Abstract
Purpose
To investigate the effects of hyperbaric oxygen (HBO) pretreatment on cognitive decline and neuronal damage in an Alzheimer’s disease (AD) rat model.
Materials and Methods
Rats were divided into three groups: normal saline (NS), AD, and HBO+AD. In the AD group, amyloid β peptide (Aβ)1-40 was injected into the hippocampal CA1 region of the brain. NS rats received NS injection. In the HBO+AD group, rats received 5 days of daily HBO therapy following Aβ1-40 injection. Learning and memory capabilities were examined using the Morris water maze task. Neuronal damage and astrocyte activation were evaluated by hematoxylin-eosin staining and immunohistochemistry, respectively. Dendritic spine density was determined by Golgi-Cox staining. Tumor necrosis factor-α, interleukin-1β, and interleukin-10 production was assessed by enzyme-linked immunosorbent assay. Neuron apoptosis was evaluated by terminal deoxynucleotidyl transferase dUTP nick end labeling. Protein expression was examined by western blotting.
Results
Learning and memory dysfunction was ameliorated in the HBO+AD group, as shown by significantly lower swimming distances and escape latency, compared to the AD group. Lower rates of neuronal damage, astrocyte activation, dendritic spine loss, and hippocampal neuron apoptosis were seen in the HBO+AD than in the AD group. A lower rate of hippocampal p38 mitogen-activated protein kinase (MAPK) phosphorylation was observed in the HBO+AD than in the AD group.
Alzheimer’s disease (AD) is a neurodegenerative disorder characterized by progressive learning and memory deficits. Although the pathogenesis of AD is not well understood, it is widely accepted that AD is associated with plaques and tangles within the brain and that the plaques distributed throughout the cortex and hippocampus are mostly insoluble deposits of amyloid β peptide (Aβ).1 The accumulation of Aβ in patients with AD can activate astrocytes and induce the generation of proinflammatory cytokines released by activated astrocytes, leading to brain damage and cognitive decline.2 Additionally, higher numbers of astrocytes have been found to surround Aβ deposits in patients with AD, compared to those without.3 Activated astrocytes also contribute to the accumulation of Aβ by secreting inflammatory proteins.4
The molecular mechanisms involved in neuronal damage, astrocyte activation, and cognitive decline associated with Aβ accumulation in patients with AD have not yet been fully elucidated. Recent studies have highlighted an important role for p38 mitogen-activated protein kinase (MAPK) signal pathway as a potential target for treatment in AD.5 p38 MAPK is activated (phosphorylated) in the early stage of neurofibrillary degeneration in the hippocampus of patients with AD.6 The phosphorylation of p38 MAPK regulates neuroinflammation, cytoskeletal remodeling, and tau hyperphosphorylation, contributing to aggravation of AD.7
Hyperbaric oxygen (HBO) treatment exhibits neuroprotective effects. HBO therapy reportedly provided neuroprotection in a rat model of spinal cord injury.8 Moreover, HBO reduced the severity of brain injury and ameliorated behavioral function in a rat model of neonatal hypoxia-ischemia.9 HBO treatment also reportedly alleviates chronic constrictive injury-induced neuropathic pain and inhibits endoneuronal tumor necrosis factor-α (TNF-α) production,10 suggesting that HBO therapy has anti-inflammatory effects. The antioxidant effects yielded by HBO improve the learning and memory impairments in AD rats. HBO exerts potential protective effects through inhibiting mitochondria-dependent apoptosis induced by Aβ-mediated oxidative stress.11 Aβ causes a significant decrease in the memory and learning capabilities of rats, accompanied by neuronal damage in the hippocampus, as well as the overproduction of methane dicarboxylic aldehyde and NO. HBO efficiently improves memory and learning ability possibly via reducing hippocampal neuron injury and enhancing the activities of oxidative scavenging enzymes.12 Thus, the use of HBO may be a promising therapeutic option for the management of AD. Nevertheless, the precise effects of HBO on the development of Aβ accumulation-induced cognitive deficits and the mechanisms of these effects remain unclear.
In the present study, we investigated the protective effects of HBO pretreatment on learning and memory dysfunction, neuronal damage, and astrocyte activation in a rat model of AD. The underlying mechanisms were also elucidated. Our findings provide valuable insights into understanding the pathogenesis of AD, as well as promising approaches to the management of cognitive decline in patients with AD.
