Abstract
Purpose
To explore the influence of S100 calcium binding protein A4 (S100A4) knockout (KO) on methionine-choline-deficient (MCD) diet-induced non-alcoholic fatty liver disease (NAFLD) in mice.
Materials and Methods
S100A4 KO mice (n=20) and their wild-type (WT) counterparts (n=20) were randomly divided into KO/MCD, Ko/methionine-choline-sufficient (MCS), WT/MCD, and WT/MCS groups. After 8 weeks of feeding, blood lipid and liver function-related indexes were measured. HE, Oil Red O, and Masson stainings were used to observe the changes of liver histopathology. Additionally, expressions of S100A4 and proinflammatory and profibrogenic cytokines were detected by qRT-PCR and Western blot, while hepatocyte apoptosis was revealed by TUNEL staining.
Results
Serum levels of aminotransferase, aspartate aminotransferase, triglyceride, and total cholesterol in mice were increased after 8-week MCD feeding, and hepatocytes performed varying balloon-like changes with increased inflammatory cell infiltration and collagen fibers; however, these effects were improved in mice of KO/MCD group. Meanwhile, total NAFLD activity scores and fibrosis were lower compared to WT+MCD group. Compared to WT/MCS group, S100A4 expression in liver tissue of WT/MCD group was enhanced. The expression of proinflammatory (TNF-α, IL-1β, IL-6) and profibrogenic cytokines (TGF-β1, COL1A1, α-SMA) in MCD-induced NAFLD mice were increased, as well as apoptotic index (AI). For MCD group, the expressions of proinflammatory and profibrogenic cytokines and AI in KO mice were lower than those of WT mice.
Non-alcoholic fatty liver disease (NAFLD) has been increasingly recognized as the most common chronic liver disease owing to excessive lipid accumulation in hepatocytes, leading to hepatocyte apoptosis, fat denaturalization, and the progression of hepatic fibrosis.1 For some cases, this disease often included a spectrum of histological changes, from simple steatosis to non-alcoholic steatohepatitis (NASH), which may develop into liver fibrosis, cirrhosis, and even hepatocellular carcinoma.2 With great changes in lifestyle and diet, as well as improvement of people's living standards, NAFLD incidence is gradually rising with a trend in younger ages, which has become one of the most prevalent chronic diseases in China after chronic viral hepatitis.3 Generally, NAFLD, accompanied with obesity, diabetes, hyperlipidemia, hypertension, and metabolic syndrome, is well-regarded as the hepatic manifestation of metabolic syndrome.4 Although effective therapies are absent due to inadequate elucidation of NAFLD pathogenesis, many factors such as immunity, genetics, metabolism, and environment are linked to the presence of NAFLD.5
S100 protein, a low molecular weight acidic protein (10–12 kDa), is reported to play a crucial role in many human diseases, and it controls multiple processes like apoptosis, inflammation, and cell movement.6 A total of 25 calcium-binding S100 family members, namely S100A1–18, hair hyaluronin, keratin fibrin, repetin, S100B, S100P, S100Z, and S100G, have been currently identified.7 As indicated by Mukai, et al.,8 S100A8 could induce the upregulation of tumor necrosis factor-alpha (TNF-α) in CXCR2-expressing CD11b+Gr-1high cells, thereby aggravating hepatitis in mice. In addition, Liu, et al.9 also found that expression levels of S100A9 was significantly elevated in patients with non-alcoholic fatty liver, which were suggested to be even higher in patients with NASH, highlighting the involvement of S100 protein in NAFLD progression. S100 calcium binding protein A4 (S100A4), another member of the S100 protein family, also known as Mts1, metastasin, p9Ka, pEL98, CAPL, and calvasculin, Fsp-1, placental calcium-binding protein, is a polypeptide comprised of 101 amino acids with a molecular weight of 11.5 kDa.10 Until now, existing studies on S100A4 mainly focused on its effects on tumors, like reducing intercellular adhesion, remodeling extracellular matrix, and promoting abnormal cell proliferation and angiogenesis, ultimately enhancing tumor cell invasion and migration.11 Moreover, S100A4 is known as an inducer of inflammatory processes, and its expression is strongly upregulated in various inflammatory diseases such as rheumatoid arthritis,12 idiopathic inflammatory myopathies13 and so on. In addition, there has been evidence stating that S100A4 levels in liver tissues were positively correlated with liver fibrosis.14 All factors mentioned above showed a possible role of S100A4 in NAFLD, which has been increasingly recognized as an inflammatory disease with different degrees of liver fibrosis.15 