Abstract
Objective
The purpose of this study was to investigate whether extension of the custom base is necessary for enhancement of bond strength, by comparing the debonding forces and residual adhesives of 3 different lingual bracket systems.
Methods
A total of 42 extracted upper premolars were randomly divided into 3 groups of 14 each for bonding with brackets having (1) a conventional limited resin custom base; (2) an extended gold alloy custom base: Incognito™; and (3) an extended resin custom base: KommonBase™. The bonding area was measured by scanning the bracket bases with a 3-dimensional digital scanner. The debonding force was measured with an Instron universal testing machine, which applied an occlusogingival shear force.
Results
The mean debonding forces were 60.83 N (standard deviation [SD] 10.12), 69.29 N (SD 9.59), and 104.35 N (SD17.84) for the limited resin custom base, extended gold alloy custom base, and extended resin custom base, respectively. The debonding force observed with the extended resin custom base was significantly different from that observed with the other bases. In addition, the adhesive remnant index was significantly higher with the extended gold alloy custom base.
Lingual orthodontic treatment is an alternative orthodontic treatment modality developed to overcome the unaesthetic nature of traditional orthodontic treatment. For efficient lingual orthodontic treatment, improved accuracy and reliability of indirect bonding is essential. To position brackets accurately, preparing a setup model for bracket positioning is now a routine procedure for lingual orthodontics.1 Because of the wide variation in the morphology of the lingual surface and the lingual arrangement of the dentition, the fabrication of an individual custom base for each lingual bracket is inevitable to minimize wire bending. Recently, various computer technologies have been applied and are being tested for these procedures.2,3
Several different methods have been introduced to fabricate the customized lingual bracket base. The conventional method makes use of a custom reference archwire to determine the position of the brackets. Gaps between the lingual surface of the tooth and bracket base are filled with bonding material to make the custom bracket base. Other methods use computerized 3-dimensional (3D) scanned data to design and manufacture the individualized custom bracket or bracket base. The last method is similar to the conventional method, in that the base material is extended to cover almost the entire lingual surface, so that it can be bonded directly.4
Although the efforts and developments in customizing lingual brackets have enabled easier bracket placement and more accurate positioning, few studies have investigated the bond strength of custom-base lingual brackets. In this study, 3 lingual bracket systems with different types of custom bases were tested, and the debonding force and residual adhesive were compared after debonding. The purpose of this study was to investigate whether the extension of the custom base was necessary to enhance the bond strength.
From among teeth extracted for orthodontic treatment, 42 upper premolars were selected for this study. Extracted teeth without restorations were stored in distilled water after cleaning the tooth surface. Teeth were excluded if careful examination revealed restorations, cracks, or surface defects. The selected teeth were randomly divided into 3 groups of 14 each.
The brackets were classified into groups 1, 2, and 3. Group 1 included brackets with a conventional limited resin base (Figure 1A). These lingual brackets had a limited surface area of resin custom base and were fabricated using a medium-viscosity light-cured resin (Light Bond Medium Paste; Reliance Orthodontic Products Inc., Itasca, IL, USA). Group 2 included brackets with an extended gold alloy base (Figure 1B) (Incognito™; TOP-Service für Lingualtechnik GmbH, Bad Essen, Germany). These were customized lingual brackets with an extended base area, which were manufactured by gold alloy casting based on computer-aided design using the 3D scanned data of a tooth model.2 Group 3 included brackets with an extended resin base (Figure 1C) (KommonBase™). These were lingual brackets with an extended surface area of resin custom base fabricated by flowable resin with 2 different viscosities (Gradia Direct Flo; Gradia Direct LoFlo, GC, Tokyo, Japan) and hard resin (Prossimo Add-On Gel, GC, Tokyo, Japan).4 The regular-sized conventional brackets in group 1 served as the control group for comparisons.
The teeth were aligned and planted on wax blocks and alginate impressions were taken to construct working models. The working models were sent to the laboratories for the fabrication of custom lingual brackets. In groups 1 and 3, the custom base was fabricated with a ready-made lingual bracket (STb; Ormco, Orange, CA, USA) after positioning, whereas group 2 brackets were molded as a single unit including the slot, body, and base.