Twenty-four 4-month-old male Sprague-Dawley rats weighing 260 to 290 g were obtained from the Center of Laboratory Animal Science of GuangDong (SCXK 2013-0002). The Animal Ethics Committee of Guangzhou Women and Children’s Medical Center (Guangzhou, China) approved all of the experimental protocols. All experiments were performed in accordance with the Guidelines for the Care and Use of Laboratory Animals of the National Institutes of Health and the Animal Welfare Act.
The rats were randomly divided into three groups of eight rats each using a random number table. The three groups were the normal saline control (NS group), AD group, and AD+HBO group. A rat model of AD was established as follows. The rats were anesthetized and placed on a stereotaxic apparatus (I-C type; RWD Life Science Co. Ltd., Shenzheng, China). Aβ1-40 was injected into the hippocampal CA1 region: 3.2 mm posterior to the bregma, 2.0 mm lateral to the midline, and 2.9 mm ventral to the surface of the skull. A hole was drilled in the parietal skull plate to expose the dura mater. A micro sample syringe (SR034 Shanghai Laser Co. Ltd., Shanghai, China) preloaded with 2 µL of Aβ1-40 (5 µg/µL, Sigma-Aldrich, St. Louis, MO, USA) was slowly inserted into the bilateral hippocampal CA1 region through the opening in the skull. Prior to use, Aβ1-40 was dissolved in distilled water to a working concentration of 5 µg/µL and incubated at 37℃ for 1 week to form Aβ oligomers. Rats in the AD and AD+HBO groups received injections of Aβ1-40. Rats in the NS group underwent identical procedures but were injected with 2 µL of NS instead of Aβ1-40.
HBO therapy was conducted as previously described.13 The chamber was filled with pure oxygen for 10 min. Rats in the AD+HBO group were placed into the chamber for HBO therapy. The chamber was compressed at a consistent compression rate of 10 kPa/min to a target pressure of 2.0 atmospheres absolute pressure, and this target pressure was maintained for 60 min. The chamber was then decompressed at a consistent compression rate of 10 kPa/min to normal atmospheric pressure. The entire procedure lasted for 100 min. Rats in the AD+HBO group received HBO therapy once daily following surgery. The HBO treatment began on the first postoperative day and ended on the fifth postoperative day. Rats in the NS and AD groups were simply placed into the chamber for approximately 100 min without compression or decompression treatment.
The Morris water maze task was used to assess spatial learning and memory ability in the rats. The Morris water maze apparatus was provided by the Institute of Materia Medica, Chinese Academy of Medical Sciences, Beijing, China. The pool was divided into four quadrants (I, II, III, and IV). A platform (15-cm diameter, 33-cm depth) was submerged 1 cm below the water surface in the center of quadrant IV. Training trials were conducted 5 days prior to surgery. Each rat underwent four training trials per day for 5 days. The rats were initially placed into the different quadrants and given 90 s to locate the hidden platform. If the rat failed to find the platform in the allotted time, it was guided to the platform, allowed to stay for 30 min, and given a score of 90 s. The tests were conducted on postoperative days 7, 14, and 21. The swimming distance (cm), escape latency (s), and average velocity (cm/s) were recorded using the ANYmaze video tracking system (Anymaze, XinRuan Information Technology Co. Ltd., Shanghai, China).
After all rats had completed the behavior tests (postoperative day 21), they were anesthetized. Four rats were then perfused with 0.9% NS and subsequently fixed with 4% paraformaldehyde. The hippocampal tissues were removed, and sectioned to 6-µm thickness for histological studies, immunohistochemical analysis, and terminal deoxynucleotidyl transferase dUTP nick end labeling (TUNEL) staining. Four other rats were euthanized, and their hippocampal tissues were removed for western blotting and enzyme-linked immunosorbent assay (ELISA).
Sections were stained with hematoxylin solution for 1 to 2 min, washed under running tap water, and differentiated in acid ethanol. Samples were counterstained in 0.5% eosin solution.