However, it remains unclear whether S100A4 is associated with the pathology of NAFLD. Methionine-choline-deficient (MCD) diet impaired the secretory process of very low-density lipoprotein from the liver, which could induce hepatic lipid accumulation, aminotransferase elevation, and hepatic histological changes including steatosis, hepatic inflammation, and fibrosis.1617 These histological changes were similar to those of human NAFLD pathology.18 Therefore, MCD diet has been used as an internationally recognized animal model of NAFLD.1920 In our study, we intended to observe the influences of S100A4 knockout (KO) on NAFLD by feeding S100A4 KO mice and their wild-type (WT) counterparts with either MCD diet or methionine-choline-sufficient (MCS) control diet, which were identical to MCD but sufficient in choline chloride (2 g/kg) and DL-methionine (3 g/kg).
A total of 100 male SPF C57BL/6 mice (age: 6–8 weeks; weighing: 18–20 g) were purchased from Shanghai Experimental Animal Center of Chinese Academy of Sciences (Shanghai, China). The current study was consistent with the Laboratory Animal Use Convention published by the National Institutes of Health,21 and all animal experimental procedures were conducted and supervised by the Medical Laboratory Animal Ethics Committee of Taihe Hospital.
The study on S100A4 KO mice was conducted with a germline inactivation of S100A4 gene, as described from the previous study.22 S100A4 KO mice (n=20) and their WT counterparts (n=20) were randomly divided into model and control groups. The mice in model group were fed with MCD diet, namely KO/MCD and WT/MCD groups with 10 mice in each group, and control group mice were treated with MCS diet, namely KO/MCS and WT/MCS groups with another 10 mice in each group. The composition of MCS was identical to MCD but sufficient in choline chloride (2 g/kg) and DL-methionine (3 g/kg). Both MCS and MCD were obtained from MP Biomedicals (Solon, OH, USA).
Mice in each group were executed after 8 weeks of feeding, and peripheral blood was obtained after removal of eye-balls. Then, serum was collected after centrifugation and stored at −20℃. The blood biochemical parameters including alanine aminotransferase (ALT), aspartate aminotransferase (AST), triglyceride (TG), and total cholesterol (TC) levels in each group were measured by an automatic biochemical analyzer 7180 (Hitachi Ltd, Tokyo, Japan). Mice were fixed on the operating table, and their skin and peritoneum were cut open using surgical scissors, exposing and removing liver tissues. A part of acquired liver tissues was stabilized in 4% paraformaldehyde for 24 h to make regular paraffin embedded slices, while the other part was fixed in 4% paraformaldehyde for 2–4 h and soaked in 30% sucrose solution overnight at 4℃, which was stored in a refrigerator at −80℃ for subsequent tests after optimal cutting temperature embedded.
Hematoxylin and eosin (HE) staining: Slices of liver tissues were dewaxed in xylene twice for 5 min, dehydrated with gradient alcohol, and washed with distilled water for 5 min. Then, slices were stained with hematoxylin stain for 5 min and differentiated with 1% hydrochloric acid for 30 s, followed by 1% eosin-alcohol dyeing for 5 min, which could be observed under a microscope after regular gradient alcohol dehydration and mounting.
Oil Red O (ORO) staining: Tissue sections were placed on slide sat room temperature for 30 min, fixed in 10% ice paraformaldehyde for 10 min, and then washed three times by distilled water. After drying for several minutes, oil red and deionized water were diluted in a 3:2 ratio and placed at room temperature for 10 min. Following that, slices experienced ORO staining for 8 min, 85% propylene glycol solution differentiation for 2 min, washed twice, hematoxylin counterstained for 30 s, flushed with water for 3 min, and then mounted for microscope observation.
Masson staining: Paraffin section of mice was observed after a series of procedures including routine dewaxing rehydration, ponceaufuchs in acid solution staining for 5–10 min followed by washing, 1% phosphomolybdic acid solution differentiation for 5 min, aniline blue solution counterstain for 5 min, treatment of 1% glacial acetic acid for 1 min, alcohol gradient dehydration, transparent through dimethylbenzene xylene, and mounting.
NAFLD was diagnosed according to NAFLD activity scores (NAS) including steatosis (0–3), lobular inflammation (0–3), and hepatocyte ballooning (0–2),23 while liver fibrosis was calculated as grade 0 (none), grade 1 (zone perisinusoidal fibrosis), grade 2 (as above with portal fibrosis), grade 3 (as above with bridging fibrosis), and grade 4 (cirrhosis).24
