Abstract
Objective
Three-dimensional (3D) printing is a recent technological development that may play a significant role in orthodontic diagnosis and treatment. It can be used to fabricate skull models or study models, as well as to make replica teeth in autotransplantation or tooth impaction cases. The aim of this study was to evaluate the accuracy of fabrication of replica teeth made by two types of 3D printing technologies.
Methods
Fifty extracted molar teeth were selected as samples. They were scanned to generate high-resolution 3D surface model stereolithography files. These files were converted into physical models using two types of 3D printing technologies: Fused deposition modeling (FDM) and PolyJet technology. All replica teeth were scanned and 3D images generated. Computer software compared the replica teeth to the original teeth with linear measurements, volumetric measurements, and mean deviation measurements with best-fit alignment. Paired t-tests were used to statistically analyze the measurements.
Results
Most measurements of teeth formed using FDM tended to be slightly smaller, while those of the PolyJet replicas tended to be slightly larger, than those of the extracted teeth. Mean deviation measurements with best-fit alignment of FDM and PolyJet group were 0.047 mm and 0.038 mm, respectively. Although there were statistically significant differences, they were regarded as clinically insignificant.
Three-dimensional (3D) printing is a fast-developing technology that promises to play a significant role in orthodontic diagnosis and treatment. In cases of autotransplantation, 3D printing that is based on 3D computed tomography data can be used to fabricate a replica graft tooth, which simplifies the preparation procedure and shortens the extraoral time of the transplant tooth.12 Honda et al.3 explained the advantages of using a replica graft tooth to shorten the procedure time. Lee et al.4 also introduced a computer-aided rapid prototyping model in autotransplantation, and emphasized the importance of preventing unnecessary damage to a donor tooth that can occur during checking for fit in the prepared site. 3D printing technology introduces new methodology for diagnosing and treating tooth impaction cases. Faber et al.5 reported that 3D-printed dental models can be included in the diagnostic procedure for evaluation of impacted teeth. The model showed the exact anatomical relationship between the impacted tooth and the other teeth; it was the main aid in intraoperative navigation to expose the tooth.
There are a number of available 3D printers with the ability to print various objects using different printing technologies.6 The most commonly used 3D printers are solid-based fused deposition modeling (FDM) devices, where a thin plastic filament is used to lay down layers to build up a plastic object. Powder-based 3D printers such as Selective Laser Sintering use nylon or a similar thermoplastic powder that is locally melted with a laser beam. Recently, different liquid-based 3D printing technologies such as stereolithography apparatus (SLA) and digital light processing (DLP), and PolyJet have been introduced. Ultra violet (UV)-curable resin is polymerized to form the desired shape by light sources in these technologies.7
The application of 3D printing technology in orthodontic practices is increasing.89 However, before introducing 3D printing technology to new areas of orthodontics, its accuracy needs to be evaluated. Keating et al.10 compared two plaster models with their replicas created by stereolithographic 3D printing technology. The authors concluded that the mean difference in the vertical dimension was highly significant for the replica, probably because of the thick layers of clear resin (0.15 mm) from which it was built. They recommended that other 3D printing technologies such as DLP and PolyJet modeling, which use thinner layers, should be investigated. Cuperus et al.11 compared SLA replica models with the real dentition on several skulls, and found that the digital models also showed statistically significant differences, but they were regarded as clinically insignificant.
So far, only a few studies have reported the accuracy of dental replica models reconstructed by different 3D printing technologies. Kasparova et al.12 compared and contrasted the qualities of two 3D printing options-open source system FDM and commercially available SLA 3D printing. They reported that the commercially available SLA 3D printing demonstrated more details than the FDM system. Systematic difference, a problem in 3D printing, is a difference that is not determined by chance, but is introduced by an inaccuracy (as of observation or measurement) inherent in the system. Hazeveld et al.13 compared DLP, jetted photopolymer, and powder-based 3D printing technologies. Plaster models and the replica models reconstructed by DLP and jetted photopolymer had similar low mean systematic differences. The powder-based 3D printing replica models, however, had a higher mean systematic difference. The authors concluded that all replicas were accurate enough to be used interchangeably with plaster models, assuming that the difference should be considered more extensively in the interpretation stage.
Solid-based FDM technology and liquid-based PolyJet technology are two of the most advanced and effective 3D printing technologies available.14 Traditionally, FDM was common, but PolyJet device was recently developed with better quality and commercially introduced specifically for orthodontics (Objet30 Orthodesk®; Stratasys™, Eden Prairie, MN, USA). Comparing the two types of 3D printing technologies is essential to allow selection of the right technology for application in an orthodontic clinical situation. The aim of this study was to evaluate the accuracy of replica teeth made by two types of 3D printing technologies.
Fifty extracted molar teeth from the Department of Orthodontics of Wonkwang University Dental Hospital, South Korea, were randomly selected and served as the original teeth group. The inclusion criteria were as follows; (1) a complete permanent molar tooth, (2) normal crown morphology, (3) no restorations, and (4) no fractures of crown or root. They were soaked in hydrogen peroxide for three hours and their surfaces were cleaned. Numbers were given to each tooth in sequence from one to fifty.
