This article has been corrected. See "ERRATUM: Expression of Erythropoietin in the Spinal Cord of Lewis Rats with Experimental Autoimmune Encephalomyelitis" in Volume 5 on page 105.
Abstract
Background and Purpose
Erythropoietin (Epo), originally recognized for its central role in erythropoiesis, has been shown to improve the outcomes in patients with various neurological disorders. The aim of this study was to elucidate the Epo expression pattern in the spinal cords of Lewis rats with experimental autoimmune encephalomyelitis (EAE) and to assess the systemic effect of Epo during the course of EAE.
Methods
We used an EAE model induced in Lewis rats by immunization with myelin basic protein. Immunized rats were given recombinant human Epo (rhEpo) intraperitoneally at a dose of 5,000 U/kg for 7 consecutive days, either starting on day 3 post-immunization (five rats) or on the day of clinical symptom onset (score ≥1, five rats). After immunization, the rats were observed daily for clinical signs of EAE. Epo expression was investigated by Western blot analysis and immunohistochemistry.
Results
Western blot analysis showed that, Epo expression was significantly elevated relative to control in the rat spinal cord during the peak stage of EAE (p<0.05), and then decreased thereafter. Immunohistochemistry demonstrated that Epo was expressed in some neurons and glial cells. Epo immunoreactivity was detected in ED1-positive macrophages and astrocytes in EAE lesions. Furthermore, we found that the intraperitoneal administration of rhEpo reduced both the disease severity and duration of paralysis in EAE rats, and reduced macrophage activity and increased Epo activity.
Erythropoietin (Epo) was initially identified as a hematopoietic growth factor and has been used extensively for the treatment of anemia in humans. Epo has recently received considerable attention due to the unexpected finding that it can exert broad neuroprotective effects in a variety of neurological disorders.1-3 The Epo receptor is widely expressed in most cerebral cell types, including neurons, microglial cells, and astrocytes.4 Epo and its receptor are required for normal brain development and the regulation of neurogenesis in the central nervous system (CNS).5,6 Neurons can produce Epo in response to hypoxia/ischemia, trauma, and immune-mediated inflammation.7-9 The increased immunoreactivity for Epo in the CNS of superoxide dismutase (SOD1) transgenic mice suggests interventional strategies to alleviate motor neuron degeneration in human amyotrophic lateral sclerosis.10 However, such immunoreactivity has not been investigated in rats with experimental autoimmune encephalomyelitis (EAE).
Multiple sclerosis (MS) is a chronic autoimmune disease of the CNS associated with demyelination, inflammation and axonal loss. Several cytokines, including anti-inflammatory cytokines and inhibitors of interleukin 1 (IL-1), and tumor necrosis factor (TNF), have shown some efficacy in reducing the severity of EAE, in an animal model of MS, but none have been shown to be clinically effective. Systemically administered Epo can delay the onset of EAE and decrease its clinical score.11,12 Epo also reduces axonal damage, inflammatory cell infiltration and demyelination, and blood-brain-barrier leakage in EAE.13 In addition, Epo exerts an anti-inflammatory effect on the CNS in EAE by delaying or reducing the increase in pro-inflammatory cytokines such as TNF-α, IL-6, IL-1β, and IL-1Ra.11,14
The mechanisms underlying the beneficial effects of Epo on the progression of EAE are not fully understood. In addition, little is known about the changes that take place in the Epo protein during EAE pathogenesis. The aim of this study was to elucidate the Epo expression pattern in the spinal cords of Lewis rats with EAE and to assess the systemic effects of Epo during the course of EAE.
Lewis rats were obtained from Harlan (Indianapolis, IN) and bred in our animal facility. Only male rats (7-8 weeks old; 160-200 g) were used in this study. All experiments followed the accepted ethical guidelines.