Total RNA was extracted by using total RNA extraction kit (Beijing Tian Enze Gene Technology Co., Ltd., Beijing, China), and cDNA was synthesized under the support of reverse transcription kit (Hangzhou Bori Technology Co., Ltd., Hangzhou, China). RT-PCR mixture was obtained from Bio-Rad (Hercules, CA, USA), and the reaction was carried out on ABI 7500 Quantitative PCR instrument (Appplied Biosystems, Foster City, CA, USA) under the following conditions: pre-denaturation at 94℃ for 5 min, followed by 40 cycles of denaturation at 94℃ for 30 s, annealing at 58℃ for 30 s, and extension at 72℃ for 20 s. GAPDH was selected as a reference gene, and formula 2−ΔΔCT was used to compare and analyze the differences in gene expressions. The experiment was repeated three times.
Liver tissue was dissolved in a pre-cooled (2 mL) phosphate-buffered saline (PBS) solution (pH 7.4). Total protein concentration of supernatant was quantified by Bradford method after ultrasonic disintegration. Protein was separated by polyacrylamide gel electrophoresis, and then was transferred to polyvinylidene difluoride (PVDF) membrane semi-dry membrane apparatus (Bio-Rad, USA). The transferred PVDF membrane was blocked by skimmed milk powder at room temperature, and washed with PBS with Tween-20 (PBST) buffer. Afterwards, 1 µg/mL anti-S100A4 antibody (ab41532) and 1 µg/mL anti-β-actin antibody (ab8227), purchased from Abcam (Cambridge, MA, USA), were added for hybridization at room temperature for 1 h. After being washed with PBST buffer for five times×3 min, membrane was incubated with the second antibody, and washed with PBST again. At last, target protein was detected with horseradish peroxidase (HRP) substrate (Bio-Rad). The relative content of target protein was expressed by the ratio of gray-value to the corresponding internal reference (S100A4/β-actin). We conducted every experiment three times for mean value.
Paraffin sections of liver tissue were routinely dewaxed and rehydrated, and 5 µL TdT and 45 µL fluoresce in-labeled dUTP were added for incubation at 37℃ for 60 min, and rinsed with PBS for 3 min×three times. Then, sections with an additional 50 µL converter-PODs were incubated at 37℃ for 30 min, with PBS washing for 3 min in triplicate. After that, moderate DAB (3, 3′-diaminobenzidine) substrate was added for coloration, and hematoxylin was used for counterstaining. Subsequently, sections were dehydrated and mounted, and positive apoptotic cells appeared reddish brown under a light microscope. Apoptotic- positive cells in a total of 1000 cells were counted by microscopic examination in 5–10 random fields, according to apoptotic index (AI).
All statistical data were analyzed using SPSS 22.0 (IBM Corp., Armonk, NY, USA). Measured data in this study were expressed as mean±standard deviation. Differences between the two groups were compared using an independent sample t-test. Among groups, differences were analyzed by one-way ANOVA, and the least significant difference test was applied for inter-group analyses. p<0.05 was considered significant.
After feeding with MCD or MCS for 8 weeks, no significant differences were found in liver function (ALT and AST) and lipid-related parameters (TG and TC) between mice of WT/MCS group and KO/MCS group (all p>0.05). However, serum levels of the above parameters were obviously higher in MCD diet mice compared to MCS-diet mice; specifically, these factors were relatively lower in mice from KO/MCD group than those of WT/MCD group (all p<0.05) (Fig. 1).
As shown in Fig. 2, livers in MCS-diet fed mice exhibited dark red color, smooth and shiny surface, and sharp edges. On the other hand, livers of mice in WT/MCD group had khaki-yellow color, while the edges became blunt with increased volume and tense capsule, and even coagulation and yellow-white focal degeneration occurred. Livers in mice from KO/MCD group showed slightly yellow color and larger size, along with slightly tough and smooth surface.
According to the detection of qRT-PCR and Western blot, S100 A4 mRNA and protein expressions in liver tissues of mice from WT/MCD group were found to be highly upregulated compared to those of mice in WT/MCS group (all p<0.05), as shown in Fig. 3, but were not detected in mice from both KO/MCS and KO/MCD groups.