The fifty original teeth were scanned to form high-resolution 3D surface datasets saved as surface model stereolithography (STL) files. We used a blue light-emitting diode scanner (Rexcan CS Plus®; Solutionix™, Bristol, UK). The scanner is optimized for scanning small- and medium-sized objects in a fully automated fashion. Its average error was 0.006 mm in a sphere-spacing error test. The resolution can be set to minimize stair stepping. Teeth were not fixed prior to scanning, and modifications to the images were minimized.
The STL files of fifty teeth were converted into physical models using two types of 3D printing technologies: FDM and PolyJet (Fortus250mc.® and Objet30 Pro®, respectively, both Stratasys™). Details of the machines and materials used in this study are presented in Table 1. The two types of 3D printing machine software process the STL file by generating slices of the model. The first layer of the physical model is created followed by successive layers, and this process is repeated until the whole model is constructed.
The extrusion process starts by extruding melted polymer from the nozzle system (extrusion die) and depositing it onto a structure. FDM begins with a software process, which processes STL files in minutes, mathematically slicing and orienting the model for the building process. If required, support structures can be automatically generated. The machine dispenses two materials-one for the model and one for a disposable supporting structure. FDM is based on an additive principle by accumulating materials in layers. A plastic filament or metal wire unwound from a coil supplies material to an extrusion nozzle which can turn the flow on and off. The nozzle is heated to melt the material and can be moved in both horizontal and vertical directions by a numerically controlled mechanism. The materials are deposited in layers as thin as 0.330 mm and the part is built from the bottom up-one layer at a time (Figure 1 [http://www.custompartnet.com/wu/fused-deposition-modeling]).
The principle of PolyJet is very similar to the printing process of an inkjet printer. But instead of jetting drops of ink onto paper, PolyJet 3D Printers jet layers of curable liquid photopolymer onto a build tray. Build-preparation software automatically calculates the placement of photopolymers and support material. The 3D printer jets and instantly UV-cures tiny droplets of liquid photopolymer. Fine layers accumulate on the build tray to create a precise 3D model or part. Where overhangs or complex shapes require support, the 3D printer jets a removable gel-like support material. The user easily removes the support materials by hand or with water. Models and parts are ready to handle and use right out of the 3D printer, with no post-curing needed (Figure 2 [http://www.3daddfab.com/technology]).
As with the original fifty teeth, numbers were written on the replica teeth with information marking them as made by FDM or PolyJet technology. 3D images of all replica teeth were also generated by the scanner (Rexcan CS Plus™).
Linear, volumetric, and mean deviation measurements were performed with commercial software (Geomagic Control® version 12; 3D Systems™, Rock Hill, SC, USA), in order to evaluate the fidelity of the replica teeth to the originals. The Geomagic Control® is a comprehensive inspection automation platform for streamlining in-line and repetitive inspection processes that use 3D scanners and other portable metrology devices. Its geometry calculation algorithms have been tested by America's National Institute of Standards and Technology and Britain's National Physical Laboratory and independently certified as Class 1 accuracy by Germany's National Metrology Institute (Physikalisch-Technische Bundesanstalt) metrology authority.
All linear measurements were taken with "best-fit alignment", which is overlapping of 3D images of the original tooth and the replica tooth by the software. The landmark points were manually determined by one observer and the distance was automatically calculated.
Using the software, the longest mesiodistal distance of the cross-sectional crown was automatically measured. The differences in crown width between the replica teeth and the original teeth were recorded.
Using the software, the distance between the highest cusp tip and the lowest root apex was automatically measured. The differences in tooth height between the replica teeth and the original teeth were recorded (Figure 3).
The Geomagic Control® was used to automatically measure the volume of each tooth. The ratios of each FDM and inkjet replica tooth volumes were compared to that of the corresponding original tooth.
The software was used to automatically measure the mean deviation of one hundred thousand points randomly designated with best-fit alignment. In each group, the mean deviation meant the absolute value of the outline discrepancy between the original teeth and the replica teeth.
The Geomagic Control® also provided color maps of all best-fit alignment images showing up to 0.2 mm deviation (Figure 4).
Paired t-tests were used to compare the accuracy of each of the two types of 3D printing, comparing the replica teeth to the original teeth.15 The measurements showed a normal distribution, confirmed with the Kolmogorov-Smirnov test and Shapiro-Wilk test.16 The intraobserver reliability of the repeated measurements was determined by the intraclass correlation coefficient (ICC) based on absolute agreement.17 For the analyses, commercial software (IBM SPSS Statistics®, version 21; IBM Co., Armonk, NY, USA) was used. The level of significance was set at p < 0.05.