Astrocyte activation was examined by immunohistochemistry. 13 Sections were probed with rabbit polyclonal anti-glial fibrillary acidic protein (anti-GFAP) primary antibody (1:1000 dilution; Dako, Copenhagen, Denmark). On the second day, the samples were incubated with goat anti-rabbit biotinylated secondary antibody (1:500 dilution; Beijing Zhongshan Golden Bridge Biological Technology Co. Ltd., China), followed by incubation in SABC complex (Vector Laboratories, CA, USA). The antibody binding was visualized with 3, 3'-diaminobenzidine tetrahydrochloride (DAB). Five fields were randomly selected from each immunostained section. Data were analyzed by Qwin V3 software. The degree of astrocyte activation was determined by the following equation: astrocyte activation (%)=(number of activated astrocytes/total number of astrocytes)×100%.
The levels of TNF-α, interleukin (IL)-1β and IL-10 in the hippocampus were measured by ELISA.10 The unilateral hippocampus was dissected, ground with a grinder, and loaded onto an ultrasonic tissue homogenizer. The supernatant was collected after centrifugation. TNF-α, IL-1β, and IL-10 production was evaluated using ELISA kits according to the manufacturer’s instructions (Wuhan Boster Biological Technology Co. Ltd., Wuhan, China). The optical density values at 490 nm were recorded using a microplate reader (NK3; Ladsystems, Helsinki, Finland). The average levels of TNF-α, IL-1β, and IL-10 were calculated based on the standard curve.
Frozen brain sections were stained with Golgi-Cox using the FD Rapid Golgi stain kit (FDNeuroTechnologies, Inc., Columbia, MD, USA). Ten CA1 neurons that were well individualized were randomly selected in the CA1 region of hippocampus from each rat. The dendritic spine analysis was carried out under an LSM 510 metaconfocal microscope (Carl-Zeiss, Gottingen, Germany) equipped with an oil-immersion objective lens and Imaris software (BitPlane AG, Zurich, Switzerland) for the tridimensional reconstruction. The spine density was measured by selecting and photographing 10 neurons from each animal and matching regions of distal tips of the dendrites. The spine numbers were counted in 40 µm segments by using a 100× objective lens, and the results were expressed as the number of spines/10 µm.
Sections were incubated with 3% hydrogen peroxide for 10 min. After being washed with tris-buffered saline (TBS), the samples were incubated with labeling buffer (20 µL/section) for 2 h at 37℃. After being washed, the samples were treated with 1% SABC diluted in TBS for 1 h at 37℃. They were then rewashed with TBS and visualized with DAB. Samples were counterstained with hematoxylin solution. Three sections were used for each group, and five fields were randomly selected from each section under 400 magnification. Images were analyzed using Image-Pro Plus 6.0 software (Olympus Co., Tokyo, Japan). The apoptotic index was calculated using the following equation: apoptotic index=(number of apoptotic cells/total number of cells)× 100%.
To detect expression of p38 MAPK and cleaved caspase 3 in hippocampal tissues, total protein was first extracted using lysis buffer. Protein extracts were separated by sodium dodecyl sulfate-polyacrylamide gel electrophoresis and then transferred to polyvinylidene difluoride membranes. The membranes were then blocked and incubated with rabbit monoclonal antiphosphorylated p38 MAPK primary antibody (1:1000 dilution; Santa Cruz, CA, USA) or anti-caspase 3 antibody (1:500 dilution; Santa Cruz, CA, USA), followed by incubation with goat anti-rabbit IgG secondary antibody (1:2000 dilution; Santa Cruz, CA, USA). β-actin was used as a loading control. The expression bands of target proteins were analyzed by Scion Image software (version 4.0.3; Scion Co., Santa Cruz, CA, USA). The densitometric values were used to conduct the statistical analysis. The relative protein expression was calculated over β-actin.
Data were analyzed by SPSS 17.0 software, and are presented as mean±standard deviation. Statistical significance among the groups was determined using one-way analysis of variance. Comparisons between two groups were conducted using the least significant difference t test. All p values <0.05 were considered to be significantly different.