In order to determine the effect of S100A4 KO on liver tissue injury in MCD diet-induced NAFLD mice, we performed HE staining, ORO staining, and Masson staining to assess hepatic inflammation and fibrosis by using NAS, to visualize lipid droplets, and to observe the distribution of collagen fibers in mice liver tissue of each group, respectively. As illustrated in Fig. 4A, MCS-fed-induced mice had regular structure of hepatic lobule, clearly visible hepatic sinus, as well as normal structure and morphology of hepatic cells, without lipid droplets deposition and collagen fibers. However, a large number of fat vacuoles accumulated in liver tissues of mice in WT/MCD group and hepatocytes showed balloon-like changes accompanied by increased inflammatory cell and collagen fibers. Other than that, reduced liver cell damage and inflammatory cell infiltration, coupled with a small amount of collagen fibers, were observed in liver tissues of mice in KO/MCD group. Moreover, steatosis, inflammatory infiltration, ballooning, total NAS, and liver fibrosis in KO/MCD group were significantly lower compared to those in WT/MCD group (all p<0.05) (Fig. 4B–F).
qRT-PCR was performed to detect the expression of pro-inflammatory (including TNF-α, IL-1β, and IL-6) and profibrogenic cytokines (including TGF-β1, COL1A1, and α-SMA) in liver tissues of mice, as demonstrated in Fig. 5. As a result, mRNA levels of TNF-α, IL-1β, IL-6, TGF-β1, COL1A1, and α-SMA were significantly higher in mice fed with MCD than those fed with MCS (all p<0.05). As for mice in MCD groups, expressions of proinflammatory-/profibrogenic cytokines in KO mice were significantly lower than those in WT group (all p<0.05).
As evaluated by TUNEL staining in Fig. 6, increased number of TUNEL-positive hepatocytes were discovered in mice of WT+MCD group compared to mice in MCS group, which exhibited less and scattered positive hepatocytes, while mice in KO+MCD group showed less positive hepatocytes than those in WT+MCD group. Furthermore, statistical analysis demonstrated that AI of mice in WT/MCD group was markedly elevated compared to mice in KO/MCS and WT/MCS groups, but was obviously decreased in mice from KO/MCD group when compared to those in WT/MCD group (all p<0.05).
“First hit” refers to several processes, including the digestion and absorption of exogenous lipids, lipoprotein metabolism, as well as conversion and decomposition of cholesterol in liver, to serve as a part of NAFLD pathogenesis.25 Concerning MCD diet-fed mice, liver function indexes (ALT and AST) and blood lipid parameters (TG and TC) were significantly lower in KO mice than in WT mice, suggesting that S100A4 deletion enhanced liver function and blood lipid levels in NAFLD mice. Similarly, patients with type 2 diabetes also had higher serum S100A4 concentrations, which were related to the metabolic pathways.1 This demonstrates an important role of S100A4 in lipid metabolism, possibly since S100A4 could activate the expression of receptor of advanced glycation end products in hepatocytes and hepatic stellate cells (HSCs), and thereby exerting functions in development of NAFLD.2627
In addition, “second hit” has been widely acknowledged as the pathophysiological model of NAFLD, which is defined as increased oxidative stress and initiation of lipid peroxidation, resulting in the formation of inflammatory mediators and activation of HSCs to produce irreversible lesions in hepatocytes.2829 A recent study demonstrated a significant role of S100A4 in the inflammatory response of diseases.30 In our study, S100A4 KO mice fed with MCD showed noticeably decreased liver steatosis, inflammation, and ballooning scores, with reduced total NAS and downregulated pro-inflammatory cytokines (including TNF-α, IL-1β, and IL-6) expressions in liver tissues, compared to WT mice fed with MCD. As documented, TNF-α is a firstly appearing cytokine in the process of liver injury, which could facilitate the release of IL-1β and IL-6, acting as a crucial factor in the progress from NAFLD to NASH,31 indicating S100A4 deletion weakened NAFLD inflammation which could be possibly related to the inflammatory response mediated by TLR4 signaling.32 In addition, S100A4 was credited as a fibroblast-specific marker in liver fibrosis. For example, S100A4 was found to be secreted by a subpopulation of macrophages in fibrotic liver,33 and its increased levels in liver tissue and serum of hepatitis patients were positively correlated with liver fibrosis.34 In our study, less amount of collagen fiber was observed in MCD diet-fed KO mice compared to WT mice, and expressions of pro-fibrogenic cytokines (including TGF-β1, COL1A1, and α-SMA) were also significantly reduced, which might have resulted from the overexpression of α-SMA stimulated by S100A4 through c-Myb in HSCs to promote liver fibrosis,33 or a common mediator of S100A4 in epithelial-mesenchymal transition,35 thus contributing to the cirrhosis progression in NAFLD.36