The footpads of both hind feet of rats in the EAE group were injected with 100 µL of an emulsion that contained equal parts of myelin basic protein (MBP)(1 mg/mL) and complete Freund's adjuvant (CFA) supplemented with Mycobacterium tuberculosis H37Ra (5 mg/mL)(Difco, Detroit, MI). Control rats were immunized with CFA alone. After immunization, the rats were observed daily for clinical signs of EAE. EAE progression was divided into seven clinical stages: Grade 0 (G.0), no signs; G.1, floppy tail; G.2, mild paraparesis; G.3, severe paraparesis; G.4, tetraparesis; G.5, moribund condition or death; and R.0, recovery.
Rabbit polyclonal anti-Epo antibody was obtained from Santa Cruz Biotechnology (Santa Cruz, CA). Mouse monoclonal anti-β-actin and mouse anti-glial fibrillary acidic protein (GFAP) were obtained from Sigma (St. Louis, MO). ED1 was obtained from Serotec (London, UK).
The rats were sacrificed under ether anesthesia. The spinal cords were dissected from rats in each group at 12-14 and 21 days post-immunization (PI)(n=5 rats/group), corresponding to the peak (G.3, day 12-14 PI) and recovery (R.0, day 21 PI) stages of EAE, respectively. Sections were cut on a cryostat in the transverse plane through the lumbar spinal cord, and every fifth section was selected for histochemistry against different antigens, or hematoxylin and eosin staining. Spinal cord samples were processed for embedding in paraffin wax after fixation in 4% paraformaldehyde in phosphate-buffered saline (PBS, pH 7.4). Additional spinal cord samples were snap-frozen and stored for immunoblot analysis.
Spinal cord tissue was homogenized in a modified radioimmunoprecipitation assay buffer {20 mM Tris, (pH 7.5), 150 mM NaCl, 1% Triton-X 100, 0.5% sodium deoxycholate, 0.1% sodium dodecyl sulfate, 1% NP-40, 10 mM NaF, 1 mM EDTA, 1 mM EGTA, 1 mM Na3VO4, 1 mM PMSF, 10 µg/mL aprotinin, and 10 µg/mL leupeptin} using 20 strokes in a homogenizer. The homogenate was transferred to microtubes and centrifuged at 14,000 rpm for 20 min, and the supernatant was harvested.
For the immunoblot assay, supernatant samples containing 40 µg of protein each were loaded into individual lanes of a 10% sodium dodecyl (lauryl) sulfate-polyacrylamide gel, electrophoresed, and immunoblotted onto nitrocellulose membranes (Schleicher and Schuell, Keene, NH). The residual binding sites on the membrane were blocked by incubation with 5% nonfat milk in Tris-buffered saline {TBS; 10 mM Tris-HCl (pH 7.4), and 150 mM NaCl} for 1 h. The membrane was then incubated with rabbit polyclonal anti-Epo (1 : 1,000 dilution) antibody for 2 h. The membranes were washed three times in TBS containing 0.1% Tween 20 and incubated with horseradish peroxidase-conjugated anti-mouse IgG (Vector, Burlingame, CA) for 1 h. Bound antibodies were detected using a chemiluminescent substrate (WEST-one™ Kit; iNtRON Biotech, Gyeonggi, Korea) according to the manufacturer's instructions. After imaging, the membranes were stripped and reprobed using anti-β-actin antibody. The optical density (per mm2) of each band was measured with a scanning laser densitometer (GS-700, Bio-Rad, Hercules, CA), and the obtained data are presented here as mean±SEM values. The ratios of the density of the Epo band relative to that of the β-actin band, were compared using Molecular Analyst software (Bio-Rad).
The data were analyzed using one-way analysis of variance (ANOVA) followed by the Student-Newman-Keuls post-hoc test for multiple comparisons. In all cases, p<0.05 was considered statistically significant.
Briefly, paraffin-embedded spinal cords (5-µm sections) were deparaffinized by treatment with citrate buffer (0.01 M, pH 6.0) in a microwave oven for 5 min. The sections were washed three times with PBS, and then treated with 0.3% hydrogen peroxide in methyl alcohol for 20 min to block endogenous peroxidase activity.
The sections were washed three washes with PBS, and then incubated with 10% normal goat serum followed by rabbit polyclonal anti-Epo. Immunoreactivity was visualized using the avidin-biotin peroxidase reaction (Vector Elite kit, Vector). Peroxidase was developed using a diaminobenzidine substrate kit (Vector). Sections were counterstained with hematoxylin before mounting. As a control, the primary antisera were omitted from a few test sections in each experiment.
