Abstract
Objective
To evaluate the bioactivity, and the biomechanical and bone-regenerative properties of Ti6Al4V miniscrews subjected to anodization, cyclic precalcification, and heat treatment (APH treatment) and their potential clinical use.
Methods
The surfaces of Ti6Al4V alloys were modified by APH treatment. Bioactivity was assessed after immersion in simulated body fluid for 3 days. The hydrophilicity and the roughness of APH-treated surfaces were compared with those of untreated (UT) and anodized and heat-treated (AH) samples. For in vivo tests, 32 miniscrews (16 UT and 16 APH) were inserted into 16 Wistar rats, one UT and one APH-treated miniscrew in either tibia. The miniscrews were extracted after 3 and 6 weeks and their osseointegration (n = 8 for each time point and group) was investigated by surface and histological analyses and removal torque measurements.
Results
APH treatment formed a dense surface array of nanotubular TiO2 layer covered with a compact apatite-like film. APH-treated samples showed better bioactivity and biocompatibility compared with UT and AH samples. In vivo, APH-treated miniscrews showed higher removal torque and bone-to-implant contact than did UT miniscrews, after both 3 and 6 weeks (p < 0.05). Also, early deposition of densely mineralized bone around APH-treated miniscrews was observed, implying good bonding to the treated surface.
Anchorage control is very important for successful orthodontic treatment since unwanted tooth movement often leads to repeated interventions that increase the overall treatment time. In this context, skeletal anchors have recently gained acceptance as a viable alternative to extraoral devices for patients with insufficient dental support for anchorage or poor compliance towards extraoral devices.1,2,3,4
Miniscrews have many advantages compared with other skeletal anchors, such as their easy placement and removal, reduced cost, applicability at numerous anatomic locations, and ability to bear immediate loading forces, in addition to reduced discomfort following implantation.5,6 The success rate of miniscrews, however, is still not as high as that of conventional prosthodontic implants.7,8,9 Conventional prosthodontic implants are generally loaded after osseointegration, and are intended to be in place permanently. Orthodontic miniscrews, on the other hand, are usually loaded before osseointegration and then removed at the end of treatment. Therefore, one proven strategy to enhance the success rate of interventions using miniscrews is to rapidly achieve stability by inducing early osseointegration.1,4
Osseointegrated anchorage devices can not only withstand light continuous forces, but also heavy dynamic loads and rotational forces. Most clinicians however, prefer to avoid the trephine procedures required to place the anchor. Although concerns exist about the stability and risk of failure of surface-treated miniscrews, they are a viable and versatile option for orthodontic anchorage, and can easily be removed with low risk of fracture or distortion.1,2,3,4,8
The alloy Ti6Al4V has been considered for orthopedic applications as an alternative to pure Ti owing to its outstanding strength and heat resistance.6 In orthodontics however, Ti6Al4V alloy implants are known to release Al and V ions, causing cytotoxicity, hypersensitivity, increased risk of carcinogenesis, and the failure, ultimately, of the clinical implant.10,11 In this context, the objective of the study was to improve the biocompatibility and osseointegration capacity of Ti6Al4V, so that the advantages of this alloy can serve for better orthodontic anchorage.
To achieve faster osseointegration, the surface of Ti6Al4V samples was subjected to anodization, cyclic precalcification, and heat (APH) treatment. APH treatment involves modifying the surface of the sample on the nanoscale, and has been the focus of an increasing number of studies. It has notably been applied to pure Ti and Ti6Al7Nb alloys resulting in satisfactory bioactivity, biocompatibility, and osseointegration.12,13 Anodization creates an oxide layer of nanotube titania in an ordered array, leading to a larger surface area and a higher surface energy. These nanotubes have been shown to improve osteoblast activity and bone bonding, while the array can be used for the targeted delivery of various chemical, drugs and biomolecules. Together, these properties lead to reduced inflammation, side effects and toxicity from metal ion release.10,14,15,16 Furthermore, calcium phosphate infiltration into the nanotube space by precalcification treatment should promote biocompatibility and osteogenesis, and speed up osseointegration.