The bracket base surface area was calculated from the 3D surface scanned data. A dental scanning system (3 series scanner, <20 µm; Dental Wings Inc., Montreal, Canada) was used for bracket base scanning. Scanned data were saved as STL files and surface area was measured in mm2 with 3D design software (SolidWorks 2012; Dassault Systems, Waltham, MA, USA).
Each tooth was cleaned and polished with pumice and a rubber cup. All teeth were thoroughly rinsed and dried before and after the etching of enamel with 37% phosphoric acid liquid (Etchant; Bisco Inc., Schaumburg, IL, USA) for 15 s. Before applying adhesive, the tooth was dried and the etched enamel surface was visually inspected to confirm a white chalky appearance. The resin base bonding surfaces for groups 1 and 3 were sandblasted by the laboratory (Arch Orthodontic Lab., Daegu, Korea). The gold alloy base for group 2 was sandblasted and coated with silane by the manufacturer. The bonding surfaces of all bracket bases were cleaned with acetone and dried before applying the adhesives.
Liquid bonding adhesive (Single Bond 2 Adhesive; 3M ESPE, St. Paul, MN, USA) was coated on the entire surface of the etched enamel with a brush and gently blown with compressed air to achieve a thin and even spread of liquid adhesive. It was then cured by a light-emitting diode (LED) source (≥1,600 mW/cm2, 420 - 490 mm; Good Doctors Co., Incheon, Korea) for 10 s. On the bonding surface of the bracket base, a low-viscosity flowable resin (CharmFil Flow, Blue; Dentkist Inc., Gunpo, Korea) was applied. The bracket was seated with firm pressure and the excess bonding resin extruding from the base was removed with an explorer. The bracket was light cured for 20 s from 3 directions: occlusal, mesial, and distal, with a total curing time of 60 s for each bracket. During the curing process, the light tip was kept as close as possible to the bond interface in a parallel orientation.
The teeth with bonded brackets were stored in distilled water for 1 week before debonding. One day before the test, the teeth were embedded in a 30 mm diameter × 20 mm high cylindrically shaped column made of white stone.
The debonding force of each bracket was measured in newtons (N) by the Instron universal testing machine (3343 Single Column Testing System; Instron, Canton, MA, USA). The samples were aligned with the bonding surface parallel to the shear blade (Figure 2). A debonding force was applied by the shear blade moving in an occlusogingival direction with a crosshead speed of 1 mm/min. The shear bond strength (N/mm2) was calculated by dividing the debonding force by the surface area of the bonding base.
After debonding of the bracket, the surfaces of teeth and bracket bases were examined to identify the location of bond failure. The residual adhesive resin on the bonded surface was assessed by the adhesive remnant index (ARI). The ARI was scored as follows: 0 = no bonding resin remaining on the tooth; 1 = less than 50% of bonding resin remaining on the tooth; 2 = more than 50% of bonding resin remaining on the tooth; 3 = all bonding resin remaining on the tooth.5
Descriptive statistics, including medians, means, and standard deviations (SD), were calculated. As these data were normally distributed and exhibited equal variances, a one-way analysis of variance (ANOVA) and Tukey's multiple comparison tests were used to determine the statistical significance of any intergroup differences in the mean debonding forces and shear bond strengths. The ARI was analyzed for percentage and frequency of fracture type, and a Kruskal-Wallis test with Bonferroni-corrected Mann-Whitney test was used to determine significant differences. The significance level was p = 0.05. All statistical analyses were performed using SPSS 14.0 for Windows (SPSS Inc., Chicago, IL, USA).
The mean base surface areas of the groups were 10.44 mm2 (SD 1.39) for the limited resin base, 32.60 mm2 (SD 2.40) for the extended gold alloy base, and 28.28 mm2 (SD 1.12) for the extended resin base (Table 1).
The debonding force for the limited resin base (group 1) ranged from 44.13 N to 78.69 N; the mean debonding force was 60.83 N (SD 10.12). For the extended gold alloy base (group 2), the debonding force ranged from 56.00 N to 86.00 N; the mean was 69.29 N (SD 9.59). For the extended resin base (group 3), the debonding force ranged from 81.90 N to 149.70 N; the mean was 104.35 N (SD 17.84). Statistical analysis indicated that the debonding force in group 3 was significantly higher than those in the other 2 groups (Table 2, Figure 3).