Before the experiments, no significant differences were observed in the learning and memory abilities of the rats among the three groups (p>0.05) (Fig. 1A, B and C). Moreover, there were no statistical differences in the swimming distance, escape latency, or average velocity among the three experimental groups on postoperative days 7 and 14 (p>0.05). However, the swimming distance and escape latency were significantly elevated in the AD group on day 21, indicating learning and memory impairment in these rats (p<0.05). The learning and memory dysfunction was partially prevented by HBO pretreatment. The swimming distance and escape latency were significantly lower in the AD+HBO than in the AD group on day 21 (p<0.05). Representative results of the track path in rats from different groups are presented in Fig. 1D. The average velocity did not change with the different treatments (p>0.05).
Next, we investigated the alterations in the hippocampal CA1 region of rats from the three experimental groups. Histological examination revealed that rats in the NS control group had an intact hippocampal structure with regularly arranged cells (Fig. 2A). The lightly stained nucleus was relatively large and round with clear nucleolus formation, and the neurons were rich in cytoplasm. In contrast, the neurons of rats in the AD group were loosely distributed and exhibited swelling and vacuolation. The nucleus was darkly stained and condensed, and some of the neurons showed irregular morphology with small cell soma. The number of damaged neurons was substantially lower in the hippocampal CA1 region of rats in the NS than in the AD group, and the nucleus was morphologically normal in the NS group. Moreover, the degree of tissue swelling and hippocampal abnormalities was significantly lower in the NS group.
As shown in Fig. 2A, the astrocytes in hippocampal CA1 of the NS group were lightly stained with GFAP and loosely distributed, and they had small cell bodies and long processes. The astrocytes in the AD group were darkly stained and exhibited large cell soma and short processes, with the typical characteristics of astrocyte activation. The percentage of activated astrocytes was much higher in the AD than in the NS group (p<0.05) (Fig. 2B). The percentage of astrocyte activation in the hippocampal CA1 region was significantly lower in the HBO+AD than in the AD group (p<0.05), although no significant difference was detected in the percentage of activated astrocytes between the NS and AD+HBO groups (p>0.05). Furthermore, the TNF-α, IL-1β, and IL-10 levels in the hippocampus were higher in the AD than in the NS group (p<0.05), whereas a significantly lower TNF-α elevation was observed in the HBO+AD than in the AD group (p<0.05). No differences were seen in the IL-1β and IL-10 expression level (p>0.05) (Fig. 2C).
As shown in Fig. 3, the AD group showed a predominant loss of dendritic spine in the hippocampal CA1 region (p<0.05), compared with the NS group. In addition, the group given HBO pretreatment showed elevated numbers of AD injury-induced dendritic spines (p<0.05), compared with the AD group.
Hippocampal CA1 sections derived from the three experimental groups underwent TUNEL staining. As shown in Fig. 4A, TUNEL-positive cells exhibited condensed chromosomes, and apoptotic bodies were visualized in some of the apoptotic cells. Most of the apoptotic cells showed small cell soma. The rate of cell apoptosis was remarkably higher in the AD than in the NS group (p<0.05) (Fig. 4B). The rate of cell apoptosis in the hippocampal CA1 region was significantly lower in the HBO+AD than in the AD group (p<0.05). The expression of cleaved caspase 3 was increased remarkably in the AD group, and this increase was efficiently reversed by HBO therapy (Fig. 4C and D). These data suggested that the HBO therapy efficiently decreased cell apoptosis in the hippocampal CA1 region.
The level of phosphorylated p38 MAPK was determined to investigate the molecular mechanism involved in HBO-associated prevention of cognition decline and neuronal apoptosis in this rat model of AD. The level of phosphorylated p38 MAPK was significantly higher in the hippocampus of rats in the AD than NS group (p<0.05) (Fig. 5). However, the phosphorylated p38 MAPK level was significantly lower in the HBO+AD than in the AD group (p<0.05).
Although the neuroprotective effects of HBO therapy have been proposed in several rodent disease models, such as spinal cord injury and hypoxia-ischemia,9 the potential neuroprotective effect of HBO against cognitive decline and hippocampal damage in animal models of AD is not entirely understood. In the present study, we demonstrated for the first time that HBO pretreatment prevents learning and memory decline, hippocampal damage, dendritic spine loss, and astrocyte activation, possibly through inhibition of p38 MAPK activation.
