277:3726–3734.
31. Huang S, He X, Ding J, Liang L, Zhao Y, Zhang Z, et al. Upregulation of miR-23a approximately 27a approximately 24 decreases transforming growth factor-beta-induced tumor-suppressive activities in human hepatocellular carcinoma cells. Int J Cancer. 2008. 123:972–978.