Shear bond strength for the limited resin base (group 1) ranged from 4.23 N/mm2 to 7.54 N/mm2; the mean bond strength was 5.83 N/mm2 (SD 0.97). For the extended gold alloy base (group 2), the debonding force ranged from 1.72 N/mm2 to 2.64 N/mm2 and the mean was 2.13 N/mm2 (SD 0.30). For the extended resin base (group 3), the debonding force ranged from 2.90 N/mm2 to 5.29 N/mm2 and the mean was 3.69 N/mm2 (SD 0.63). Statistical analysis indicated that the shear bond strengths of all groups were significantly different (Table 3 and Figure 4).
The ARI was significantly higher with the extended gold alloy custom base. Between the groups with bases fabricated with resin (groups 1 and 3), no significant difference was found in ARI scores. Most of the debonding occurred within the adhesive, whereas the extended gold alloy custom base showed significantly more bracket-adhesive fracture (Table 4).
During a debonding test procedure, many factors can affect the final result. Therefore, a thorough review of the literature6-8 was performed to standardize the test protocol.
Premolars are the most common teeth extracted for orthodontic treatment. Upper premolars are less diverse in shape and size than lower premolars. To ensure that the tooth samples had consistent bracket base morphology, only upper premolars were selected in this study. Various storage media have been used for orthodontic bonding tests. Storage media such as ethanol solutions have been shown to dehydrate the enamel surface and thereby decrease the bond strength.9 Storage in other water-based media, such as artificial saliva and distilled water, has also been reported to reduce the bond strength, but the effect on the enamel is not clearly known.6
A low-viscosity flowable resin with 57% filler content was used as the bonding adhesive in this study. To aid visual identification, blue-colored flowable resin was selected. Flowable resins with various filler contents have been reported to be acceptable for orthodontic bracket bonding.10,11 When flowable resins were compared with composite resin adhesives, no significant differences were reported.12,13 The low viscosity of the flowable resin allows minimal adhesive thickness and enhances flowability. As a result, the adhesive spreads out more, thereby improving the fit of the custom base to the tooth surface. In recent years, these advantages have made flowable resin the first choice of adhesive for light-cured indirect bonding techniques.
The duration of light exposure has been reported to be more important than light intensity in ensuring reliable bonding of light-cured brackets.14 Prolonged light exposure results in a higher bond strength, and a minimum period of light exposure is necessary for optimal bond strength.15,16 In procedures employing an LED light, a significant decrease in bond strength was observed when the distance from the light tip was increased.17,18 Therefore, in this study, to eliminate the effect of time and distance, the total light exposure time was 60 s, with the light tip in contact with the tooth from 3 directions.
Because the brackets in group 2 had a gold alloy base, the lack of polymerization due to a lack of light penetration through the base was thought to pose a problem. However, we tried to achieve adequate polymerization by employing a uniform bonding protocol, a light source fully accessible from every direction, and an extended duration of light exposure. Nevertheless, further tests with self-cured or dual-cured adhesives should be considered to avoid this potential problem.
Previous studies on indirect bonding techniques have reported no significant differences in various combinations of resin bases and adhesives.19-21 Several methods of intraoral positioning and bonding have been introduced for the lingual bracket systems tested in this study. Nonetheless, the brackets were directly bonded during the test to avoid the influence of fitting of the positioner and to improve the accessibility to light. Moreover, the light-cured adhesive was differentiated from the base resin by the use of adhesives with color.
According to a previous study,22 the average force transmitted to a bracket during mastication has been found to range from 40 N to 120 N. Hence, it would be reasonable to assume that there will be more bond failures if the bond force is below optimal, whereas more fractures of the enamel would occur if the force is too strong. The debonding forces of the brackets were 60.83 ± 10.12 N for the limited resin base, 69.29 ± 9.59 N for the extended gold alloy base, and 104.35 ± 17.84 N for the extended resin base. The results show that all the lingual bracket systems tested in this study can withstand occlusal and orthodontic forces and their bond strengths are in the optimal range. However, our conclusions were derived from an in vitro study and must be interpreted with caution when applying the results to the clinical setting.