AD is mainly characterized by the deposition of Aβ in the hippocampus and cortex, which lead to neuronal damage and learning and memory decline in affected patients. Evidence indicates that bilateral injection of Aβ1-40 into the CA1 region of the hippocampus induces neurodegeneration, DNA fragmentation, synaptic dysfunction, and impaired cognition in rats.1415 An in vitro study also indicated that Aβ can induce apoptotic and autophagic cell death.1617 In the present study, we found that injection of Aβ1-40 into the CA1 region of the bilateral hippocampus significantly impaired the learning and memory capability of rats 12 days after the initial injection. The cognitive decline was accompanied by an abnormal hippocampal structure and neuronal degeneration. Moreover, increased rates of hippocampal neuron apoptosis, astrocyte activation, and secretion of pro-inflammatory cytokines TNF-α and IL-1β and anti-inflammatory cytokine IL-10 were detected in rats with AD, suggesting the presence of neuroinflammation induced by Aβ injection. These results are consistent with those of previous reports24 in that the accumulation of Aβ may activate astrocytes and trigger the generation of proinflammatory cytokines, which further accelerate the production of Aβ.18 Dendritic spine density has been evidenced as associated with AD-related memory deficits and cognitive decline in animal models of this disease;1920 for example, a mouse model of AD showed reduced dendritic spine density in both the cortex and hippocampus. 21 In accordance with this observation, the AD rat model used in the current study also showed a reduced number of dendritic spines in the hippocampal CA1 region.
Although HBO therapy has shown neuroprotection against several neuronal injuries, the precise effects of HBO treatment on learning and memory improvement remain unknown. In the present study, HBO pretreatment, initiated before the AD pathology was established, greatly attenuated the learning and memory decline in our rat model of AD. HBO pretreatment reduced structural alternations in the hippocampus, decreased the apoptosis of hippocampal neurons, inhibited astrocyte activation, and reduced the generation of TNF-α in the hippocampus of rats with AD. Our findings are consistent with those of previous studies in that HBO pretreatment attenuates neuropathic hyperalgesia in rats by inhibiting astrocyte activation and TNF-α release.22 Notably, the IL-1β and IL-10 levels were not obviously altered following HBO treatment in rats with AD, implying that HBO may have limited effects on IL-1β release. Nevertheless, the underlying mechanism requires further study. HBO therapy was shown to ameliorate the dendritic spine loss in the AD rat model. Since dendritic spine density is correlated with the disease-related memory deficits, we speculate that HBO pretreatment may prevent the characteristic cognitive decline observed in rats with AD, possibly through a mechanism that inhibits dendritic spine loss in the hippocampus.
Activation of p38MAPK has been found to protect against oxidative stress23 and apoptosis;24 however, a growing body of evidence suggests that p38MAPK mediates pro-apoptotic cascades. The activation of p38 MAPK plays an important role in the pathogenesis of AD. Zhao, et al.13 described that the rate of p38 MAPK activation was especially high at the site of neurofibrillary tangle and plaque formation. Moreover, p38 MAPK activation in neurons and astrocytes may be induced by the release of cytokines, such as TNF-α and IL-1β.7 In the present study, we detected a significantly higher rate of p38 MAPK phosphorylation in the hippocampus of rats with AD. HBO pretreatment greatly reduced the rate of p38 MAPK phosphorylation. These findings indicate that HBO pretreatment may reduce neuronal damage and astrocyte activation and subsequently decrease TNF-α production and p38 MAPK phosphorylation, collectively contributing to the improvement of cognitive decline in rats with AD. However, we could not rule out the possibility that the increased p-p38 MAPK level is a consequence, rather than a cause, of AD-like neuronal damage. Blockage of p38 MAPK activation using specific inhibitors may help to understand the underlying mechanism of p38 MAPKmediated neuronal injury in AD rats. Indeed, an intensive global search is underway for the development of small-molecule p38 MAPK inhibitors that may be useful for AD therapy.25 We are planning to further investigate the effects of p38 MAPK inhibitors on Aβ deposition-induced learning and memory dysfunction.