In recent years, “third hit” of NAFLD was pointed out, namely hepatocyte apoptosis, which could accelerate the transformation of NAFLD into liver cirrhosis.4 In NAFLD patients, hepatocyte proliferation was blocked, and apoptotic cells was replaced by proliferation and differentiation of hepatic progenitor cells, leading to hepatic fibrosis and inflammatory cell infiltration, and so on.37 In our research, S100A4 KO reduced hepatocyte apoptosis in MCD diet-induced mice, showing that S100A4 deficiency might play a protective role in NAFLD via inhibiting hepatocyte apoptosis. A possible reason could be correlated with elevated levels of WT p53,38 which enhances TGF-β-induced p66Shc signaling, ROS accumulation, and hepatocyte apoptosis.39 On the contrary, S100A4 KO could also lead to the stabilization of p53 protein in two p53 WT cell lines (A549 and HeLa), which implies that S100A4 could accelerate p53 degradation.40 Therefore, whether S100A4 can affect hepatocyte apoptosis in NAFLD mice via regulation of p53 should be further explored, and we plan to deeply investigate this matter in our subsequent and future studies. In summary, S100A4 was upregulated in NAFLD mice, and S100A4 KO significantly improved liver function and blood lipid levels, contributing to reduced hepatic fibrosis and inflammation as well as inhibited hepatocyte apoptosis in MCD diet-induced NAFLD mice, which provided new clues for the treatment of NAFLD.
Figures and Tables
Fig. 1
S100A4 KO improves liver function and blood lipid-related parameters in MCD diet-induced non-alcoholic fatty liver disease mice. Influences of S100A4 KO on serum ALT (A), AST (B), TC (C), and TG (D) levels in mice. *p<0.05 compared to MCS group, †p<0.05 compared to WT/MCD group. S100A4, S100 calcium binding protein A4; MCD, methionine-choline-deficient; MCS, methionine-choline-sufficient; KO, knockout; WT, wild-type; ALT, aminotransferase; AST, aminotransferase; TG, triglyceride; TC, total cholesterol.
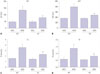
Fig. 2
Effect of S100A4 KO on liver morphology in MCD diet-induced non-alcoholic fatty liver disease mice. S100A4, S100 calcium binding protein A4; KO, knockout; WT, wild-type; MCD, methionine-choline-deficient; MCS, methionine-choline-sufficient.
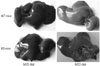
Fig. 3
Relative S100A4 mRNA and protein expressions in liver tissues of mice detected by qRT-PCR (A) and Western blot (B and C). *p<0.05 compared to WT/MCS group. S100A4, S100 calcium binding protein A4; MCD, methionine-choline-deficient; MCS, methionine-choline-sufficient; KO, knockout; WT, wild-type.
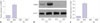
Fig. 4
S100A4 KO reduces liver tissue damage and inflammatory cell infiltration in MCD diet-induced NAFLD mice. (A) HE staining, ORO staining, and Masson staining were used to observe pathological changes of liver tissues in each group (×400); (B–E) Comparison of steatosis (B), lobular inflammation (C), hepatocyte ballooning (D), total NAS (E), and fibrosis (F) in liver tissues of mice in each group. *p<0.05 compared to MCS group, †p<0.05 compared to WT/MCD group. S100A4, S100 calcium binding protein A4; MCD, methionine-choline-deficient; MCS, methionine-choline-sufficient; KO, knockout; WT, wild-type; HE, hematoxylin and eosin; ORO, Oil Red O; NAFLD, non-alcoholic fatty liver; NAS, NAFLD activity scores.
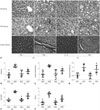
Fig. 5
S100A4 KO on expressions of proinflammatory cytokines (TNF-α, IL-1β, and IL-6) (A) and profibrogenic cytokines (TGF-β1, COL1A1 and α-SMA) (B) in liver tissues of MCD diet-induced non-alcoholic fatty liver disease mice detected by qRT-PCR. *p<0.05 compared to MCS group, †p<0.05 compared to WT/MCD group. S100A4, S100 calcium binding protein A4; MCD, methionine-choline-deficient; MCS, methionine-choline-sufficient; KO, knockout; WT, wild-type.
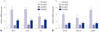
Fig. 6
S100A4 KO on hepatocyte apoptosis in MCD diet-induced non-alcoholic fatty liver disease mice evaluated by TUNEL staining (×400). Black arrows indicate hepatocyte apoptosis. *p<0.05 compared to MCS group, †p<0.05 compared to WT/MCD group. S100A4, S100 calcium binding protein A4; MCD, methionine-choline-deficient; MCS, methionine-choline-sufficient; KO, knockout; WT, wild-type.
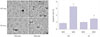
References
1. Arner P, Petrus P, Esteve D, Boulomié A, Näslund E, Thorell A, et al. Screening of potential adipokines identifies S100A4 as a marker of pernicious adipose tissue and insulin resistance. Int J Obes (Lond). 2018; 01. 30. [Epub]. DOI: 10.1038/s41366-018-0018-0.