Epo expression was examined using double immunofluorescence with cell-type-specific markers, including ED1 for monocyte-like macrophages, and anti-GFAP for astrocytes. The paraffin sections were deparaffinized, hydrated, washed three times with 0.1 M PBS, and incubated in the following order: 10% normal goat serum for 1 h at RT, rabbit polyclonal anti-Epo overnight at 4℃, and fluorescein isothiocyanate-labeled goat anti-rabbit IgG (Sigma) for 1 h at RT. The sections were then washed and blocked with 10% normal goat serum for 1 h at RT, incubated with either ED1 (mouse monoclonal anti-rat macrophages), or anti-GFAP overnight at 4℃, and washed and incubated with tetramethyl rhodamine isothiocyanate-labeled goat anti-mouse IgG (Sigma).
To minimize lipofuscin autofluorescence, the sections were washed three times in PBS at RT for 1 h each, dipped briefly in distilled H2O, treated with 10 mM CuSO4 in ammonium acetate buffer (50 mM CH3COONH4, pH 5.0) for 20 min, dipped briefly again in distilled H2O, and returned to PBS. The double immunofluorescence-stained specimens were examined with a laser confocal microscope (FV500, Olympus, Tokyo, Japan).
Immunized rats were given recombinant human Epo (rhEpo, 5,000 U/kg; Espogen, LG Life Sciences, Seoul, Korea) intraperitoneally for 7 consecutive days, either starting on day 3 PI (five rats) or on the day of clinical symptom onset (score ≥1, five rats). Control animals for rhEpo-treated EAE rats received vehicle (saline) alone from day 3 PI (five rats). In addition, three normal rats were injected intraperitoneally with rhEpo (5,000 U/kg), and three other normal rats received saline. The immunized rats were observed daily for clinical signs of EAE, and categorized according to the aforementioned stages. The progression of EAE was compared between rhEpo-treated and vehicle-treated control animals using Student's unpaired, two-tailed t-test. In all cases, p<0.05 was considered significant. To study the protein expression in rhEpo-treated EAE, the spinal cord from one rat per group was sampled on day 15 PI. The spinal cords were removed and analyzed according to the methods described above.
The level of Epo in the spinal cord was evaluated semiquantitatively by Western blot analysis during the course of EAE. Epo immunoreactivity was detected at low levels in the spinal cords of normal control rats (density, 0.118±0.03 OD/mm2; n=3). Epo immunoreactivity increased significantly in the spinal cord during the peak stage of EAE (G.3, day 12 PI; 0.298±0.017 OD/mm2; n=3; p<0.05 vs. controls) and subsequently decreased during the recovery stage (R0, day 21 PI; 0.206±0.002 OD/mm2; n=3; p<0.05 vs. peak stage of EAE). However, the expression of Epo during the recovery stage of EAE was still higher than the control level (p<0.05 vs. controls)(Fig. 1).
Epo was constitutively expressed in glial cells and neurons in the spinal cords of the normal control rats (Fig. 2A and B). During the peak stage of EAE (G.3, day 12 PI) there were many Epo-positive glial cells in the white matter of the spinal cord (Fig. 2C) and massive inflammatory cell infiltration into the parenchyma, where some round cells and neurons were positive for Epo (Fig. 2D). During the recovery stage (R0, day 21 PI), there were fewer inflammatory cells than during the peak stage of EAE, with only a few glial cells and neurons being positive for Epo (Fig. 2E and F). In addition, some Epo-positive vessels and ependymal cells were detected in the spinal cords of normal control rats and rats with EAE (data not shown).
The patterns of Epo immunofluorescence in the spinal cords of rats with EAE were similar to those seen with single immunoperoxidase staining (Fig. 2). Epo (Fig. 3A, green) was abundant in ED1-positive cells (Fig. 3B, red; Fig. 3C, merge), suggesting that most of the macrophages were positive for Epo in EAE lesions at the peak stage. In addition, Epo (Fig. 3D, green) was detected in GFAP-positive-astrocytes (Fig. 3E, red) in the white matter (Fig. 3F, merge).
To examine the effects of rhEpo on the course of EAE, immunized rats were injected intraperitoneally with rhEpo, and the duration of paralysis in rhEpo-treated rats was compared with that in control EAE rats treated with vehicle (vehicle treatment on day 3 PI; 6.5±0.29 days). The duration of paralysis was significantly reduced by rhEpo (early treatment, given on day 3 PI; 4.6±0.4 days; p<0.05 vs. vehicle), but there was no significant difference in the duration of paralysis between the rhEpo-treated and vehicle-treated rats on the day of clinical symptom onset (late treatment; 5.00±1.14 days). Furthermore, both the early and late rhEpo treatment significantly ameliorated the clinical severity of EAE in comparison with vehicle treatment (p<0.05)(Fig. 4A).