The linear measurements of the extracted teeth, FDM replicas, and PolyJet replicas are presented in Table 2. The mean crown width of the FDM replicas was significantly smaller than that of the extracted teeth, with a mean difference of -0.116 mm. The mean crown width of the PolyJet replicas was significantly greater than that of the original group, with the mean difference being 0.064 mm. The mean tooth heights of both FDM and PolyJet replicas were smaller than that of the extracted teeth, but only the FDM replicas showed a statistically significant difference.
The volumetric measurements of all three groups are presented in Tables 3 and 4. Compared to the extracted teeth, most volumetric measurements of the FDM replicas showed significantly decreased values, while those of the PolyJet replicas showed significantly increased values. The volume ratio measurement of each replica tooth was calculated assuming that the volume measurement of an original tooth was 100%. Compared to the extracted teeth, the mean volume of the FDM replicas showed a 1.48% decrease and that of the PolyJet replicas showed a 0.71% increase. Both FDM and PolyJet replicas showed a statistically significant change in volume.
The comparison of mean deviation measurements with best-fit alignment is presented in Table 5. The mean value of 100,000 point deviations was 0.047 mm for the FDM replicas and 0.038 mm for the PolyJet replicas. The mean deviation measurements of the PolyJet replicas were statistically significantly 0.009 mm smaller than those of the FDM replicas.
Although automatic software can be used for measurements, the points positioned by an observer will affect the measured distance when crown width and tooth height are measured. One of the greatest sources of random error is the difficulty in identifying these landmarks.18 One observer made all measurements; therefore, only intraobserver variation was relevant. The ICC of 0.927 (95% confidence interval, 0.859-0.974) showed that the intraoperater reproducibility was high. The high ICC scores the system earned indicates that measurement error would not have significant influence on the outcome.17
The products made by the additive process of 3D printing will be influenced by the technology applied. These technologies can cause differences in final model dimensions because of material shrinkage during building or postcuring, and by the minimal thickness of the layers.19 All of these factors can have an effect on the accuracy of 3D printed models. The results from our study were similar to the study by Murugesan et al.20 The dimensions of controlled STL files were closer to the PolyJet replicas than to the FDM replicas. The thickness difference (FDM: 0.330 mm, PolyJet: 0.016 mm) of the layers may have affected the results.10 We also found that most measurements of the FDM replicas showed a decreasing tendency, while those of the PolyJet replicas showed an increasing tendency. We consider this to be caused by differences in material shrinkage. The exception of tooth height may be due to the inaccurate reconstruction of the complicated root apex.
No previous study has defined the clinically acceptable measurement differences between the replica teeth and natural teeth. However, several studies comparing plaster models with dental replica models reported that measurement differences of less than 0.25 mm are clinically acceptable because it is almost identical to the tolerances for manual measurements.13 In this study, mean deviations of the replica teeth manufactured with FDM and PolyJet methods were 0.047 mm and 0.038 mm, respectively. This means that both 3D printing technologies can be used clinically with very high accuracy. The PolyJet technology replicas showed statistically significant higher accuracy but the difference was not clinically significant. Some orthodontic treatments may be affected by the accuracy of 3D printer. For example, an inaccurate occlusal surface of a replica tooth can make an inaccurate transfer cap in indirect bonding.
Using the color map of best-fit alignment images, the errors most commonly seen were in the furcation area of the root, the occlusal groove, and the root apex in both sets of replicas. This is most likely due to the difficulty of obtaining an accurate scan image on these overlapping and complicated parts. Formatting the data into STL files may also have resulted in distortion, as data conversion and manipulation were necessary.21 Bibb et al.22 reported that since the light beam from structured light scanners travels in a straight line, any object surfaces that are obscured or are at too great an angle to the line of sight will not be scanned. This results in "voids" or "holes" in the scanned surface data. For irregular objects, multiple images of the same object should be acquired from different angles. The data from each of these scans can then be "stitched" together using a special software program that produces a single composite surface model.23
Murugesan et al.20 reported that scanning electron microscopy analysis showed a uniform smooth surface on PolyJet-generated models with adequate surface details. The authors stated that this is attributable to the jet of water which is used to remove supporting structures and hence provides a smooth surface. In our study, the PolyJet technology replicas also had smooth surfaces. Conversely, the surface of the FDM models was very rough, with layer patterns (Figure 5). It can be inferred that the differences between the maximum layer thickness of the two different technologies (FDM layer: 0.330 mm, PolyJet layer: 0.016 mm) was an another reason for different surface textures.
To our knowledge, this is the first study conducted comparing replica teeth to natural teeth. Future studies should evaluate other 3D printing methods with different 3D printing machines and materials.
The differences between original teeth and replica teeth constructed with FDM and PolyJet technologies were statistically significant, but they were well within clinically acceptable range. This study confirms that 3D-printed replica teeth manufactured with FDM and PolyJet technologies are usable in orthodontic clinical diagnosis and treatments requiring high accuracy.
References
1. Park YS, Jung MH, Shon WJ. Autotransplantion of a displaced mandibular second premolar to its normal position. Am J Orthod Dentofacial Orthop. 2013; 143:274–280. PMID: 23374935.