2. Afonso MB, Rodrigues PM, Simão AL, Castro RE. Circulating microRNAs as potential biomarkers in non-alcoholic fatty liver disease and hepatocellular carcinoma. J Clin Med. 2016; 5:pii: E30.


3. Nair S. Nonalcoholic Fatty liver disease from the perspective of an internist. Ochsner J. 2002; 4:92–97.
4. Patel A, Harrison SA. Hepatitis C virus infection and nonalcoholic steatohepatitis. Gastroenterol Hepatol (N Y). 2012; 8:305–312.
5. Dongiovanni P, Valenti L. Genetics of nonalcoholic fatty liver disease. Metabolism. 2016; 65:1026–1037.


6. Saleh A, Kamel L, Ghali A, Ismail A, El Khayat H. Serum levels of astroglial S100-beta and neuron-specific enolase in hepatic encephalopathy patients. East Mediterr Health J. 2007; 13:1114–1123.


7. Dempsey BR, Rintala-Dempsey AC, Shaw GS. S100 proteins. In : Choi S, editor. Encyclopedia of signaling molecules. New York (NY): Springer;2012. p. 1711–1717.
8. Mukai K, Miyagi T, Nishio K, Yokoyama Y, Yoshioka T, Saito Y, et al. S100A8 production in CXCR2-expressing CD11b+Gr-1high cells aggravates hepatitis in mice fed a high-fat and high-cholesterol diet. J Immunol. 2016; 196:395–406.