Western blot analysis of ED1 showed that immunoreactivity in the spinal cord was higher in the control EAE rats treated with vehicle on day 15 PI than in the normal control rats (Fig. 4B). ED1 immunoreactivity was lower in EAE-affected rats treated with rhEpo than in vehicle-treated rats (Fig. 4B). Epo immunoreactivity in the spinal cord was higher in the normal rats treated with rhEpo than in the normal rats treated with saline (Fig. 4B). As shown Fig. 1, Epo immunoreactivity in the spinal cord was higher in the control EAE rats treated with vehicle than in the normal rats treated with saline (Fig. 4B). However, the Epo expression did not differ significantly between the normal rats treated with rhEpo, the EAE rats treated with saline, and the EAE rats treated with rhEpo (Fig. 4B).
This is the first study to demonstrate that the level of Epo expression increases within the CNS during autoimmune inflammation. Based on the results of Western blot analysis of Epo expression, it is postulated that inflammatory cells and reactive astrocytes at the peak stage of EAE are important sources of Epo in the spinal cord of rats with EAE. The results of the present study also indicate that Epo expression increases significantly in the spinal cords of EAE-affected rats, which suggests that Epo plays a key role in the amelioration of EAE.
The present findings are in agreement with those of studies concerning Epo expression in ischemic brain injuries, showing that astrocytes are one of the main cell types expressing Epo.15,16 They suggest that Epo activates the neuronal Epo receptors and thus may inhibit hypoxia-induced apoptosis in neurons.15 In the present study, we found that the expression of Epo increased in reactive astrocytes during the peak stage of EAE, suggesting that astrocytes protects neurons under cytotoxic conditions.
In this study, we found that Epo was primarily localized in ED1-positive macrophages at the peak stage of EAE. This finding is very surprising in view of the general concept of macrophages in EAE. In response to stimuli, macrophages undergo a series of processes such as chemotaxis, phagocytosis, and the release of inflammatory mediators that damage neuronal networks.17 However, a previous study suggested that the production of several potent neurotrophic factors in leukocytes curbs the potentially neurodamaging consequences of severe CNS inflammation.18 Therefore, the production of Epo by activated macrophages might be an important mechanism for neuron protection in EAE.
The administration of rhEpo decreased the clinical severity of EAE, and this effect was paralleled by a marked decrease in macrophage activity in the spinal cord. However, there was no significant difference in Epo expression between EAE-vehicle and EAE-rhEpo treatments. As shown in Figs. 1-3, the activity of macrophages contributed to the increase in Epo immunoreactivity during the peak stage of EAE. Thus, we postulate that rhEpo transported across the blood-brain barrier into the spinal cord contributes to increased Epo expression. The onset of disease manifestation did not differ significantly between the early and late groups which we attribute to the use of an inadequate therapeutic dose and the timing of treatment initiation. Transferring this to the human disease where the starting point of autoimmunity can not be determined, it might be useful to apply neuroprotective treatment for longer than that the duration of acute neurological deterioration.
Increased Epo expression in EAE animals has not been demonstrated previously, and therefore the effect of its expression on the inflammatory events associated with EAE is unknown. One interesting possibility for the effects of Epo on inflammatory cells comes from Trzonkowski et al., finding that the intensity of apoptosis in both CD4+ and CD8+ T cells at baseline was lower in hemodialysis patients than in healthy volunteers, and that it increased with rhEpo treatment.19 In general, the initiation and termination of EAE pathogenesis involve T-cell and/or macrophage activation in the peripheral lymph nodes and CNS, and inflammatory cell apoptosis, respectively.20,21 Therefore, we do not exclude the possibility that the increased Epo expression at rhEpo administration or during the peak stage of EAE increases inflammatory cell apoptosis, which contributes to the suppression of EAE initiation or the acceleration of recovery.