Studies of the relationship between anodization or precalcification surface treatment and osseointegration have focused so far on prosthetic implants,12,13 whereas the effects on miniscrews used as orthodontic anchors remain unclear. The purpose of this study is therefore to evaluate the bioactive, biomechanical, and osseoregenerative effects of APH treatment on Ti6Al4V orthodontic miniscrews.
Commercially available Ti6Al4V plates (10 × 10 × 2 mm; Kobe Steel Ltd., Kobe, Japan) and Ti6Al4V miniscrews (Φ 1.4 × 4 mm, n = 32; Jeil Medical Corp., Seoul, Korea) were used in this study. To produce a Ca-P coating and a nanotubular surface on the alloy, samples were subjected to APH treatment as previously described.13 Nanotubular arrays were formed by anodization at 20 V for 1 hour in a solution of Glycerol/H2O/NH4F (79, 20, and 1 wt% respectively). Ca-P particles were loaded into the arrays by cyclic pre-calcification, which involves soaking the samples repeatedly first in NaH2PO4 (0.05 M, 80℃) and then saturated Ca(OH)2 (100℃) for 1 min at a time. In this case, 30 cycles were completed before a final heat-treatment at 500℃ for 2 hours. Untreated (UT) specimens and TiO2 nanotubular (AH) specimens (which were subjected to anodization and heat-treatment, but not to cyclic pre-calcification) were prepared as control groups.
The topographical characteristics and chemical composition of the resulting surfaces were observed with a field emission scanning electron microscope (FE-SEM, S-800; Hitachi, Tokyo, Japan) equipped with an energy dispersive X-ray spectrometer (EDS; Bruker, Billerica, MA, USA). X-ray diffraction (XRD, Dmax III-A type; Rigaku, Tokyo, Japan) was used for phase analysis.
As a test of their bioactivity, the samples were immersed in SBF for 3 days while looking for hydroxyapatite (HA) precipitation. The SBF solution was prepared using Hanks' solution (H2387; Sigma-Aldrich Chemical Co., St. Louis, MO, USA), with addition of calcium chloride dihydrate 0.185 g/L, magnesium sulfate 0.09767 g/L, sodium hydrogen carbonate 0.35 g/L (pH 7.4). Changes in the surface microstructure were studied by FE-SEM and EDS.
The mean surface roughness (Ra) was obtained from three repeat measurements using a surface roughness tester (Surftest SV-3000; Mitutoyo Corporation, Kawasaki, Japan). The hydrophilic properties of the surfaces were evaluated by measuring the contact angle of 10 µL SBF solution dropped from 5 mm above the sample surface. Images were obtained by stereoscopic microscope (EZ4D; Leica Microsystems CMS GmbH, Wetzlar, Germany) and the contact angles were measured using Screen Protractor 4.0 (Iconico Inc., New York, NY, USA).
The study was conducted in compliance with the principles of the Helsinki Declaration and ethical clearance was obtained from the Institutional Animal Care and Use Committee of the Chonbuk National University Laboratory Animal Center (approval number: CBU 2012-0027). Experiments were conducted on the tibias of 16 male Wistar rats (250-280 g, 7 weeks old) using 16 UT and 16 APH-treated miniscrews. Miniscrew implantation surgery was performed under general anesthetic using 75 mL/100 g intramuscular zolazepam-tiletamine (Zoletil 50; Virbac, Carros, France) and additional local anesthetic was applied on the surgical site using 2% lidocaine with epinephrine (1:100,000). An incision of 1.0 cm length was made, and the bone surface of the tibia was surgically exposed with a periosteal elevator. The cortical bone was drilled with a 1.2 mm pilot drill bur (Jeil Medical Corp.) at 250 rpm under copious saline irrigation. Miniscrews were inserted into the medial region of the bilateral tibia diaphysis using a self-tapping process until the screw thread was completely implanted in the bone cortex. For each rat, one UT and one APH-treated miniscrew were placed on opposite tibias. The surgical wound was sealed by 3-0 suture silk. Postoperatively, antibiotics (amoxicillin, 6 mg/kg) and anti-inflammatories (nabumetone, 5 mg/kg) were administered orally. Eight rats were sacrificed, both at 3 and 6 weeks after insertion, with an overdose of thiopental (JW Pharmaceutical Corp., Seoul, Korea).