9. Liu X, Wang Y, Ming Y, Song Y, Zhang J, Chen X, et al. S100A9: a potential biomarker for the progression of non-alcoholic fatty liver disease and the diagnosis of non-alcoholic steatohepatitis. PLoS One. 2015; 10:e0127352.


10. Malashkevich VN, Dulyaninova NG, Ramagopal UA, Liriano MA, Varney KM, Knight D, et al. Phenothiazines inhibit S100A4 function by inducing protein oligomerization. Proc Natl Acad Sci U S A. 2010; 107:8605–8610.


11. Hou S, Tian T, Qi D, Sun K, Yuan Q, Wang Z, et al. S100A4 promotes lung tumor development through β-catenin pathway-mediated autophagy inhibition. Cell Death Dis. 2018; 9:277.


12. Oslejsková L, Grigorian M, Gay S, Neidhart M, Senolt L. The metastasis associated protein S100A4: a potential novel link to inflammation and consequent aggressive behaviour of rheumatoid arthritis synovial fibroblasts. Ann Rheum Dis. 2008; 67:1499–1504.


13. Cerezo LA, Kuncová K, Mann H, Tomcík M, Zámecník J, Lukanidin E, et al. The metastasis promoting protein S100A4 is increased in idiopathic inflammatory myopathies. Rheumatology (Oxford). 2011; 50:1766–1772.


14. Louka ML, Ramzy MM. Involvement of fibroblast-specific protein 1 (S100A4) and matrix metalloproteinase-13 (MMP-13) in CCl4-induced reversible liver fibrosis. Gene. 2016; 579:29–33.


15. Wree A, Broderick L, Canbay A, Hoffman HM, Feldstein AE. From NAFLD to NASH to cirrhosis-new insights into disease mechanisms. Nat Rev Gastroenterol Hepatol. 2013; 10:627–636.


16. Okubo H, Kushiyama A, Sakoda H, Nakatsu Y, Iizuka M, Taki N, et al. Involvement of resistin-like molecule β in the development of methionine-choline deficient diet-induced non-alcoholic steatohepatitis in mice. Sci Rep. 2016; 6:20157.


17. Rizki G, Arnaboldi L, Gabrielli B, Yan J, Lee GS, Ng RK, et al. Mice fed a lipogenic methionine-choline-deficient diet develop hypermetabolism coincident with hepatic suppression of SCD-1. J Lipid Res. 2006; 47:2280–2290.


18. Rinella ME, Elias MS, Smolak RR, Fu T, Borensztajn J, Green RM. Mechanisms of hepatic steatosis in mice fed a lipogenic methionine choline-deficient diet. J Lipid Res. 2008; 49:1068–1076.


19. Wang Y, Li J, Zhuge L, Su D, Yang M, Tao S, et al. Comparison between the efficacies of curcumin and puerarin in C57BL/6 mice with steatohepatitis induced by a methionine- and choline-deficient diet. Exp Ther Med. 2014; 7:663–668.


20. Kim SB, Kang OH, Lee YS, Han SH, Ahn YS, Cha SW, et al. Hepatoprotective effect and synergism of bisdemethoycurcumin against MCD diet-induced nonalcoholic fatty liver disease in mice. PLoS One. 2016; 11:e0147745.


21. National Research Council. Guide for the care and use of laboratory animals. 8th ed. Washington (DC): National Academies Press;2011.
22. EL Naaman C, Grum-Schwensen B, Mansouri A, Grigorian M, Santoni-Rugiu E, Hansen T, et al. Cancer predisposition in mice deficient for the metastasis-associated Mts1(S100A4) gene. Oncogene. 2004; 23:3670–3680.