In summary, we postulate that the increased expression of Epo in EAE rats is closely associated with neuron protection and the activation of inflammatory cell death, which leads to the prevention of or functional recovery from EAE paralysis.
Figures and Tables
Fig. 1
Western blot analysis of Epo expression in the spinal cord of rats with EAE. Spinal cord samples were collected from normal control rats, rats at the peak stage of EAE (G.3, day 12 PI), and rats during the recovery stage of EAE (R.0, day 21 PI). Representative photographs of Western blots; arrowheads indicate the expression of Epo and β-actin (upper). Semiquantitative analysis of Epo immunoreactivity in spinal cords, normalized to the intensity of β-actin expression in the same immunoblot. Epo was detected in the normal controls, and its expression was significantly increased in EAE-affected spinal cords. Data are (mean±SEM values) from three experiments (lower), *p<0.05 vs. normal controls and the recovery stage of EAE, †p<0.05 vs. normal controls. PI: post-immunization, Epo: erythropoietin, EAE: experimental autoimmune encephalomyelitis.
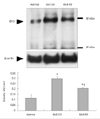
Fig. 2
Immunohistochemical staining of Epo in the spinal cords of normal control rats (A and B) and rats at the peak (day 12 PI)(C and D) and recovery (day 21 PI)(E and F) stages of EAE. Epo was weakly detected in some glial cells (A, arrows) and neurons (B, arrows) in the spinal cords of normal control rats. Glial cells at the peak stage of EAE showed increased immunoreactivity for Epo (C, arrows). Epo-positive inflammatory cells (D, arrowheads) and neurons (D, arrow) were detected in the parenchyma. At the recovery stage of EAE, some inflammatory cells (E, arrowhead), glial cells (E, arrows) and neurons (F, arrows) were positive for Epo. Tissue from a rat at the peak stage of EAE stained without the primary antisera showed no staining (G). Counterstaining was with hematoxylin. Scale bars=40 µm. Epo: Erythropoietin, PI: post-immunization, EAE: experimental autoimmune encephalomyelitis.
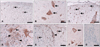
Fig. 3
Immunofluorescent co-localization of Epo (A and D) with ED1 (B) and anti-GFAP (E) in the spinal cords of rats at the peak stage of EAE (day 12 PI). A large amount of Epo (A, green; arrows) was immunostained in ED1-positive macrophages (B, red; arrows)(C, merge; arrows). Epo immunoreactivity (D, green; arrow) was co-localized in GFAP (E, red; arrow)-positive astrocytes (F, merge; arrow). Scale bars: A-F, 20 µm. Epo: Erythropoietin, anti-GFAP: anti-glial fibrillary acidic protein, EAE: experimental autoimmune encephalomyelitis, PI: post-immunization.
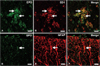
Fig. 4
Effect of recombinant human erythropoietin (rhEpo) therapy on the clinical course and protein expression in EAE. A: Rats were immunized with myelin basic protein and injected with saline (vehicle), rhEpo (5,000 U/kg) from day 3 PI (early group), or rhEpo from the day of clinical symptom onset (late group). Rats were scored daily for clinical signs of EAE. Data are the mean±SEM values from five animals per group. B: Western blot analysis of ED1 and Epo expressions in the Epo-treatment experiment. Spinal cord samples were collected from normal rats that were injected with saline (lane 1), rhEpo (lane 2), and from EAE-affected rats (day 15 PI) that were injected with vehicle (lane 3), rhEpo from day 3 PI (lane 4), and Epo from the day of clinical symptom onset (lane 5). *p<0.05 vs. vehicle. EAE: experimental autoimmune encephalomyelitis, PI: post-immunization.
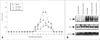
Acknowledgments
This work was supported by a grant from the Cheju National University Hospital Research Fund (2006).
The authors thank Heechul Kim for technical assistance.
References
1. Sirén AL, Ehrenreich H. Erythropoietin--a novel concept for neuroprotection. Eur Arch Psychiatry Clin Neurosci. 2001. 251:179–184.
2. Buemi M, Cavallaro E, Floccari F, Sturiale A, Aloisi C, Trimarchi M, et al. The pleiotropic effects of erythropoietin in the central nervous system. J Neuropathol Exp Neurol. 2003. 62:228–236.