Note that in clinical practice, there are two approaches to loading miniscrews: self-drilling (without pilot drilling) and self-tapping (with pilot drilling).5,9,17,18 The advantages of the self-drilling method are its ease of use, low mechanical involvement, intensive bone-implant contact, and low thermal damage.5,18 However, it tends to cause more microdamage to the cortical bone than the self-tapping method,9,17 and because friction during insertion could have impaired the surfaces, these factors led us to follow the self-tapping approach.
The removal torque value (RTV) was measured at 3 and 6 weeks after insertion. The implant sites in the rat tibia were surgically exposed to the bone and examined after carefully removing any overgrowing bone and soft tissue. Removal torque tests were performed using a digital torque gauge (9810P; Aikoh Engineering Co. Ltd., Tokyo, Japan) with a precision of 0.1 N·cm (n = 6 for each group). After stabilizing the legs, the torque was increased incrementally by slowly rotating the gauge counterclockwise. The peak torque value was recorded by a single examiner when rupture occurred between the miniscrew and the bone. After this test, the extracted miniscrew surfaces were examined with FE-SEM and EDS.
Two miniscrew-containing tibias were removed from both the 3- and 6-week groups, fixed with 10% formalin, stained in Villanueva bone, dehydrated in a series of increasing concentrations of alcohol, and embedded in methyl methacrylate. Subsequently, the blocks were cut parallel to the miniscrew axis and ground to make 40-µm thick sections. The miniscrew-bone interface was examined and bone-implant contact (BIC%) was measured on 5 threads using an optical microscope (DM 2500M, Leica Microsystems CMS GmbH) equipped with the Leica Application Suite (version 1.6.0; Leica Microsystems AG, Heerbrugg, Switzerland) under 100× magnification. BIC% values were determined as the percentage of direct contact between mineralized bone and the miniscrew surface within each thread. Five threads from two miniscrews from both groups were chosen randomly for this evaluation.
After calculating the means and standard deviations of the contact angle, Ra, RTV, and BIC%, independent t-tests were used to compare those results between groups and measuring periods, with the threshold for statistical significance set at a p-value of 0.05. All statistical analyses were carried out using SPSS sortware ver. 12.0 (SPSS Inc., Chicago, IL, USA).
Figure 1A shows the shape of the Ti6Al4V miniscrews used in this study. After anodization, their surface consisted of a highly compact and ordered arrangement of nanotubes, as evidenced in Figure 1B and 1C. As described above, cyclic precalcification was performed in order to induce precipitation of apatite-like Ca-P on these nanotubes. Figure 2A shows that the HA precipitation obtained on nanotubular Ti6Al4V was dense and homogeneous. As shown in the side view (Figure 2B), not only did HA precipitate densely onto the surface layer, but it also filled out empty spaces in the nanotubes.
To evaluate surface bioactivity, the samples were immersed in SBF solution for 3 days. Figure 2C and 2D show that APH samples are densely covered in HA protuberances over the entire surface. Furthermore, EDS analysis revealed Ca and P concentrations of 29.78 ± 2.64 wt.% and 14.70 ± 0.97 wt.%, respectively, such that the Ca/P ratio (1.59) was close to that of HA (1.67). This suggests that APH surfaces accelerate the precipitation of HA, implying high bioactive ability. Likewise, XRD data, presented in Figure 3, reveal the presence of octacalcium phosphate and HA in the APH samples.
Table 1 shows that for all groups (UT, AH and APH), the surface roughness is inversely correlated with the contact angle. Moreover, the surface roughness, and thereby the hydrophilicity, is increased by AH and APH treatments with the latter showing the greater effect.
Figure 4 presents the RTVs of UT and APH groups that were measured 3 and 6 weeks after insertion. The torque values are higher for the APH group at both 3 and 6 weeks than for the UT group (4.8 ± 2.7 Ncm versus 2.3 ± 1.2 Ncm at 3 weeks; 8.4 ± 3.1 Ncm versus 4.1 ± 1.4 Ncm at 6 weeks; p < 0.05).