2. Shahbazian M, Wyatt J, Willems G, Jacobs R. Clinical application of a stereolithographic tooth replica and surgical guide in tooth autotransplantation. Virtual Phys Prototyp. 2012; 7:211–218.


3. Honda M, Uehara H, Uehara T, Honda K, Kawashima S, Honda K, et al. Use of a replica graft tooth for evaluation before autotransplantation of a tooth. A CAD/CAM model produced using dental-cone-beam computed tomography. Int J Oral Maxillofac Surg. 2010; 39:1016–1019. PMID: 20630704.


4. Lee SJ, Jung IY, Lee CY, Choi SY, Kum KY. Clinical application of computer-aided rapid prototyping for tooth transplantation. Dent Traumatol. 2001; 17:114–119. PMID: 11499760.


5. Faber J, Berto PM, Quaresma M. Rapid prototyping as a tool for diagnosis and treatment planning for maxillary canine impaction. Am J Orthod Dentofacial Orthop. 2006; 129:583–589. PMID: 16627189.


6. Stampfl J, Liska R. New materials for rapid prototyping applications. Macromol Chem Phys. 2005; 206:1253–1256.


7. Liu Q, Leu MC, Schmitt SM. Rapid prototyping in dentistry: technology and application. Int J Adv Manuf Technol. 2006; 29:317–335.


8. Azari A, Nikzad S. The evolution of rapid prototyping in dentistry: a review. Rapid Prototyp J. 2009; 15:216–225.


9. Kim JH, Kim KB, Kim WC, Kim JH, Kim HY. Accuracy and precision of polyurethane dental arch models fabricated using a three-dimensional subtractive rapid prototyping method with an intraoral scanning technique. Korean J Orthod. 2014; 44:69–76. PMID: 24696823.


10. Keating AP, Knox J, Bibb R, Zhurov AI. A comparison of plaster, digital and reconstructed study model accuracy. J Orthod. 2008; 35:191–201. PMID: 18809782.