3. Brines M, Cerami A. Emerging biological roles for erythropoietin in the nervous system. Nat Rev Neurosci. 2005. 6:484–494.


5. Shingo T, Sorokan ST, Shimazaki T, Weiss S. Erythropoietin regulates the in vitro and in vivo production of neuronal progenitors by mammalian forebrain neural stem cells. J Neurosci. 2001. 21:9733–9743.


6. Yu X, Shacka JJ, Eells JB, Suarez-Quian C, Przygodzki RM, Beleslin-Cokic B, et al. Erythropoietin receptor signaling is required for normal brain development. Development. 2002. 129:505–516.


7. Brines ML, Ghezzi P, Keenan S, Agnello D, de Lanerolle NC, Cerami C, et al. Erythropoietin crosses the blood-brain barrier to protect against experimental brain injury. Proc Natl Acad Sci U S A. 2000. 97:10526–10531.


8. Chung YH, Kim SI, Joo KM, Kim YS, Lee WB, Yun KW, et al. Age-related changes in erythropoietin immunoreactivity in the cerebral cortex and hippocampus of rats. Brain Res. 2004. 1018:141–146.


9. Wang L, Zhang Z, Wang Y, Zhang R, Chopp M. Treatment of stroke with erythropoietin enhances neurogenesis and angiogenesis and improves neurological function in rats. Stroke. 2004. 35:1732–1737.


10. Chung YH, Joo KM, Kim YS, Lee KH, Lee WB, Cha CI. Enhanced expression of erythropoietin in the central nervous system of SOD1 (G93A) transgenic mice. Brain Res. 2004. 1016:272–280.


11. Agnello D, Bigini P, Villa P, Mennini T, Cerami A, Brines ML, et al. Erythropoietin exerts an anti-inflammatory effect on the CNS in a model of experimental autoimmune encephalomyelitis. Brain Res. 2002. 952:128–134.


12. Zhang J, Li Y, Cui Y, Chen J, Lu M, Elias SB, et al. Erythropoietin treatment improves neurological functional recovery in EAE mice. Brain Res. 2005. 1034:34–39.


13. Li W, Maeda Y, Yuan RR, Elkabes S, Cook S, Dowling P. Beneficial effect of erythropoietin on experimental allergic encephalomyelitis. Ann Neurol. 2004. 56:767–777.


14. Savino C, Pedotti R, Baggi F, Ubiali F, Gallo B, Nava S, et al. Delayed administration of erythropoietin and its non-erythropoietic derivatives ameliorates chronic murine autoimmune encephalomyelitis. J Neuroimmunol. 2006. 172:27–37.


15. Ruscher K, Freyer D, Karsch M, Isaev N, Megow D, Sawitzki B, et al. Erythropoietin is a paracrine mediator of ischemic tolerance in the brain: evidence from an in vitro model. J Neurosci. 2002. 22:10291–10301.


16. Sirén AL, Knerlich F, Poser W, Gleiter CH, Brück W, Ehrenreich H. Erythropoietin and erythropoietin receptor in human ischemic/hypoxic brain. Acta Neuropathol. 2001. 101:271–276.


17. Rotshenker S. Microglia and macrophage activation and the regulation of complement-receptor-3 (CR3/MAC-1)-mediated myelin phagocytosis in injury and disease. J Mol Neurosci. 2003. 21:65–72.


18. Hammarberg H, Lidman O, Lundberg C, Eltayeb SY, Gielen AW, Muhallab S. Neuroprotection by encephalomyelitis: rescue of mechanically injured neurons and neurotrophin production by CNS-infiltrating T and natural killer cells. J Neurosci. 2000. 20:5283–5291.


19. Trzonkowski P, Debska-Slizien A, Szmit E, Myśliwska J, Szymańska K, Hak Ł, et al. Long-term therapy with recombinant human erythropoietin increases CD8+ T-cell apoptosis in haemodialysis patients. Nephrol Dial Transplant. 2005. 20:367–376.