Subsequently, the surfaces of the extracted miniscrews were examined with FE-SEM and EDS. For the UT group, both after 3 weeks (Figure 5A(a)) and 6 weeks (Figure 5A(c)), the surface predominantly shows interface fracture patterns between new bone and miniscrews. In contrast, the surfaces of the APH group show cohesive fracture patterns within newly formed bone at both stages (Figure 5A(b) and 5A(d)). The Ca and P concentration levels for the UT group are low, and the Ca/P ratio decreased from 3 to 6 weeks after insertion, from 1.21 to 0.50. On the other hand, those for the APH group remain high with a Ca/P ratio of 1.45 at 6 weeks, similar to that of HA.
After 3 weeks, very little bone formation is observed in Figure 6A(a) for the UT miniscrews, with a very low BIC% value (8.25 ± 6.67%), meanwhile the surface of the APH-treated miniscrews, shown in Figure 6B(b), is almost entirely covered with newly formed bone and the BIC% is 84.00 ± 8.47% (p < 0.05). After 6 weeks, bone adhesion is enhanced for both groups, with BIC% values of 61.75 ± 12.81% for the UT group (Figure 6A(c)), and 91.50 ± 3.58% for the APH group (Figure 6A(d)). Significant bone formation therefore occurs for both groups (p < 0.05), but the APH surface shows better and faster ossointegration (p < 0.05). This suggests that APH treatment leads to faster osseointegration and stabilization of the miniscrew.
In this study, APH treatment of Ti6Al4V alloys was investigated as a possible method to improve the initial stability and the success rate of orthodontic miniscrews. The biocompatibility of APH-treated Ti6Al4V miniscrews was investigated by immersion in SBF, contact angle and removal torque tests, surface analysis, and by evaluating their osseointegration into rat tibias. The results demonstrate that APH treatment provides a bioactive surface for inducing early osseointegration leading to better initial stability.
The formation of bone-growth-related material such as Ca-P minerals is an important issue for prosthodontic implants.19 In order to induce nanoscale nucleation and growth of HA, cyclic precalcification was conducted by immersion of the nanotubes in calcium and phosphate-enriched fluids. APH treatment of Ti6Al4V alloys has been shown to enhance alloy bioactivity in SBF immersion tests through the formation of HA and HA-like precipitates such as octacalcium phosphates.13 Because the composition of SBF solution is very close to that of human blood plasma, this suggests that similar results can be achieved in vivo. Moreover, a coating of nanotubular structures incorporating Ca-P was employed to increase the surface area of the alloys.16,19 As well as increasing the surface roughness compared with UT and AH groups, the surface wettability was also improved, leading to enhanced cell reaction.20 Thereby, APH-treated Ti6Al4V surfaces form a biocompatible interface making the alloy an excellent material for osseointegrated miniscrews.
Biomechanical testing showed that APH-treated miniscrews were better osseointegrated than untreated ones. For the latter, no tissues had attached to the surface 3 weeks after surgery and only small amounts of Ca and P were present. Besides, the Ca/P ratio of 1.21 indicates that this is nothing more than the natural accumulation of Ca and P that occurs in the body. EDS analysis showed that the thin layer that formed subsequently on the UT samples had a low Ca-P concentration and was therefore probably unmineralized tissue. Conversely, the increased Ca-P content and Ca/P ratio found on the surface of extracted APH-treated miniscrews showed that bone mineralization was accelerated in these samples. Moreover, cohesive rather than interface fractures were found on APH-treated miniscrews indicating strong bonding between the new bone and the alloy surface, and higher BIC% values were recorded for the APH group than for the UT group at both early and late stages of healing. The results from surface analysis and bioactivity tests are therefore confirmed, both qualitatively and quantitatively, that bone formation is enhanced on APH surfaces. This is in good agreement with the results of other studies where surface-treated miniscrews displayed higher mechanical fixation than untreated miniscrews.1,2,3,21 The APH treatment we propose for Ti6Al4V miniscrews is therefore promising in view of clinical applications.