For ease of positioning and to improve the fit of the lingual brackets, the custom bases of 2 lingual bracket systems were extended to follow the surface morphology and lingual borders of the teeth. As a result of the base extension, the bonding surface was consequently increased. We can reasonably assume that the extended bond surface area might lead to an increase in bonding force. Previous studies have shown an increase in bond strength when the base area is increased.23-25 In our study, no differences were found between the limited resin base and extended gold alloy base, or groups 1 and 2. The effect of bond area extension occurred only when the same base material, as shown from groups 1 and 3, were compared. Most of the previous studies compared labial brackets with similar materials or structures. In addition, there have been reports that demonstrated other factors, including the adaptability, retentive structures, or the chemical composition of the bond surface might be more critical to bond strength than the base area.23,26,27 The bond strength is calculated as a debonding force divided by a bonding surface area. Due to the large area of bracket bases tested in this study, the bond strengths were smaller than the values obtained in other studies, which were distributed from 5 to 25 MPa.6
For groups 1 and 3, most of the observations demonstrated an ARI score of 1 and 2. This indicated that the common site of bond failure was within the adhesives. In contrast, the most frequent ARI score from the extended gold alloy base group was 3, showing more adhesive-base failure, indicating a relatively weak adhesion between the adhesive and base material. Thus, when bonding a customized bracket with gold alloy, surface treatment of the bonding base is necessary to improve bond strength.
With regard to bond strength, the minimal extension of the custom base fabricated with resin adhesives appears to be satisfactory. In instances where base extension is required, a resin base will offer better bond strength.
The results suggest that the bonding force of 3 custom-base lingual brackets can withstand occlusal and orthodontic forces. However, an extended resin base showed significantly higher debonding force than a limited resin base or extended gold alloy base brackets. Comparing a limited resin base and an extended resin base, no significant difference was found in ARI scores and most of the debonding occurred within the adhesive. On the basis of these results, the authors conclude that effective bonding of lingual brackets can be obtained without extension of the custom base.
Figures and Tables
Figure 1
Three different types of custom-base lingual brackets used in this study. A, Group 1, limited resin base: conventional base; B, group 2, gold alloy base: Incognito™; C, group 3, extended resin base: KommonBase™.
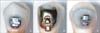
Figure 3
Box plots of debonding forces. A horizontal line inside the box indicates the median value of the samples within each group. The lower margin of the box indicates the middle value within data between minimum and median, whereas the upper margin of the box indicates the middle value within data between median and maximum. Upper and lower horizontal lines outside the box represent maximum and minimum values within a 1.5 interquartile range. A dot outside the whisker indicates an outlier, exceeding the 1.5 interquartile range. Differing superscripts indicate differences between groups according to Tukey's multiple comparison test at α = 0.05.
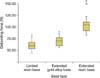
Figure 4
Box plots of shear bond strengths. A horizontal line inside the box indicates the median value of the samples within each group. The lower margin of the box indicates the middle value within data between minimum and median, whereas the upper margin of the box indicates the middle value within data between median and maximum. Upper and lower horizontal lines outside the box represent maximum and minimum values within a 1.5-interquartile range. A dot outside the whisker indicates an outlier, exceeding the 1.5 interquartile range. Differing superscripts indicate differences between groups according to Tukey's multiple comparison test at α = 0.05.
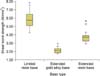
Table 4
Adhesive remnant index (ARI) score
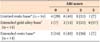
Values are presented as number (%).
was scored as follows: 0 = no bonding resin remaining on the tooth; 1 = less than 50% of bonding resin remaining on the tooth; 2 = more than 50% of bonding resin remaining on the tooth; 3 = all bonding resin remaining on the tooth.
Differing superscript letters indicate differences between groups according to Kruskal-Wallis and Mann-Whitney tests at α = 0.05.
Notes
References
1. Kyung HM. Individual indirect bonding technique (IIBT) using set-up model. Korean J Hist Dent. 1989; 27:73–82.
2. Wiechmann D. A new bracket system for lingual orthodontic treatment. Part 1: Theoretical background and development. J Orofac Orthop. 2002; 63:234–245.