In summary, HBO pretreatment greatly improved the learning and memory ability by inhibiting dendritic spine loss and reduced the neuron apoptosis, astrocyte activation, and TNF-α production in the hippocampus of rats with AD. The neuroprotective effects of HBO might be associated with blockade of p38 MAPK activity. Therefore, HBO therapy, a noninvasive, safe, and inexpensive technique, may be useful in elderly patients with AD. Nevertheless, according to the results of the present study, the neuroprotective effects of HBO remain limited, because, although HBO pretreatment partially prevented cognitive decline, neuronal damage, dendritic spine loss and astrocyte activation, these parameters did not return to baseline levels. Therefore, other approaches might be needed to effectively prevent neuronal damage in patients with AD. Although patients are generally tolerant of HBO therapy, the clinical application of HBO is still limited due to its side effects, including neurotoxicity26 and pulmonary toxicity.27 Therefore, the treatment pressure should be carefully considered when this clinical therapy is applied.
Figures and Tables
Fig. 1
Learning and memory ability in rats of the three experimental groups. The Morris water maze test was conducted before or on postoperative days 7, 14, and 21. The swimming distance (A), escape latency (B), and average velocity (C) were recorded in the NS, AD, and AD+HBO groups (D). The track path was recorded on postoperative day 21. n=8. *p<0.05 compared with NS, †p<0.05 compared with AD. NS, normal saline; AD, Alzheimer’s disease; HBO, hyperbaric oxygen.
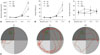
Fig. 2
Neuronal damage and astrocyte activation in the hippocampal CA1 region. (A) Sections derived from the NS, AD, or AD+HBO group on postoperative day 21 were stained with hematoxylin and eosin for histological examination (upper panels). Some of the samples were stained with anti-GFAP antibody. The immunopositive cells are indicated by arrows. Magnification, 400×. (B) The percentage of activated astrocytes was calculated. (C) The TNF-α and IL-1β levels in the hippocampus were examined by ELISA. n=4. *p<0.05 compared with NS, †p<0.05 compared with AD. NS, normal saline; AD, Alzheimer’s disease; HBO, hyperbaric oxygen; TNF-α, tumor necrosis factor-α; IL, interleukin; ELISA, enzyme-linked immunosorbent assay.
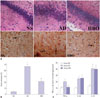
Fig. 3
Dendritic spine density in the hippocampal CA1 region. (A) Sections derived from the NS, AD, or AD+HBO group on postoperative day 21 were stained with Golgi-Cox. Magnification, 1000×. (B) The number of dendritic spines per 10 µm was calculated. Ten neurons from each animal were included in the calculation, and two animals from each group were used for analysis. *p<0.05 compared with NS, †p<0.05 compared with AD. NS, normal saline; AD, Alzheimer’s disease; HBO, hyperbaric oxygen.
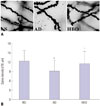
Fig. 4
Cell apoptosis in the hippocampal CA1 region. (A) Sections derived from the NS, AD, or AD+HBO group on postoperative day 21 were analyzed by TUNEL assay. Representative images are presented. Magnification, 400×. (B) The percentage of apoptotic cells was calculated. (C) Protein expression of cleaved caspase 3. β-actin was used as a loading control. (D) The relative expression of cleaved caspase 3 was calculated. n=4. *p<0.05 compared with NS, †p<0.05 compared with AD. NS, normal saline; AD, Alzheimer’s disease; HBO, hyperbaric oxygen; TUNEL, terminal deoxynucleotidyl transferase dUTP nick end labeling.
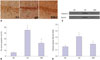
Fig. 5
p38 MAPK phosphorylation in the hippocampus. (A) Proteins were extracted from the NS, AD, or AD+HBO group on postoperative day 21. Western blotting analysis was conducted using anti-phosphorylated p38 MAPK antibody. Representative results are presented. (B) The relative expression of phosphorylated p38 MAPK was calculated. n=4. *p<0.05 compared with NS, †p<0.05 compared with AD. NS, normal saline; AD, Alzheimer’s disease; HBO, hyperbaric oxygen; MAPK, mitogen-activated protein kinase.
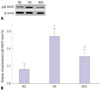
ACKNOWLEDGEMENTS
This work was supported by the Science and Technology Plan Project of Guangdong and Medjaden Academy & Research Foundation for Young Scientists (grant no.2011B061200001 and MJR20150037).We thank Dr. Lingxin Meng for supporting this study.
References
1. James BD, Bennett DA, Boyle PA, Leurgans S, Schneider JA. Dementia from Alzheimer disease and mixed pathologies in the oldest old. JAMA. 2012; 307:1798–1800.