There have been other reports recently of modified miniscrews that improve orthodontic anchorage through enhanced osseointegration.2,22 However, the necessity of and actual gains from such modification strategies remain controversial. Sandblasted, large-grit, and acid-etched orthodontic mini-implants showed higher removal torque than machined ones, indicating higher osseointegration shortly after insertion.2 Furthermore, a study evaluating the removal torque of miniscrews in dogs concluded that miniscrews were partially osseointegrated very soon after loading.8 In contrast, Chen et al.11 reported that non-osseointegrated palatal implants showed almost the same anchorage effect as osseointegrated palatal implants, thereby suggesting that waiting for osseointegration may be unnecessary for orthodontic implants; and Wu et al.5 concluded that miniscrew stabilization in the bone under immediate loading is mainly obtained through mechanical retention rather than osseointegration. To resolve the conflict between these findings, this extended study of APH-treated miniscrews has confirmed the benefits of surface treatment and outlined a practical and efficient approach to produce surface-modified miniscrews.
APH treatment of Ti6Al4V alloys accelerates the formation of a surface layer of HA in SBF. Moreover, APH-treated Ti6Al4V miniscrews show remarkably enhanced bone formation and bone binding affinity upon insertion into rat tibias. APH-treated miniscrews therefore exhibit a bioactive and biocompatible surface that should improve the initial stability when used for orthodontic anchorage. Especially in situations where poor bone quality or immediate loading requires rapid recovery, the accelerated osseointegration afforded by APH-treated miniscrews should prove valuable in a clinical context.
Figures and Tables
Figure 1
Field emission scanning electron microscope images of Ti6Al4V miniscrews: (A) untreated, and (B, C) nanotubes formed on the surface; (B) top and (C) cross-sectional views.
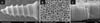
Figure 2
Ca-P coating on nanotubular Ti6Al4V miniscrews (A, B) after anodization, cyclic precalcification, and heat (APH) treatment and (C, D) after APH treatment and 3-day immersion in simulated body fluid. The arrow marks the interface between the nanotube layer and the newly formed crystal layer. The cross marks the point where the high magnification image (C) was taken.
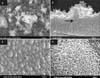
Figure 3
X-ray diffraction patterns of (A) UT, (B) AH, and (C) APH samples.
UT, Untreatment; AH, anodization and heat treatment; APH, anodization, cyclic precalcification and heat treatment; OCP, octa calcium phosphate; HA, hydroxyapatite; A, TiO2 anatase; Ti, titanium.
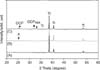
Figure 4
Removal torque values 3 and 6 weeks after miniscrew implantation. Asterisks (*) indicate significant differences between the groups (p < 0.05).
UT, Untreatment; APH, anodization, cyclic precalcification and heat treatment.
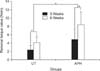
Figure 5
Morphology and chemical composition of the surface of extracted miniscrews. A, Field emission scanning electron microscope images of (a, c) UT and (b, d) APH samples in (a, b) 3 weeks and (c, d) 6 weeks after insertion. B, Corresponding results by energy dispersive X-ray spectrometer.
UT, Untreatment; APH, anodization, cyclic precalcification and heat treatment.
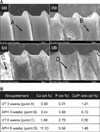
Figure 6
A, Histologic images (Villanueva staining, ×100) of the bone-miniscrew interface for (a, c) UT and (b, d) APH samples (a, b) in 3 weeks and (c, d) 6 weeks after insertion. B, Percentage of bone-implant contact (BIC%) measured on 5 threads of UT and APH-treated miniscrew interfaces. Values are presented as mean ± standard deviation.
*,†,‡Each marks indicate significant differences between groups (p < 0.05).
UT, Untreatment; APH, anodization, cyclic precalcification and heat treatment.
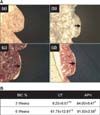
Notes
References
1. Ikeda H, Rossouw PE, Campbell PM, Kontogiorgos E, Buschang PH. Three-dimensional analysis of peri-bone-implant contact of rough-surface miniscrew implants. Am J Orthod Dentofacial Orthop. 2011; 139:e153–e163.