23. Alam S, Alam M, Alam SMNE, Chowdhury ZR, Kabir J. Prevalence and predictor of nonalcoholic steatohepatitis (NASH) in nonalcoholic fatty liver disease (NAFLD). J Bangladesh Coll Phys Surg. 2014; 32:71–77.


24. García-Galiano D, Sánchez-Garrido MA, Espejo I, Montero JL, Costán G, Marchal T, et al. IL-6 and IGF-1 are independent prognostic factors of liver steatosis and non-alcoholic steatohepatitis in morbidly obese patients. Obes Surg. 2007; 17:493–503.


25. Ying LI, Hong ZF. Hypothesis of “two hits” in NAFLD. Med Recapitulate. 2013; 19:594–596.
26. Abu El-Asrar AM, Nawaz MI, De Hertogh G, Alam K, Siddiquei MM, Van den Eynde K, et al. S100A4 is upregulated in proliferative diabetic retinopathy and correlates with markers of angiogenesis and fibrogenesis. Mol Vis. 2014; 20:1209–1224.
27. Takeuchi M, Takino JI, Sakasai-Sakai A, Takata T, Tsutsumi M. Toxic AGE (TAGE) theory for the pathophysiology of the onset/progression of NAFLD and ALD. Nutrients. 2017; 9:pii: E634.


28. Tilg H, Moschen AR. Evolution of inflammation in nonalcoholic fatty liver disease: the multiple parallel hits hypothesis. Hepatology. 2010; 52:1836–1846.


29. Berlanga A, Guiu-Jurado E, Porras JA, Auguet T. Molecular pathways in non-alcoholic fatty liver disease. Clin Exp Gastroenterol. 2014; 7:221–239.
30. Klingelhöfer J, Senolt L, Baslund B, Nielsen GH, Skibshøj I, Pavelka K, et al. Up-regulation of metastasis-promoting S100A4 (Mts-1) in rheumatoid arthritis: putative involvement in the pathogenesis of rheumatoid arthritis. Arthritis Rheum. 2007; 56:779–789.


31. Tilg H, Moschen AR, Szabo G. Interleukin-1 and inflammasomes in alcoholic liver disease/acute alcoholic hepatitis and nonalcoholic fatty liver disease/nonalcoholic steatohepatitis. Hepatology. 2016; 64:955–965.


32. Sharifnia T, Antoun J, Verriere TG, Suarez G, Wattacheril J, Wilson KT, et al. Hepatic TLR4 signaling in obese NAFLD. Am J Physiol Gastrointest Liver Physiol. 2015; 309:G270–G278.


33. Chen L, Li J, Zhang J, Dai C, Liu X, Wang J, et al. S100A4 promotes liver fibrosis via activation of hepatic stellate cells. J Hepatol. 2015; 62:156–164.


34. Yan LB, Zhang QB, Zhu X, He M, Tang H. Serum S100 calcium binding protein A4 improves the diagnostic accuracy of transient elastography for assessing liver fibrosis in hepatitis B. Clin Res Hepatol Gastroenterol. 2018; 42:64–71.


35. Schneider M, Hansen JL, Sheikh SP. S100A4: a common mediator of epithelial-mesenchymal transition, fibrosis and regeneration in diseases? J Mol Med (Berl). 2008; 86:507–522.


36. Syn WK, Jung Y, Omenetti A, Abdelmalek M, Guy CD, Yang L, et al. Hedgehog-mediated epithelial-to-mesenchymal transition and fibrogenic repair in nonalcoholic fatty liver disease. Gastroenterology. 2009; 137:1478–1488.


37. Maher JJ. Pathogenesis of NAFLD and NASH. In : Chalasani N, Szabo G, editors. Alcoholic and non-alcoholic fatty liver disease. Cham: Springer;2016. p. 71–101.
38. Mazzucchelli L. Protein S100A4: too long overlooked by pathologists? Am J Pathol. 2002; 160:7–13.