2. Cameron B, Landreth GE. Inflammation, microglia, and Alzheimer’s disease. Neurobiol Dis. 2010; 37:503–509.


3. Zilka N, Ferencik M, Hulin I. Neuroinflammation in Alzheimer’s disease: protector or promoter? Bratisl Lek Listy. 2006; 107:374–383.
4. Malm T, Ort M, Tähtivaara L, Jukarainen N, Goldsteins G, Puoliväli J, et al. beta-Amyloid infusion results in delayed and age-dependent learning deficits without role of inflammation or beta-amyloid deposits. Proc Natl Acad Sci U S A. 2006; 103:8852–8857.


5. Munoz L, Ammit AJ. Targeting p38 MAPK pathway for the treatment of Alzheimer’s disease. Neuropharmacology. 2010; 58:561–568.


6. Sun A, Liu M, Nguyen XV, Bing G. P38 MAP kinase is activated at early stages in Alzheimer’s disease brain. Exp Neurol. 2003; 183:394–405.


7. Corrêa SA, Eales KL. The Role of p38 MAPK and its substrates in neuronal plasticity and neurodegenerative disease. J Signal Transduct. 2012; 2012:649079.


8. Zhao BS, Song XR, Hu PY, Meng LX, Tan YH, She YJ, et al. Hyperbaric oxygen treatment at various stages following chronic constriction injury produces different antinociceptive effects via regulation of P2X4R expression and apoptosis. PLoS One. 2015; 10:e0120122.


9. Liu XH, Yan H, Xu M, Zhao YL, Li LM, Zhou XH, et al. Hyperbaric oxygenation reduces long-term brain injury and ameliorates behavioral function by suppression of apoptosis in a rat model of neonatal hypoxia-ischemia. Neurochem Int. 2013; 62:922–930.


10. Li F, Fang L, Huang S, Yang Z, Nandi J, Thomas S, et al. Hyperbaric oxygenation therapy alleviates chronic constrictive injury-induced neuropathic pain and reduces tumor necrosis factor-alpha production. Anesth Analg. 2011; 113:626–633.


11. Tian X, Zhang L, Wang J, Dai J, Shen S, Yang L, et al. The protective effect of hyperbaric oxygen and Ginkgo biloba extract on Aβ25-35-induced oxidative stress and neuronal apoptosis in rats. Behav Brain Res. 2013; 242:1–8.