2. Kim SH, Lee SJ, Cho IS, Kim SK, Kim TW. Rotational resistance of surface-treated mini-implants. Angle Orthod. 2009; 79:899–907.


3. Chang CS, Lee TM, Chang CH, Liu JK. The effect of microrough surface treatment on miniscrews used as orthodontic anchors. Clin Oral Implants Res. 2009; 20:1178–1184.


4. Chaddad K, Ferreira AF, Geurs N, Reddy MS. Influence of surface characteristics on survival rates of mini-implants. Angle Orthod. 2008; 78:107–113.


5. Wu X, Deng F, Wang Z, Zhao Z, Wang J. Biomechanical and histomorphometric analyses of the osseointegration of microscrews with different surgical techniques in beagle dogs. Oral Surg Oral Med Oral Pathol Oral Radiol Endod. 2008; 106:644–650.


6. Suzuki EY, Suzuki B. Placement and removal torque values of orthodontic miniscrew implants. Am J Orthod Dentofacial Orthop. 2011; 139:669–678.


7. Wu TY, Kuang SH, Wu CH. Factors associated with the stability of mini-implants for orthodontic anchorage: a study of 414 samples in Taiwan. J Oral Maxillofac Surg. 2009; 67:1595–1599.


8. Chen Y, Kang ST, Bae SM, Kyung HM. Clinical and histologic analysis of the stability of microimplants with immediate orthodontic loading in dogs. Am J Orthod Dentofacial Orthop. 2009; 136:260–267.


9. Lee NK, Baek SH. Effects of the diameter and shape of orthodontic mini-implants on microdamage to the cortical bone. Am J Orthod Dentofacial Orthop. 2010; 138:8.e1–8.e8.


10. Morais LS, Serra GG, Muller CA, Andrade LR, Palermo EF, Elias CN, et al. Titanium alloy mini-implants for orthodontic anchorage: immediate loading and metal ion release. Acta Biomater. 2007; 3:331–339.


11. Chen F, Terada K, Hanada K, Saito I. Anchorage effect of osseointegrated vs nonosseointegrated palatal implants. Angle Orthod. 2006; 76:660–665.
12. Park IS, Yang EJ, Bae TS. Effect of cyclic precalcification of nanotubular TiO2 layer on the bioactivity of titanium implant. Biomed Res Int. 2013; 2013:293627.
13. Nguyen TDT, Park IS, Lee MH, Bae TS. Enhanced biocompatibility of a pre-calcified nanotubular TiO2 layer on Ti-6Al-7Nb alloy. Surf Coatings Technol. 2013; 236:127–134.


14. Eaninwene G 2nd, Yao C, Webster TJ. Enhanced osteoblast adhesion to drug-coated anodized nanotubular titanium surfaces. Int J Nanomedicine. 2008; 3:257–264.
15. Peng L, Mendelsohn AD, LaTempa TJ, Yoriya S, Grimes CA, Desai TA. Long-term small molecule and protein elution from TiO2 nanotubes. Nano Lett. 2009; 9:1932–1936.
16. Webster TJ, Ejiofor JU. Increased osteoblast adhesion on nanophase metals: Ti, Ti6Al4V, and CoCrMo. Biomaterials. 2004; 25:4731–4739.


17. Yadav S, Upadhyay M, Liu S, Roberts E, Neace WP, Nanda R. Microdamage of the cortical bone during mini-implant insertion with self-drilling and self-tapping techniques: a randomized controlled trial. Am J Orthod Dentofacial Orthop. 2012; 141:538–546.


18. Chen Y, Shin HI, Kyung HM. Biomechanical and histological comparison of self-drilling and self-tapping orthodontic microimplants in dogs. Am J Orthod Dentofacial Orthop. 2008; 133:44–50.


19. Oh SH, Finõnes RR, Daraio C, Chen LH, Jin S. Growth of nano-scale hydroxyapatite using chemically treated titanium oxide nanotubes. Biomaterials. 2005; 26:4938–4943.


20. Yao C, Perla V, McKenzie JL, Slamovich EB, Webster TJ. Anodized Ti and Ti6Al4V possessing nanometer surface features enhances osteoblast adhesion. J Biomed Nanotechnol. 2005; 1:68–73.


21. Salata LA, Burgos PM, Rasmusson L, Novaes AB, Papalexiou V, Dahlin C, et al. Osseointegration of oxidized and turned implants in circumferential bone defects with and without adjunctive therapies: an experimental study on BMP-2 and autogenous bone graft in the dog mandible. Int J Oral Maxillofac Surg. 2007; 36:62–71.