11. Cuperus AM, Harms MC, Rangel FA, Bronkhorst EM, Schols JG, Breuning KH. Dental models made with an intraoral scanner: a validation study. Am J Orthod Dentofacial Orthop. 2012; 142:308–313. PMID: 22920696.
12. Kasparova M, Grafova L, Dvorak P, Dostalova T, Prochazka A, Eliasova H, et al. Possibility of reconstruction of dental plaster cast from 3D digital study models. Biomed Eng Online. 2013; 12:49. PMID: 23721330.


13. Hazeveld A, Huddleston Slater JJ, Ren Y. Accuracy and reproducibility of dental replica models reconstructed by different rapid prototyping techniques. Am J Orthod Dentofacial Orthop. 2014; 145:108–115. PMID: 24373661.


14. Stanek M, Manas D, Manas M, Navratil J, Kyas K, Senkerik V, et al. Comparison of different rapid prototyping methods. Intern J Math Comput Simul. 2012; 6:550–557.
15. Park HM. Comparing group means: T-tests and One-way ANOVA, Using Stata, SAS, R, and SPSS, Working Paper. Bloomington, IN: The University Information Technology Services Center for Statistical and Mathematical Computing, Indiana University;2009.
16. Lilliefors HW. On the Kolmogorov-Smirnov test for normality with mean and variance unknown. J Am Stat Assoc. 1967; 62:399–402.


17. Müller R, Büttner P. A critical discussion of intraclass correlation coefficients. Stat Med. 1994; 13:2465–2476. PMID: 7701147.


18. Bell A, Ayoub AF, Siebert P. Assessment of the accuracy of a three-dimensional imaging system for archiving dental study models. J Orthod. 2003; 30:219–223. PMID: 14530419.


19. Barker TM, Earwaker WJ, Lisle DA. Accuracy of stereolithographic models of human anatomy. Australas Radiol. 1994; 38:106–111. PMID: 8024501.


20. Murugesan K, Anandapandian PA, Sharma SK, Vasantha Kumar M. Comparative evaluation of dimension and surface detail accuracy of models produced by three different rapid prototype techniques. J Indian Prosthodont Soc. 2012; 12:16–20. PMID: 23449946.


21. Cheah CM, Chua CK, Tan KH, Teo CK. Integration of laser surface digitizing with CAD/CAM techniques for developing facial prostheses. Part 1: Design and fabrication of prosthesis replicas. Int J Prosthodont. 2003; 16:435–441. PMID: 12956501.
22. Bibb R, Freeman P, Brown R, Sugar A, Evans P, Bocca A. An investigation of three-dimensional scanning of human body surfaces and its use in the design and manufacture of prostheses. Proc Inst Mech Eng H. 2000; 214:589–594. PMID: 11201406.


23. Halazonetis DJ. Acquisition of 3-dimensional shapes from images. Am J Orthod Dentofacial Orthop. 2001; 119:556–560. PMID: 11343029.