3. Fillion D. Clinical advantages of the Orapix-straight wire lingual technique. Int Orthod. 2010; 8:125–151.


4. Komori A, Fujisawa M, Iguchi S. KommonBase for precise direct bonding of lingual orthodontic brackets. Int Orthod. 2010; 8:14–27.


5. Artun J, Bergland S. Clinical trials with crystal growth conditioning as an alternative to acid-etch enamel pretreatment. Am J Orthod. 1984; 85:333–340.


6. Finnema KJ, Ozcan M, Post WJ, Ren Y, Dijkstra PU. In-vitro orthodontic bond strength testing: a systematic review and meta-analysis. Am J Orthod Dentofacial Orthop. 2010; 137:615–622.


7. Stanford SK, Wozniak WT, Fan PL. The need for standardization of test protocols. Semin Orthod. 1997; 3:206–209.


8. Powers JM, Kim HB, Turner DS. Orthodontic adhesives and bond strength testing. Semin Orthod. 1997; 3:147–156.
9. Gittner R, Müller-Hartwich R, Jost-Brinkmann PG. Influence of various storage media on shear bond strength and enamel fracture when debonding ceramic brackets: an in vitro study. Semin Orthod. 2010; 16:49–54.


10. Park SB, Son WS, Ko CC, García-Godoy F, Park MG, Kim HI, et al. Influence of flowable resins on the shear bond strength of orthodontic brackets. Dent Mater J. 2009; 28:730–734.


11. Ryou DB, Park HS, Kim KH, Kwon TY. Use of flowable composites for orthodontic bracket bonding. Angle Orthod. 2008; 78:1105–1109.


12. D'Attilio M, Traini T, Di Iorio D, Varvara G, Festa F, Tecco S. Shear bond strength, bond failure, and scanning electron microscopy analysis of a new flowable composite for orthodontic use. Angle Orthod. 2005; 75:410–415.
13. Tecco S, Traini T, Caputi S, Festa F, de Luca V, D'Attilio M. A new one-step dental flowable composite for orthodontic use: an in vitro bond strength study. Angle Orthod. 2005; 75:672–677.
14. Mavropoulos A, Cattani-Lorente M, Krejci I, Staudt CB. Kinetics of light-cure bracket bonding: power density vs exposure duration. Am J Orthod Dentofacial Orthop. 2008; 134:543–547.


15. Silta YT, Dunn WJ, Peters CB. Effect of shorter polymerization times when using the latest generation of light-emitting diodes. Am J Orthod Dentofacial Orthop. 2005; 128:744–748.


16. Swanson T, Dunn WJ, Childers DE, Taloumis LJ. Shear bond strength of orthodontic brackets bonded with light-emitting diode curing units at various polymerization times. Am J Orthod Dentofacial Orthop. 2004; 125:337–341.


17. Cacciafesta V, Sfondrini MF, Scribante A, Boehme A, Jost-Brinkmann PG. Effect of light-tip distance on the shear bond strengths of composite resin. Angle Orthod. 2005; 75:386–391.
18. Sfondrini MF, Cacciafesta V, Scribante A, Boehme A, Jost-Brinkmann PG. Effect of light-tip distance on the shear bond strengths of resin-modified glass ionomer cured with high-intensity halogen, light-emitting diode, and plasma arc lights. Am J Orthod Dentofacial Orthop. 2006; 129:541–546.


19. Klocke A, Shi J, Kahl-Nieke B, Bismayer U. Bond strength with custom base indirect bonding techniques. Angle Orthod. 2003; 73:176–180.
20. Yi GK, Dunn WJ, Taloumis LJ. Shear bond strength comparison between direct and indirect bonded orthodontic brackets. Am J Orthod Dentofacial Orthop. 2003; 124:577–581.


21. Linn BJ, Berzins DW, Dhuru VB, Bradley TG. A comparison of bond strength between direct- and indirect-bonding methods. Angle Orthod. 2006; 76:289–294.
23. Cozza P, Martucci L, De Toffol L, Penco SI. Shear bond strength of metal brackets on enamel. Angle Orthod. 2006; 76:851–856.
24. MacColl GA, Rossouw PE, Titley KC, Yamin C. The relationship between bond strength and orthodontic bracket base surface area with conventional and microetched foil-mesh bases. Am J Orthod Dentofacial Orthop. 1998; 113:276–281.


25. Wang WN, Li CH, Chou TH, Wang DD, Lin LH, Lin CT. Bond strength of various bracket base designs. Am J Orthod Dentofacial Orthop. 2004; 125:65–70.