12. Tian X, Wang J, Dai J, Yang L, Zhang L, Shen S, et al. Hyperbaric oxygen and Ginkgo Biloba extract inhibit Aβ25-35-induced toxicity and oxidative stress in vivo: a potential role in Alzheimer’s disease. Int J Neurosci. 2012; 122:563–569.


13. Zhao BS, Meng LX, Ding YY, Cao YY. Hyperbaric oxygen treatment produces an antinociceptive response phase and inhibits astrocyte activation and inflammatory response in a rat model of neuropathic pain. J Mol Neurosci. 2014; 53:251–261.


14. Xuan A, Long D, Li J, Ji W, Zhang M, Hong L, et al. Hydrogen sulfide attenuates spatial memory impairment and hippocampal neuroinflammation in β-amyloid rat model of Alzheimer’s disease. J Neuroinflammation. 2012; 9:202.
15. Wu J, Bie B, Yang H, Xu JJ, Brown DL, Naguib M. Suppression of central chemokine fractalkine receptor signaling alleviates amyloid-induced memory deficiency. Neurobiol Aging. 2013; 34:2843–2852.


16. Deng J, Qi XL, Guan ZZ, Yan XM, Huang Y, Wang YL. Pretreatment of SH-SY5Y cells with dicaffeoylquinic acids attenuates the reduced expression of nicotinic receptors, elevated level of oxidative stress and enhanced apoptosis caused by β-amyloid peptide. J Pharm Pharmacol. 2013; 65:1736–1744.


17. Yang Y, Chen S, Zhang J, Li C, Sun Y, Zhang L, et al. Stimulation of autophagy prevents amyloid-β peptide-induced neuritic degeneration in PC12 cells. J Alzheimers Dis. 2014; 40:929–939.


18. Zhao J, O’Connor T, Vassar R. The contribution of activated astrocytes to Aβ production: implications for Alzheimer’s disease pathogenesis. J Neuroinflammation. 2011; 8:150.


19. Rozkalne A, Hyman BT, Spires-Jones TL. Calcineurin inhibition with FK506 ameliorates dendritic spine density deficits in plaque-bearing Alzheimer model mice. Neurobiol Dis. 2011; 41:650–654.


20. Song JM, DiBattista AM, Sung YM, Ahn JM, Turner RS, Yang J, et al. A tetra(ethylene glycol) derivative of benzothiazole aniline ameliorates dendritic spine density and cognitive function in a mouse model of Alzheimer’s disease. Exp Neurol. 2014; 252:105–113.


21. Wang R, Palavicini JP, Wang H, Maiti P, Bianchi E, Xu S, et al. RanBP9 overexpression accelerates loss of dendritic spines in a mouse model of Alzheimer’s disease. Neurobiol Dis. 2014; 69:169–179.


22. Gu N, Niu JY, Liu WT, Sun YY, Liu S, Lv Y, et al. Hyperbaric oxygen therapy attenuates neuropathic hyperalgesia in rats and idiopathic trigeminal neuralgia in patients. Eur J Pain. 2012; 16:1094–1105.


23. Mori H, Oikawa M, Tamagami T, Kumaki H, Nakaune R, Amano J, et al. Oxidized proteins in astrocytes generated in a hyperbaric atmosphere induce neuronal apoptosis. J Alzheimers Dis. 2007; 11:165–174.


24. Park JG, Yuk Y, Rhim H, Yi SY, Yoo YS. Role of p38 MAPK in the regulation of apoptosis signaling induced by TNF-alpha in differentiated PC12 cells. J Biochem Mol Biol. 2002; 35:267–272.


25. Bühler S, Laufer SA. p38 MAPK inhibitors: a patent review (2012 - 2013). Expert Opin Ther Pat. 2014; 24:535–554.


26. Heyboer M 3rd, Jennings S, Grant WD, Ojevwe C, Byrne J, Wojcik SM. Seizure incidence by treatment pressure in patients undergoing hyperbaric oxygen therapy. Undersea Hyperb Med. 2014; 41:379–385.