Figure 3
Measurement of crown width and tooth height (mm). Measured, The length of printed tooth; nominated, the length of original tooth.
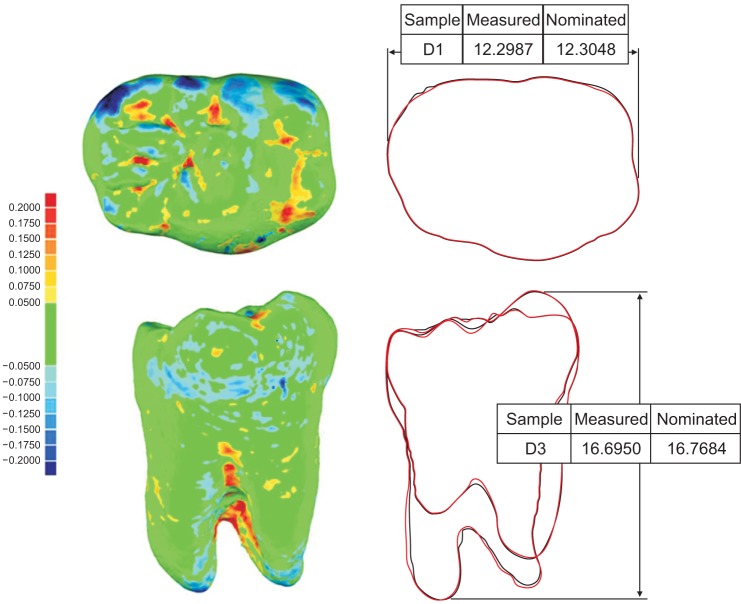
Figure 5
No. 1 original tooth (left) and replica teeth made with fused deposition modeling (middle) and PolyJet (right) three-dimensional printing technologies.
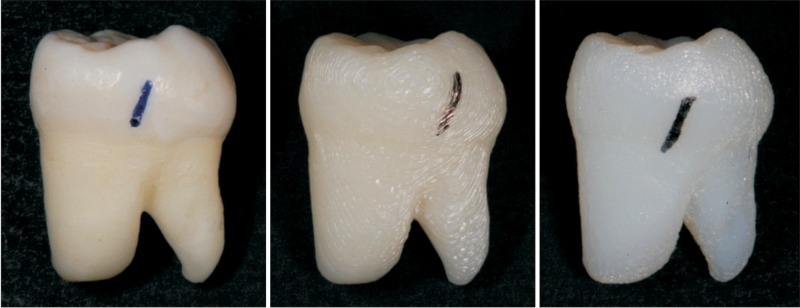
Table 1
Details of the FDM and PolyJet 3D printers and their model materials
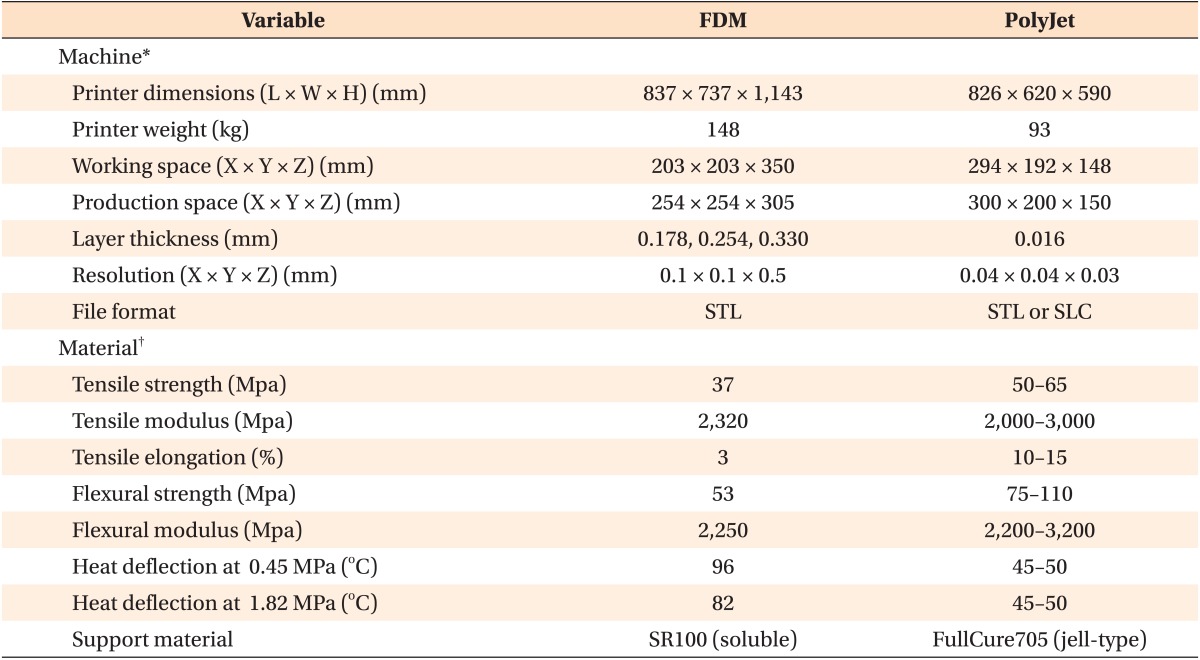
FDM, Fusion deposition modeling; 3D, three-dimensional; L, length; W, width; H, height; X, X-axis; Y, Y-axis; Z, Z-axis; ABS, acrylonitrile butadienestyrene; STL, stereolithography; SLC, single level cell.
*FDM: Fortus250mc™, PolyJet: Objet30 Pro™; †FDM: ABS plus-P430™, PolyJet: VeroWhitePlus™; all from Stratasys™, Eden Prairie, MN, USA.
Table 3
Volumetric measurements made on extracted teeth, FDM replicas, and PolyJet replicas (n = 50)
